Wound Healing, Suture Material, and Surgical Instrumentation
Gary H. Lipscomb
DEFINITIONS
Absorbable sutures—Sutures that lose the majority of their tensile strength before 60 days when implanted in body tissues.
Healing by primary intention—Wound healing that occurs if the wound layers are reapproximated following injury.
Healing by secondary intention—Wound healing that occurs if wounds are left open after injury and allowed to close spontaneously from the formation of granulation tissue.
Healing by third intention—Wound healing that occurs when the wound is closed after an initial period of delay of several days also referred to as delayed primary closure.
Knot-pull tensile strength—Breaking strength of a suture when tied around a plastic tube using a surgeon’s flat knot and stressed from either end.
Nonabsorbable sutures—Sutures that maintain the majority of their tensile strength before 60 days when implanted in body tissues.
WOUND HEALING
Ideally, organic tissue lost by destruction or injury would be replaced with tissue identical in form and function. This process is known as regeneration. Although tissue regeneration does occur in lower animals (e.g., salamanders), humans have lost this ability for the most part. With the exception of the epidermis of the skin, mucosa of the intestinal tract, and liver, damaged human tissue heals by the laying down of collagen, a repair process better known as scarring. This process is responsible for emergently sealing the wound and ultimately providing longterm structural support for the injured organ but is unable to reproduce other functions of the replaced tissue. As a result, the healing process also leads to disease if an organ becomes unable to function properly owing to extensive replacement of functioning tissue by nonfunctioning scar. Heart failure following a massive myocardial infarction is an example. In other cases, the healing process itself directly produces disease (e.g., postoperative adhesion formation following surgical procedures or tubal occlusion after pelvic inflammatory disease).
Physiology of Wound Healing
The healing of a wound involves several distinct biologic processes: (a) inflammation, (b) epithelialization, (c) fibroplasia, (d) wound contraction, and (e) scar maturation. Although considered distinct, these processes do not occur in a strict sequence but often occur simultaneously with each other. These repair mechanisms are also nonspecific. They are activated whether a wound is made with a surgical scalpel and sutured closed or by trauma and then allowed to heal without surgical closure. However, the nature of the wound influences the degree to which each individual process is involved, and this in turn can affect the ultimate success of the repair.
Inflammation
The inflammatory phase of healing is the initial response to any injury involving more than an epithelial surface. This phase can be divided into two separate but simultaneously occurring responses: a vascular and a cellular response. Both are initiated by amines, most notably histamine, as well as the kinins and proteolytic enzymes released by the injured tissue. Immediately after injury, a transient vasoconstriction of the local vasculature lasts for 5 to 10 minutes. Vasoconstriction is followed by vasodilatation and an increase in vascular permeability. Edema caused by the escape of plasma through altered vessel walls becomes clinically apparent at this point.
The cellular response is characterized by the migration of leukocytes into the injured area. Although the agents responsible for this active migration of leukocytes remain unclear, chemotactic factors are thought to play a major role. Initially, the polymorphonuclear leukocytes and monocytes in the wound are present in the same concentration as in the systemic circulation. As a result, polymorphonuclear leukocytes predominate for the first 3 days. Because polymorphonuclear leukocytes are relatively short-lived compared with monocytes, the latter stages of the inflammatory phase are characterized by a predominance of monocytes that transform into macrophages. These leukocytes actively phagocytize bacteria, foreign proteins, and necrotic debris. As polymorphonuclear leukocytes die, their intracellular enzymes and debris are released into the wound and become part of the wound exudate. These released enzymes also facilitate the breakdown of material not phagocytized by the leukocytes. This accumulated exudate or pus develops even in the absence of bacteria. Even when this exudate is sterile, the presence of proteolytic enzymes, including collagenase, can interfere with epithelialization and fibroplasia and, thus, interfere with continued wound healing. Poor wound healing, however, is more common in wounds contaminated with bacteria and foreign material. In these cases, the inflammatory response may persist for long periods of time.
Epithelialization
By virtue of their exposed location, the epithelial surfaces of the gastrointestinal, urogenital, and respiratory tract as well as the skin itself are continually subjected to the physical and chemical trauma associated with the activities of daily living. As a result, these surfaces are constantly replacing damaged or destroyed cells through a process known as epithelialization. Epithelialization occurs by migration and subsequent maturation of immature epithelial cells from the deeper basal layers of surrounding areas. If the cellular damage is confined entirely to the epithelium, the healing response is merely an exaggerated form of the basic normal replacement process.
If an injury involves the supporting connective tissue beneath the epithelium, however, the other components of the healing processes, in addition to epithelialization, become involved. If the injury severs blood vessels, the vessels retract, and the process of hemostasis is initiated. If bleeding is not too severe, a blood clot soon forms. This clot subsequently contracts, dehydrates, and becomes a scab. Within 12 hours, basal cells from the surrounding epithelial surfaces begin migrating onto the injured surface. Epithelial cells move beneath the scab, detaching it from the wound and sealing the surface. In incised and sutured wounds, epithelialization generally produces a watertight seal within 24 hours of injury. This new layer of epithelial cells is initially thin and poorly attached to the underlying surface, rendering it susceptible to injury from even minor trauma. Final epithelial healing is accomplished by differentiation and maturation of the migrated cells and by scar formation through fibroplasia.
Fibroplasia
The process by which wounds regain strength is termed fibro-plasia. Fibroplasia results in the production of the collagen necessary to form a fibrous scar and ultimately determines the final strength of the healed wound. This process begins with the differentiation of mesenchymal cells into fibroblasts. Fibroblasts then migrate into the wound, apparently along fibrin strands produced during clot formation. Once at the injury site, fibroblasts proliferate and manufacture the glycoproteins and mucopolysaccharides that make up the ground substance of connective tissue. Ground substance is an amorphous matrix that is believed to induce aggregation of collagen subunits and influences the final orientation of the fibers.
Once the ground substance is produced, fibroblasts begin to synthesize the basic building block of collagen-tropocollagen. Tropocollagen is a stiff elongated macromolecule of three helically intertwined chains of amino acids consisting of two identical α1 chains and one α2 chain. Within the ground substance and at the proper pH, osmolality, and temperature, tropocollagen molecules polymerize into collagen fibrils by forming covalent bonds with their neighbor. These fibrils bond with other fibrils to form collagen bundles. It is not until 4 to 5 days after injury that the wound produces enough collagen to result in a measurable increase in wound tensile strength. Before this time, the wound is held together only by fibrous adhesion. This time frame between wounding and an increase in tensile strength originally was referred to by Howes and Harvey as the “lag period” of wound healing.
Wound Contraction
The manner in which tissue heals is dependent on whether tissue integrity is simply interrupted (as in a surgical incision) or tissue is removed (as in an avulsion injury). In both types of injury, tissue seals itself, begins to reepithelialize, and synthesizes collagen for structural support. However, when large amounts of tissue are missing, the edges of the wound must be brought closer together so that the previously noted tissue responses can repair the defect. This process is known as contraction. Contraction of wound margins begins about 5 days after injury and corresponds with the fibroplasia phase of healing. Because this process can be inhibited by cytochrome poisons, such as potassium cyanide, and by smooth muscle relaxants, older theories attributing wound contraction to passive collagen changes have been discarded. Instead, wound contraction appears to be an active process produced by contractile proteins within the fibroblasts. If the area is too large for contraction to bring the edges together, the wound remains covered with granulation tissue, or if small enough, it is covered with epithelium only. Epithelialization of such a wound prevents weeping, but without the normal underlying supporting stroma, it remains too fragile to provide lasting protection. Pathologic progression of skin contraction ultimately may result in restriction of joint or limb mobility. This deformity is termed contracture and should not be confused with contraction.
Scar Maturation
The bulky scar formed during the fibroplasia phase consists of randomly oriented soluble collagen fibers. This scar has little tensile strength. During scar maturation, the disordered fibers are replaced with fibers arranged in a more orderly fashion, producing a denser and stronger scar. Collagen fibers also continue to form covalent bonds within fibrils as well as between adjacent fibrils and fibers, resulting in a continued increase in wound tensile strength over time. This maturation process may continue for years.
During scar maturation and remolding, the breakdown of old disordered collagen slightly exceeds production of new organized collagen fibers. The resulting new scar is softer and less bulky than is the original scar but also is stronger because of its more organized and extensively cross-linked nature. However, if collagen production exceeds breakdown, then a keloid or hypertrophied scar results.
Surgical Wound Healing
Depending on the manner of wound closure, three types of surgical wound healing are recognized: primary, secondary, and third intention. Figure 13.1 illustrates these types of wound healing.
Primary Intention
Healing occurs by primary intention if the wound layers are reapproximated following injury. This apposition of tissue layers allows healing to occur in a minimum of time, with no separation of wound edges and with minimum scar formation. This is the desired mode of healing for surgical incisions.
Secondary Intention
It has been known for centuries that a wound has a higher resistance to infection when left open rather than closed. This was demonstrated experimentally in dogs by Bilroth, who applied dressings soaked in liquid feces and pus to wounds. Wounds left open remained healthy in appearance, whereas those that were subsequently closed became infected. As a result, contaminated or infected surgical wounds often are left unapproximated and allowed to close spontaneously. This type of wound healing is referred to as healing by secondary intention. This healing process obviously is more complicated and prolonged than that of primary intention. The wound eventually heals by a combination of contraction and the formation of granulation tissue with the wound gradually filling in from the raw surfaces. This type of healing is slow and frequently characterized by formation of excessive scar tissue. Granulation tissue from the healing wound also may protrude above the wound margin during this process. This can prevent final epithelialization of the surface and require further treatment for complete healing.
Third Intention
Wound healing by third intention, also known as delayed primary closure, refers to the technique of wound closure after a period of delay. This method often is used after postoperative wound breakdown or as an alternative to healing by secondary intention of wounds that should not be closed primarily, such as grossly contaminated or infected wounds. The timing of closure is important. After delays of 7 to 8 days, the wound edges become increasingly difficult to approximate because of the increasing collagen content. Edlich and colleagues have suggested that closure on or after the 4th day appears to be ideal. This concept also is supported by Lowery and Curtis, who showed that wounds closed after a delay of between 3 and 6 days have the lowest infection rates. Furthermore, studies by Fogdestam revealed that wounds closed during this time frame also have greater wound strength at 20 days than do wounds closed primarily.
Methods of Wound Closure
Wound dehiscence with evisceration is a serious complication of abdominal surgery. This complication is associated with prolonged morbidity and high mortality. Published mortality figures range from 18% to 35%, with a mean of 20%. Wound dehiscence occurs in 0.5% to 5% of all abdominal surgeries but is less frequent (0.1% to 0.7%) with gynecologic abdominal procedures. Potential reasons for the decreased rate of dehiscence associated with gynecologic surgery include the use of transverse incisions, healthier patients, lower infection rates, and a lower rate of bowel enterotomies.
Traditionally, gynecologic surgeons have been taught that vertical incisions are associated with a greater likelihood of dehiscence than are transverse incisions. Critical review of the earlier data supporting this conclusion reveals that significant confounding variables often were ignored. In general, vertical incisions often were performed emergently on sicker patients or those who had other risk factors for dehiscence, such as cancer. Transverse incisions typically were performed on relatively healthy patients undergoing elective surgery. At least two randomized studies by Greenall and colleagues and Stone and associates have shown no difference in hernia formation or dehiscence between vertical and transverse incisions.
Proper suture selection is critical in preventing wound dehiscence. The wound may break down if the suture has too little initial tensile strength or if the suture is absorbed too quickly before the wound regains enough strength to resist normal stress. The suture also is only as strong as the knots placed in it. If the knot is tied using an improper technique or too few knots are placed, the knot can slip and the suture line fail. The issues involved with proper suture selection and knot security are discussed in detail later in this chapter. Proper surgical technique is one of the most critical factors in preventing wound dehiscence. Several studies have indicated that the most common cause of wound dehiscence is intact sutures pulling through fascia. Animal studies have confirmed that incisions closed with wide loose fascial bites have greater tensile strength than do those closed using smaller fascial bites. Sanders and DiClementi also have shown that tightly tied fascial sutures result in fascial necrosis beneath the sutures. Thus, the old adage that one should “approximate and not strangulate fascia” is well taken.
Although the use of loose, wide fascial bites appears to reduce the likelihood of suture pull-through, the optimum distance sutures should be placed from the fascial edge is unknown. However, data obtained by Campbell and colleagues can provide some guidelines. When the pullout force and pullout energy of sutures placed in cadaver fascia were plotted against bite size, bites of 0.9 cm yielded the maximum pullout force, but maximum pullout energy was obtained with bite sizes of 1.2 to 1.5 cm. Unfortunately, it is unknown whether pullout energy or pullout force is more clinically relevant.
Tera and Aberg, working with human cadavers, have shown that the strength of a sutured midline incision is approximately doubled if sutures are placed lateral enough to include the edge of rectus muscle. Normally a bite of approximately 1.5 to 2 cm is required to include rectus muscle in a midline closure. From these studies, it appears that fascia sutures should be placed at least 1.0 cm from the fascia edge and that bites of 1.5 cm or larger are probably preferable.
The use of large tissue bites may be responsible, in part, for the success of mass closure techniques, such as retention sutures, or the Smead-Jones closure, in which wide sutures are passed through fascia, muscle, and peritoneum. The Smead-Jones mass closure consists of a combination “far” mass closure bite at least 2.5 cm from the fascial edge on each side of the incision with a second “near” bite through the fascial edge. This far-far/near-near technique is considered the gold standard for vertical closure because it has been shown consistently to be more secure than simple layered closure.
Unfortunately, the Smead-Jones technique is time consuming and requires a large number of sutures. One solution to this problem has been the use of a continuous running mass closure. In gynecologic surgery, abdominal incisions traditionally have been closed in layers with interrupted sutures. The belief that interrupted sutures provide greater wound security than does a continuous suture is not well documented, and there are several well-performed studies using both general surgery and gynecologic oncology patient populations showing continuous closure to be comparable to Smead-Jones closure provided that adequate tissue bites are taken. A recent meta-analysis of available randomized trials suggests that a continuous technique also is associated with decrease in subsequent ventral hernia formation. The continuous technique not only is the most rapid of all the mass closure methods but also
is more rapid than are traditional interrupted layer techniques. One theoretical advantage of a continuous suture line is that any stress on the incision is distributed along the entire suture line and not confined to one loop of suture, thereby potentially decreasing the likelihood of suture pullout.
is more rapid than are traditional interrupted layer techniques. One theoretical advantage of a continuous suture line is that any stress on the incision is distributed along the entire suture line and not confined to one loop of suture, thereby potentially decreasing the likelihood of suture pullout.
TABLE 13.1 U.S. Pharmacopeia-Required Knot-Pull Tensile Strength (LB) | ||||||||||||||||||||||||||||||||||||||||||
---|---|---|---|---|---|---|---|---|---|---|---|---|---|---|---|---|---|---|---|---|---|---|---|---|---|---|---|---|---|---|---|---|---|---|---|---|---|---|---|---|---|---|
|
Mass closure techniques classically have used a nonabsorbable permanent suture. The use of nonabsorbable sutures occasionally produces painful palpable knots or results in the formation of suture sinuses. The development of slowly absorbed synthetic sutures has allowed the use of these sutures for running continuous closures, thus avoiding the potential complications of nonabsorbable sutures. Several studies are now available showing excellent results using a continuous absorbable suture line for fascial closure even in high-risk patients. Although the use of continuous polyglycolic acid and polyglactin 910 sutures to close vertical midline incisions has been discouraged by some surgeons, others have obtained good results in patients at low risk for dehiscence. Since these initial studies, other synthetic absorbable sutures (polydioxanone and polyglyconate) that retain their tensile strength much longer than polyglycolic acid and polyglactin 910 have become available. Because of this delayed loss of tension strength compared with other absorbable suture material, polydioxanone and polyglyconate sutures are especially well suited for continuous fascial closure and should be considered the absorbable sutures of choice for mass closure of patients at high risk for dehiscence.
The manner in which the incision is made also may influence the dehiscence rate. Although no good data are available, it has been suggested that the shearing produced when scissors are used to incise the fascia results in increased fascia necrosis and an increased breakdown rate when compared with incisions produced by a scalpel. Likewise, the use of electrosurgery to incise the fascia has been implicated by some data to result in an increase in the dehiscence rate. These factors are magnified if the fascia then is closed improperly. Because such fascia necrosis generally occurs only at the cut edge of the fascia, the use of generous fascia bites during fascial closure probably is more important in preventing fascial dehiscence than is the manner of opening the fascia.
SUTURE MATERIAL
It is unknown when humankind first learned to use strings or animal sinews to ligate bleeding vessels or approximate tissue. Any material used for this purpose is commonly referred to as suture, whereas the act of reapproximating tissue with suture is known as suturing. The first recorded use of suture and suturing dates to the 16th century bc in the Edwin Smith papyrus, the oldest record of a surgical procedure. Over the centuries, many different materials have been used as sutures. These materials include metals (gold, silver, and tantalum wire), plant material (linen and cotton), and animal products (horsehair, tendons, intestinal tissue, and silk).
The United States Pharmacopeia (USP) is the official compendium that defines the various classes of suture as well as sets standards for dimensions and minimum tensile strength for each class of suture marketed in the United States. Most sutures today significantly exceed the minimum tensile strength required by the USP (Table 13.1).
Sutures are divided into size categories based on diameter as defined by the USP. Sutures progressively larger than 0 are numbered in increasing numerical order; that is, 1, 2, etc. Sizes progressively smaller than 0 are indicated by an increasing number of zeros; that is, 0, 00, 000. The smaller the suture, the more zeros. For simplicity, the smaller numbers often are written numerically as 1-0, 2-0, etc., where the first numeral refers to the number of zeros.
The USP characterizes sutures based on their rate of absorption by bodily tissues. Sutures initially are classed as either absorbable or nonabsorbable. Absorbable sutures lose the majority of their tensile strength before 60 days when implanted in body tissues. Absorbable sutures are further subdivided by the USP into natural and synthetic sutures. Figure 13.2 illustrates the percentages of tensile strength remaining for common absorbable sutures at various postoperative time intervals.
Nonabsorbable sutures are defined as sutures that maintain the majority of their tensile strength for more than 60 days in body tissue. These sutures are further subdivided by the USP into three classes: class I is composed of silk or synthetic fibers; class II is composed of cotton or linen fibers or coated natural or synthetic fibers, with the coating forming a casting of significant thickness but not contributing appreciably to strength (these coatings typically are added to improve handling characteristics or resist degradation); and class III sutures are composed of monofilament or multifilament metal wire.
Natural Absorbable Sutures
Plain and Chromic Catgut
One of the oldest suture materials is plain catgut. Catgut consists of highly purified strands of collagen obtained from the submucosa of animals. Despite its name, catgut normally is obtained from sheep or cattle intestines. The name is believed to have originated from the Arabic term kitgut, which referred to the strings of a musical instrument known as a kit. Kitgut was made from sheep intestines and probably served as a readily available source of suture material. Over the years, the term has evolved into catgut.
![]() FIGURE 13.2 Percentage of in vivo tensile strength of absorbable sutures remaining at various postoperative times. |
Because plain catgut is a foreign protein, it elicits a marked inflammatory response in tissue. It is rapidly degraded by proteolytic enzymes released by white blood cells. This suture loses more than 70% of its tensile strength in 7 days and is totally digested by 70 days. Plain gut is used in tissue in which strength is needed for very short periods of time. It is ideal for Pomeroy tubal ligations because it dissolves rapidly and thus allows the severed ends to fall apart. Less rapidly absorbed sutures, particularly permanent suture, are associated with higher failure rates, probably because of fistula formation.
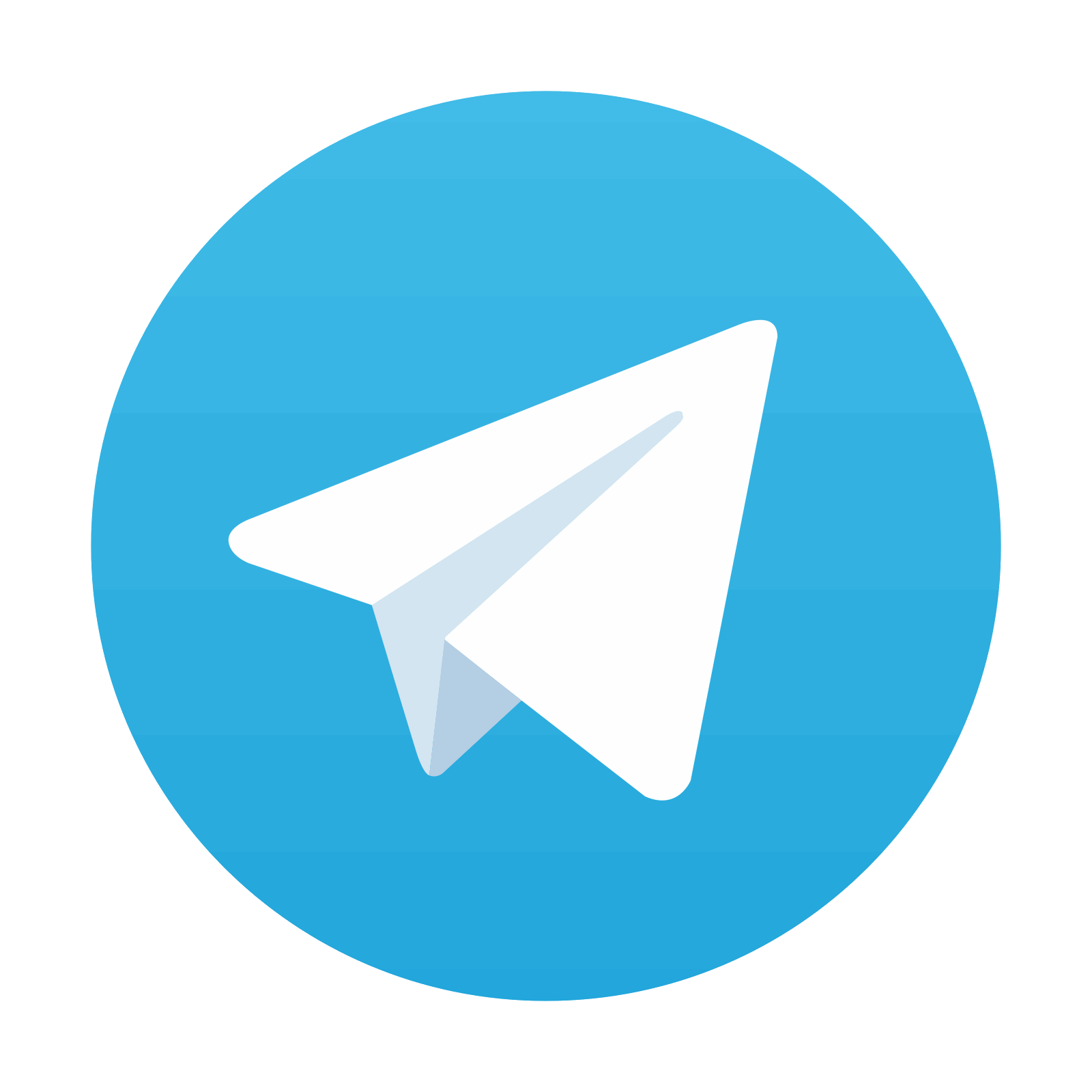
Stay updated, free articles. Join our Telegram channel

Full access? Get Clinical Tree
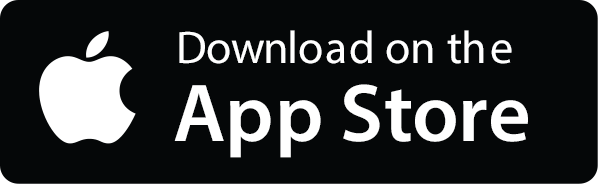
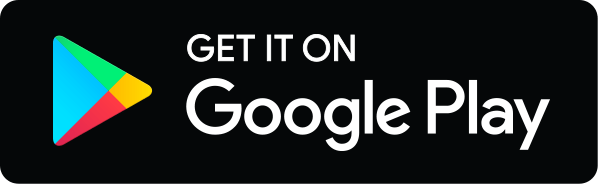
