The Ovary—Embryology and Development
![]() |
The great names of early Western medicine were Hippocrates, Soranus, and Galen. Although Aristotle (384-322 B.C.) referred to castration as a common agricultural practice, it was Soranus who provided the first anatomic description of the ovaries. Soranus of Ephesus (a city founded by Greeks on the coast of what is now Turkey) lived from 98 to 138 A.D. and has often been referred to as the greatest gynecologist of antiquity.1 He studied in Alexandria and practiced in Rome. His great text was lost for centuries and was not published until 1838.
Galen was born in 130 A.D. in Pergamum, a Greek city in eastern Turkey, studied in Alexandria and became a famous practitioner and teacher of medicine also in Rome. He lived 70 years and wrote about 400 treatises, 83 of which are still in existence. Galen preserved in his own writings (in Greek) Aristotle’s descriptions of reproduction. He was a true scholar and was regarded as the ultimate authority on anatomy and physiology until the sixteenth century.2 It was Galen who established bleeding as the appropriate treatment for almost every disorder. Although in retrospect Galen’s conclusions and teachings contained many errors, how many other individuals have been able to satisfy the needs of scholars and physicians for hundreds of years?
After Galen, no further thoughts or advances were recorded for well over 1,000 years as the dark weight of the medieval ages descended on Western civilization. During the medieval
years, it was safe to copy Galen’s works but literally dangerous to contribute anything original. Medieval scholars believed it was impossible to progress in knowledge beyond Galen. The doctrine according to Galen was not challenged until the introduction of printing made Galen’s works available to scholars.
years, it was safe to copy Galen’s works but literally dangerous to contribute anything original. Medieval scholars believed it was impossible to progress in knowledge beyond Galen. The doctrine according to Galen was not challenged until the introduction of printing made Galen’s works available to scholars.
Although Leonardo da Vinci (1452-1519) drew accurately the anatomy of the uterus and the ovaries, the major advances in anatomic knowledge can be traced to the University of Padua, the famed Italian university where a succession of anatomists made important contributions.3 It was Andreas Vesalius (1514-1564), who while still in his 20s, because of his own human dissections, realized that Galen described only animals. Appointed Professor of Surgery and Anatomy at the University of Padua at the age of 23, he published De Humani Corporis Fabrica, his authoritative, illustrated book on human anatomy, in 1543, at the age of 29. Vesalius was harshly attacked by the medical establishment, and one year after the publication of his book, he left Padua to become the court physician in Spain.
Vesalius was the first to describe ovarian follicles and probably the corpus luteum. Fallopius (1523-1562), remembered for his description of the fallopian tubes, was a pupil of Vesalius, and then a successful and popular teacher of anatomy at Padua. Fabricius (Girolamo Fabrici d’Aquapendente, 1533-1619), a pupil of Fallopius, succeeded Fallopius as chair of anatomy at Padua and made major contributions to embryology. Studying an organ in birds and observing that it contained eggs, Fabricius called it the “ovary.” During this period of time, the ovaries came to be recognized as structures, but their function remained a mystery.
William Harvey published the first original English book on reproductive anatomy and physiology in 1651, at the age of 69,35 years after his discovery of the circulation of blood. He obtained his medical education at the University of Padua, where he learned to describe accurately his own observations, a practice he was to continue and that culminated in his writings. Unfortunately, Harvey promoted and maintained the Aristotelian belief that the egg was a product of conception, a result of an interaction between semen and menstrual blood. This view was corrected by Bishop Niels Stensen of Denmark in 1667, and in 1672, at the age of 31, the Dutch physician Regnier de Graaf published his great work on the female reproductive organs, De Mulierum Organis Generationi Inservientibus Tractatus Novus (A New Treatise on the Female Reproductive Organs), that established the ovary as the source of the ovum.
Ovarian follicles had been described by Vesalius and Fallopius, but the impact of his publication earned de Graaf eternal recognition as the ovarian follicle became known as the graafian follicle, even though de Graaf believed that the whole follicle was the egg. de Graaf was the first to accurately describe the corpus luteum, although Marcello Malpighi, whose works were published posthumously in 1697, invented the name “corpus luteum.”
With the discovery of mammalian spermatozoa by van Leeuwenhoek in 1677, it became possible to speculate that fertilization resulted from the combination of a spermatozoon and the graafian follicle. It would be another 150 years before it was appreciated that the oocyte resides within the follicle (described in 1827 by Carl Ernst von Baer), and that there is a relationship between the ovaries and menstruation. The process of fertilization was described by Newport in 1853-1854, and Oscar Hertwig, studying sea urchins, reported in 1876 the penetration of a spermatozoon into an egg and chromosome reduction during meiosis, bringing to a close the era of descriptive anatomy of the ovary and marking the beginning of scientific explorations into physiology and endocrinology.
The Human Ovary
The physiologic responsibilities of the ovary are the periodic release of gametes (eggs, oocytes) and the production of the steroid hormones estradiol and progesterone. Both activities are integrated in the continuous repetitive process of follicle maturation, ovulation, and corpus luteum formation and regression. The ovary, therefore, cannot be viewed as a relatively static endocrine organ whose size and function expand and contract, depending on the vigor of stimulating tropic hormones. Rather, the female gonad is a heterogeneous ever-changing tissue whose cyclicity is measured in weeks, rather than hours.
The ovary consists of three major portions: the outer cortex, the central medulla, and the rete ovarii (the hilum). The hilum is the point of attachment of the ovary to the mesovarium. It contains nerves, blood vessels, and hilus cells, which have the potential to become active in steroidogenesis or to form tumors. These cells are very similar to the testosterone-producing Leydig cells of the testes. The outermost portion of the cortex is called the tunica albuginea, topped on its surface by a single layer of cuboidal epithelium, referred to as the ovarian surface epithelium or the ovarian mesothelium (epithelial ovarian carcinomas account for 90% of human ovarian cancers). The oocytes, enclosed in complexes called follicles, are in the inner part of the cortex, embedded in stromal tissue. The stromal tissue is composed of connective tissue and interstitial cells, which are derived from mesenchymal cells, and have the ability to respond to luteinizing hormone (LH) or human chorionic gonadotropin (hCG) with androgen production. The central medullary area of the ovary is derived largely from mesonephric cells.
The Fetal Ovary
During fetal life, the development of the human ovary can be traced through four stages:4,5 (1) the indifferent gonad stage, (2) the stage of differentiation, (3) the period of oogonal multiplication and oocyte formation, and finally, (4) the stage of follicle formation.
The Indifferent Gonad Stage
At approximately 5 weeks of gestation, the paired gonads are structurally consolidated coelomic prominences overlying the mesonephros, forming the gonadal ridges. At this point, the gonad is morphologically indistinguishable as a primordial testis or ovary. The gonad is composed of primitive germ cells intermingled with coelomic surface epithelial cells and an inner core of medullary mesenchymal tissue. Just below this ridge lies the mesonephric duct. This indifferent stage lasts about 7-10 days. Together, the mesonephros and the genital ridge are called the urogenital ridge, indicating the close association of the urinary and reproductive systems.
The origin of the gonadal somatic cells is still not certain. The earliest recognizable gonad contains, besides the germ cells, somatic cells derived from at least three different tissues: coelomic epithelium, mesenchyme, and mesonephric tissue. In one early model, the gonad was believed to be formed by the invasion of the “germinal epithelium” into the underlying mesenchyme. The germinal epithelium is simply that part of the coelomic epithelium
that gives rise to gonadal tissue. The invading cells were thought to form the primary sex cords that contain the germ cells surrounded by somatic cells (the cells destined to form the tissue that holds the germ cells). In a newer model, well-supported by experimental data, the somatic cells of the gonad are believed to arise from the mesonephros and not the coelomic epithelium.6,7 and 8 Ultrastructural studies have even suggested that both coelomic epithelial and underlying mesonephric cells provide the somatic cells that are destined to become follicular cells.9
that gives rise to gonadal tissue. The invading cells were thought to form the primary sex cords that contain the germ cells surrounded by somatic cells (the cells destined to form the tissue that holds the germ cells). In a newer model, well-supported by experimental data, the somatic cells of the gonad are believed to arise from the mesonephros and not the coelomic epithelium.6,7 and 8 Ultrastructural studies have even suggested that both coelomic epithelial and underlying mesonephric cells provide the somatic cells that are destined to become follicular cells.9
The contribution of mesonephric cells requires migration into the gonad. This movement is regulated by fibroblast growth factor 9 in the male, and it may be repressed by a gene encoding a protein known as Sprouty2.10 Thus the Y chromosome directs sexual development by influencing gene expression via specific regulating and signaling proteins.
The primordial germ cells originate within the primitive ectoderm, but the specific cells of origin cannot be distinguished.11 The germ cells are first identified at the end of the third week after fertilization in the primitive endoderm at the caudal end in the dorsal wall of the adjacent yolk sac, and, soon, they also appear in the splanchnic mesoderm of the hindgut.12,13 The gonadal ridge is the one and only site where the germ cells can survive. By displacement because of growth of the embryo and also by active ameboid movement along the dorsal mesentery of the hindgut toward the genital ridges, the germ cells “migrate” from the yolk sac around the hindgut to their gonadal sites between weeks 4 and 6 of gestation.11,14
The factors that initiate and guide the migration of the germ cells are not known, although chemotactic and adhesive peptides, such as fibronectin and laminin, are involved. In rodents, germ cell proliferation and migration involve stem cell factor (kit ligand) and the expression of its receptor (c-Kit), a transmembrane tyrosine kinase receptor encoded by the c-kit oncogene.15 In gonads obtained from individuals with intersex disorders that have a high risk of testicular tumors, the expression of kit protein was detected at a later gestational age than in normal controls, consistent with both later germ cell migration and a change in the oncogene expression.16 The kit gene is located on chromosome 4, and mutations in this gene have not been discovered in women with premature ovarian failure.17
The germ cells begin their proliferation during their migration.9 The germ cells are the direct precursors of sperm and ova, and by the sixth gestational week, on completion of the indifferent state, these primordial germ cells have multiplied by mitosis to a total of 10,000. By the sixth week of gestation, the indifferent gonads contain the germ cells and supporting cells derived from the coelomic epithelium and the mesenchyme of the gonadal ridge.
Male or female differentiation of the gonad is directed by the sex chromosomes. But the decision to be male or female must be communicated to the cells of the indifferent gonad.18 This communication, too, is genetic in origin, involving signaling proteins and their receptors, programmed by the fundamental impact that depends on whether a Y chromosome is present. A genetic program involving hundreds of genes is initiated in the indifferent gonad at the urogenital ridge that leads to differentiation into a testis or an ovary.19
The Stage of Differentiation
If the indifferent gonad is destined to become a testis, differentiation along this line will take place at 6-9 gestational weeks. The absence of testicular evolution (formation of medullary primary sex cords, primitive tubules, and incorporation of germ cells) gives implicit evidence of the existence of a primitive, albeit momentarily quiescent, ovary. In contrast to the male, female internal and external genitalia differentiation precedes gonadal maturation. These events are related to the genetic constitution and the territorial receptivity of the
mesenchyme. If either factor is deficient or defective, improper development occurs. As has been noted, primitive germ cells are unable to survive in locations other than the gonadal ridge. If partial or imperfect gonadal tissue is formed, the resulting abnormal nonsteroidal and steroidal events have wide ranging morphologic, reproductive, and behavioral effects.
mesenchyme. If either factor is deficient or defective, improper development occurs. As has been noted, primitive germ cells are unable to survive in locations other than the gonadal ridge. If partial or imperfect gonadal tissue is formed, the resulting abnormal nonsteroidal and steroidal events have wide ranging morphologic, reproductive, and behavioral effects.
The Testes
The factor that determines whether the indifferent gonad will become a testis is called, appropriately, the testes-determining factor (TDF), a product of a single gene located on the Y chromosome.20,21 The testicular-determining factor gene is located within a region named SRY, the sex-determining region on the Y chromosome.22 The protein product of the SRY gene contains a DNA-binding domain to activate gene transcription that diverts the development of gonadal somatic cells from the pathway to follicle cells to Sertoli cells.23 Rare cases have been reported of phenotypic, infertile males with a 46,XX karyotype, the male differentiation is due to a translocation of a Y chromosome fragment containing SRY to an autosome or an X chromosome.
Normal testis development requires not only the presence of the SRY gene but its interaction with autosomal genes.24,25 Genes similar to SRY have been named SOX genes (the similarity is with the SRY box region that contains the DNA-binding sequence).23 SRY expression precedes SOX expression; indeed, SRY cooperatively with steroidogenic factor-1, SF1, and Fgf9, a member of the fibroblast growth factor family, upregulates the SOX9 gene.21 Thus, SRY along with partner proteins targets male-specific genes that are essential for testicular development. This process is discussed in detail in Chapter 9. In mice, the developmental consequences of activating and inactivating mutations in Sox9 resemble those of similar mutations in Sry, implying not only that Sox9 is required for testis differentiation, but also that Sry activation of Sox9 may be all that is necessary to activate other genes important to testis development, such as Fgf9 (fibroblast growth factor 9), and to repress genes that induce ovary development, such as Wnt4 (a member of the wingless family of genes), Rspo1 (R-spondin 1), Dax1 (dosage-sensitive sex reversal, adrenal hypoplasia critical region, on chromosome X, gene 1), and Foxl2 (forkhead box L2).26
Both testis and ovary differentiation require dominantly acting genes, with SRY inducing testis development via up-regulation of SOX9, and with other genes, primarily WNT4 and RSPO1, teaming to promote ovary development via repression of SOX9. This concept views the fate of the bipotential gonad as balanced between opposing forces and SRY as the key factor. In XY gonads, SRY induces SOX9 and tips differentiation toward testis development, and in XX gonads lacking SRY, other genes combine to repress SOX9 and promote ovary development.27
The expression of the SRY gene is confined to the genital ridge during fetal life, but the gene is also active in the germ cells of the adult, perhaps playing a role in spermatogenesis.20 The traditional view assigns active gene control and expression for testicular differentiation and a passive, “default” mode of development for the ovary. However, recent evidence has challenged that concept, revealing that ovarian development requires the actions of genes such as WNT4, RSPO1, and DAX1, which team to repress the expression of genes in the testis pathway (e.g. SOX9), and other genes that promote ovarian development.28,29 “Passive” is not an accurate description because even before the gonad differentiates into an ovary, robust gene activity associated with the female genotype occurs in the urogenital ridge.19
When the Y chromosome containing SRY is present, the gonads develop into testes. The male phenotype is dependent on the products (antimüllerian hormone and testosterone) of the fetal testes, whereas the female phenotype is the result of an absence of these fetal gonadal products.30 Antimüllerian hormone (AMH), which inhibits the formation of the müllerian ducts, is secreted at the time of Sertoli cell differentiation, beginning at 7 weeks.
AMH expression is altered only by mutations in the AMH gene, located on chromosome 19p13.3.31 Regression of the müllerian ducts is dependent on the presence of an adequate number of Sertoli cells, and the regulation of AMH receptor.32 Mutations in the AMH receptor gene results in the presence of the uterus, fallopian tubes, and the upper vagina in 46,XY men with normal external virilization.
After involution of the müllerian system, AMH continues to be secreted, but there is no known function. However, evidence in the mouse suggests a role in early germ cell transformation during spermatogenesis.33 In the ovary, very small amounts of AMH mRNA are present early in life, and although there may be no role in female development, its production later in life by the granulosa cells leads to autocrine and paracrine actions in oocyte maturation and follicular development.34 Serum AMH levels in adult women correlate with the number of ovarian follicles present and predict the response to stimulation with ovulation-inducing therapy.35
The testis begins its differentiation in week 6-7 of gestation by the appearance of Sertoli cells that aggregate to form the testicular cords. The primordial germ cells are embedded in the testicular cords that will form the Sertoli cells and spermatogonia. The mature Sertoli cells are the site of production of ABP (androgen-binding protein, important in maintaining the high local androgen environment necessary for spermatogenesis) and inhibin.
The Leydig cells differentiate (beginning week 8) from mesenchymal cells of the interstitial component surrounding the testicular cords. Thus, secretion of AMH precedes steroidogenesis in Leydig cells. Shortly after the appearance of the Leydig cells, secretion of testosterone begins. Androgen secretion increases in conjunction with increasing Leydig cell numbers until a peak is reached at 15-18 weeks. At this time, Leydig cell regression begins, and at birth only a few Leydig cells are present.
The cycle of fetal Leydig cells follows the rise and decline of fetal human chorionic gonadotropin (hCG) levels during pregnancy. This relationship and the presence of hCG receptors in the fetal testes indicate a regulatory role for hCG.4 The pattern of hCG in the fetus parallels that of the mother, peaking at about 10 weeks and declining to a nadir at 20 weeks of gestation, but the concentrations are only 5% of maternal concentrations. Human chorionic gonadotropin stimulation produces Leydig cell hypertrophy, and peak fetal testosterone levels are seen at 15-18 weeks.36 However, normal masculine differentiation occurs in mouse models lacking LH receptors, and molecular evidence indicates that fetal Leydig cells (but not adult cells) respond to adrenocorticotropic hormone (ACTH) as well as hCG37 A primary role for ACTH is supported by the report of a male with an inactivating mutation of the gene for the hCG/LH receptor who developed female genitalia along with a vas deferens and epididymis.38
Testosterone synthesis in human fetal testes begins at the 8th week of gestation, reaches a peak between 15-18 weeks, and then declines. Testicular function in the fetus can be correlated with the fetal hormonal patterns. Although the initial testosterone production and sexual differentiation are in response to the fetal levels of ACTH and hCG, further testosterone production and masculine differentiation are maintained by the fetal pituitary gonadotropins. Decreased testosterone levels in late gestation probably reflect the decrease in gonadotropin levels. The fetal Leydig cells, by an unknown mechanism, avoid downregulation and respond to high levels of hCG and LH by increased steroidogenesis and cell multiplication. This generation of cells is replaced by the adult generation of Leydig cells that becomes functional at puberty and responds to high levels of hCG and LH with down-regulation and decreased steroidogenesis. Leydig cells, therefore, are composed of two distinct populations, one active during fetal life and one active during adult life.
The fetal spermatogonia, derived from the primordial germ cells, are in the testicular cords, surrounded by the Sertoli cells. In contrast to the female, male germ cells do not start meiotic division before puberty.
![]() |
The differentiation of the wolffian system begins with the increase in testicular testosterone production. The classic experiments by Jost indicate that this effect of testosterone is due to local action, explaining why male internal genitalia in true hermaphrodites are only on the side of the testis.30 Not all androgen-sensitive tissues require the prior conversion of testosterone to dihydrotestosterone (DHT). In the process of masculine differentiation, the development of the wolffian duct structures (epididymis, the vas deferens, and the seminal vesicle) is dependent on testosterone as the intracellular mediator, whereas development of the urogenital sinus and urogenital tubercle into the male external genitalia, urethra, and prostate requires the conversion of testosterone to DHT39 In the female, the loss of the wolffian system is due to the lack of locally produced testosterone.
The Stage of Oogonal Multiplication and Oocyte Formation
Just as testicular development requires SRY and SOX9 expression, specific molecular signaling pathways are necessary for ovarian differentiation and oocyte survival. In the developing ovary, the most important genes are WNT4 and RSPO1. Both activate the beta-catenin signaling pathway in somatic cells, which results in the loss of cell-cell adhesion between female germ cells that is required for their entry into meiosis.40 WNT4 and RSPO1 also combine to suppress somatic cell SOX9 expression. Thus, ovarian differentiation is dependent on essential genetic molecular signaling that is active in the absence of SRY. Evidence suggests that RSPO1 also may act directly to suppress male differentiation, even in the absence of SRY.41 The ultimate fate of the gonad depends on which molecular signaling pathway dominates.42
At 6-8 weeks, the first signs of ovarian differentiation are reflected in the rapid mitotic multiplication of germ cells, reaching 6-7 million oogonia by 16-20 weeks.12,43 This represents the maximal oogonal content of the gonad. From this point in time, germ cell content will irretrievably decrease until, some 50 years later, the store of oocytes will be finally exhausted.
By mitosis, the germ cells give rise during week 9 to the oogonia. The oogonia are transformed to oocytes as they enter the first meiotic division and arrest in prophase. This process begins at 11-12 weeks, perhaps in response to a factor or factors produced by the rete ovarii,44 that may act directly on the germ cells, or indirectly via actions on the somatic cells. Studies in mice suggest that retinoic acid derived from the mesonephros may act as a functional meiosis-inducing factor in the female germ cells.45 Progression of meiosis to the diplotene stage is accomplished throughout the rest of pregnancy and completed by birth. Arrest of meiosis at the end of the first stage is probably maintained by inhibiting substances produced by granulosa cells. A single ovum is formed from the two meiotic divisions of the oocyte, one just before ovulation and the second (forming the haploid ovum) at the time of sperm penetration. The excess genetic material is extruded as one polar body at each meiotic division. Gonadotropins and various growth factors (but not sex steroids) can induce resumption of meiosis in vitro, but only in oocytes enclosed by cumulus-granulosa cells. A family of sterols is present in follicular fluid, presumably secreted by the cumulus cells in response to gonadotropins, that activates oocyte meiosis and oocyte maturation.46,47 Follicle-stimulating hormone (FSH) induces the resumption of meiosis, a reaction that requires the presence of the gap junction network that allows communication between the cumulus cells and the oocyte.
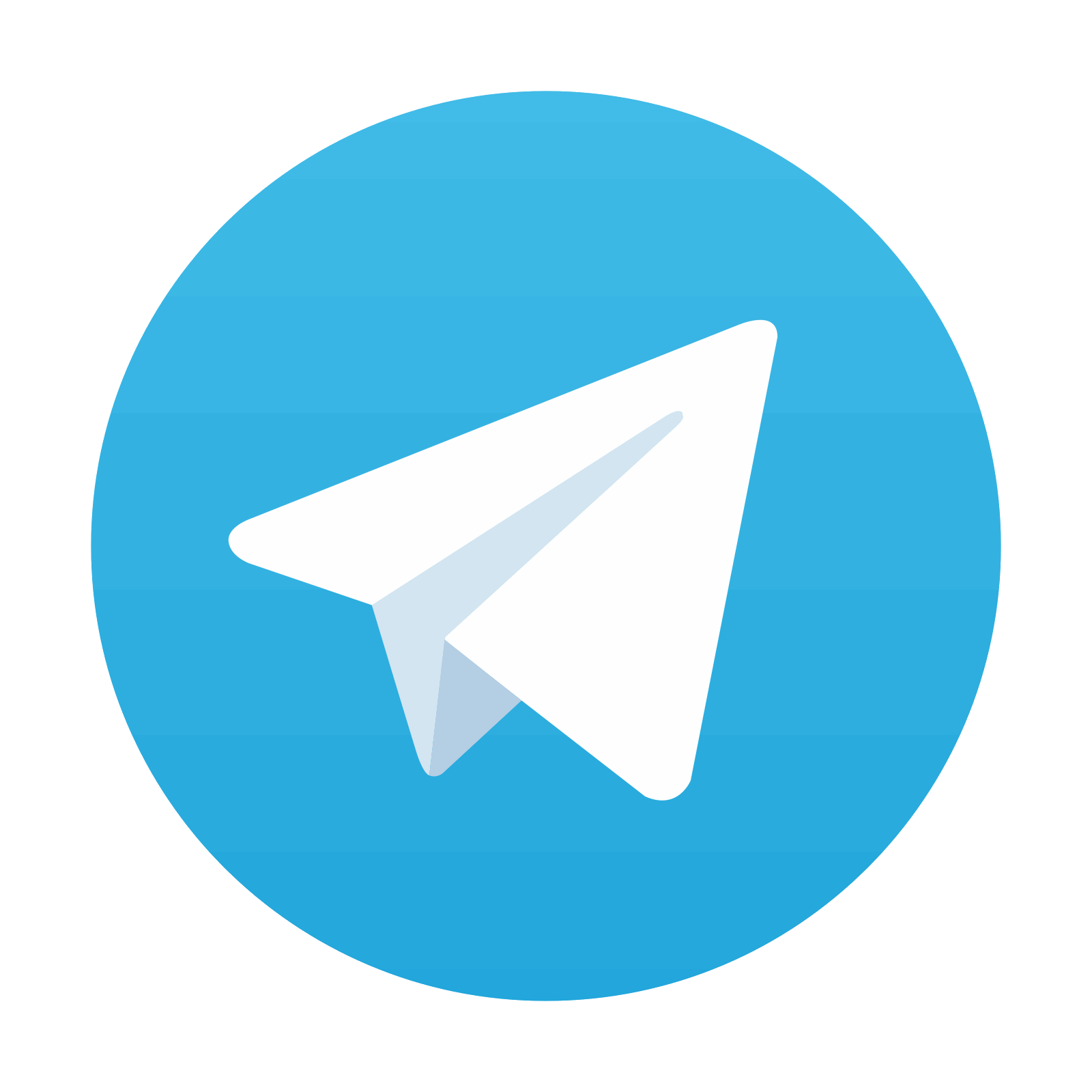
Stay updated, free articles. Join our Telegram channel

Full access? Get Clinical Tree
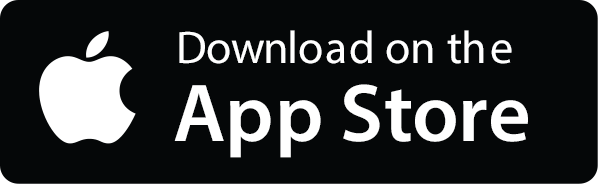
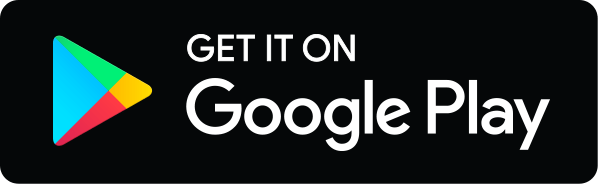