Regulation of the Menstrual Cycle
![]() |
Many superstitious beliefs have surrounded menstruation throughout recorded history. Indeed, attitudes and ideas about this aspect of female physiology have changed slowly. Hopefully, the scientific progress of the last few decades, which has revealed the dynamic relationships between the pituitary and gonadal hormones and the cyclic nature of the normal reproductive process, will yield a better understanding. The hormone changes, correlated with the morphologic and autocrine-paracrine events in the ovary, make the coordination of this system one of the most remarkable events in biology.
The diagnosis and management of abnormal menstrual function must be based on an understanding of the physiologic mechanisms involved in the regulation of the normal cycle. To understand the normal menstrual cycle, it is helpful to divide the cycle into three phases: the follicular phase, ovulation, and the luteal phase. We will examine each of these phases, concentrating on the changes in ovarian and pituitary hormones, what governs the pattern of hormone changes, and the effects of these hormones on the ovary, pituitary, and hypothalamus in regulating the menstrual cycle.
The Follicular Phase
During the follicular phase an orderly sequence of events takes place that ensures the proper number of follicles is ready for ovulation. In the human ovary the end result of
this follicular development is (usually) one surviving mature follicle. This process, which occurs over the space of 10-14 days, features a series of sequential actions of hormones and autocrine-paracrine peptides on the follicle, leading the follicle destined to ovulate through a period of initial growth from a primordial follicle through the stages of the preantral, antral, and preovulatory follicle.
this follicular development is (usually) one surviving mature follicle. This process, which occurs over the space of 10-14 days, features a series of sequential actions of hormones and autocrine-paracrine peptides on the follicle, leading the follicle destined to ovulate through a period of initial growth from a primordial follicle through the stages of the preantral, antral, and preovulatory follicle.
The Primordial Follicle
The primordial germ cells originate in the endoderm of the yolk sac, allantois, and hindgut of the embryo, and by 5-6 weeks of gestation, they have migrated to the genital ridge. A rapid mitotic multiplication of germ cells begins at 6-8 weeks of pregnancy, and by 16-20 weeks, the maximum number of oocytes is reached: a total of 6-7 million in both ovaries.1 The primordial follicle is non-growing and consists of an oocyte, arrested in the diplotene stage of meiotic prophase, surrounded by a single layer of spindle-shaped granulosa cells.
Until their numbers are exhausted, follicles begin to grow and undergo atresia under all physiologic circumstances. Growth and atresia are not interrupted by pregnancy, ovulation, or periods of anovulation. This dynamic process continues at all ages, including infancy and around the menopause. From the maximum number at 16-20 weeks of pregnancy, the number of oocytes will irretrievably decrease. The rate of decrease is proportional to the total number present; thus, the most rapid decrease occurs before birth, resulting in a decline from 6-7 million to 2 million at birth and to 300,000 at puberty. From this large reservoir, about 400 follicles will ovulate during a woman’s reproductive years.
The mechanism for determining which follicles and how many will start growing during any one cycle is unknown. The number of follicles that starts growing each cycle appears to be dependent upon the size of the residual pool of inactive primordial follicles.2,3 Reducing the size of the pool (e.g., unilateral oophorectomy) causes the remaining follicles to redistribute their availability over time. It is possible that the follicle which is singled out to play the leading role in a particular cycle is the beneficiary of a timely match of follicle “readiness” (perhaps prepared by autocrine-paracrine actions in its microenvironment) and appropriate tropic hormone stimulation. The first follicle able to respond to stimulation may achieve an early lead that it never relinquishes. Nevertheless, each cohort of follicles that begins growth is engaged in a serious competition that ends with only one follicle succeeding.
Rescue From Atresia (Apoptosis)
The follicle destined to ovulate is recruited in the first few days of the cycle.4 The early growth of follicles occurs over the time span of several menstrual cycles, but the ovulatory follicle is one of a cohort recruited at the time of the luteal-follicular transition.5,6 The total duration of time to achieve preovulatory status is approximately 85 days. The majority of this time (until a late stage) involves responses that are independent of hormonal regulation.7 Eventually, this cohort of follicles reaches a stage where, unless recruited (rescued) by follicle-stimulating hormone (FSH), the next step is atresia. Thus, follicles are continuously available (2-5 mm in size) for a response to FSH. An increase in FSH is the critical feature in rescuing a cohort of follicles from atresia, the usual fate of most follicles, eventually allowing a dominant follicle to emerge and pursue a path to ovulation. In addition, maintenance of this increase in FSH for a critical duration of time is essential.8 Without the appearance and persistence of an increase in the circulating FSH level, the cohort is doomed to the process of apoptosis, programmed physiologic cell death to eliminate superfluous cells.9 “Apoptosis” is derived from Greek and means falling off, like leaves from a tree.
![]() |
“Recruitment” has been traditionally used to describe the continuing growth of antral follicles in response to FSH. A more useful concept is that the cohort of follicles responding to FSH at the beginning of a cycle is rescued from apoptosis. Remember that the very early development of follicles begins continuously and independently from gonadotropin influence. The fate of almost all of these follicles is apoptosis; only those exposed to an increase in FSH stimulation because of the juxtaposition of their readiness to respond and the increase in FSH during the luteal-follicular transition have the good fortune to compete for selection as a dominant follicle.
The first visible signs of follicular development are an increase in the size of the oocyte and the granulosa cells becoming cuboidal rather than squamous in shape. These changes are better viewed as a process of maturation rather than growth. At this same time, small gap junctions develop between the granulosa cells and the oocyte. Gap junctions are channels that when open permit the exchange of nutrients, ions, and regulatory molecules. Thus, the gap junctions serve as the pathway for nutritional, metabolite, and signal interchange between the granulosa cells and the oocyte. In one direction, inhibition of the final maturation of the oocyte (until the LH surge) is maintained by factors derived from the granulosa cells. In the other direction, the process of follicular growth is influenced by regulatory factors that originate in the oocyte.
The molecular events that regulate primordial follicle formation involve a variety of factors, all locally produced and regulated, including members of the transforming growth factor β (TGF-β) superfamily of proteins and another family of trophic factors called neurotrophins. Activins, inhibins, antimüllerian hormone (AMH) and bone morphogenetic proteins (BMPs) are members of the TGF-β family of proteins. Activins promote and inhibins retard primordial follicle development, and their relative local concentrations in the fetal ovary during the time of follicle assembly may determine the size of the ovarian follicular pool.10 AMH is an important inhibitor of primordial follicle growth, and
BMPs exert the opposite effect.10 Neurotrophins and their receptors are essential for the differentiation and survival of various neuronal populations in the central and peripheral nervous systems, but their presence in the developing ovary suggests they also play a role in ovarian development. Four mammalian neurotrophins have been identified, including nerve growth factor (NGF), brain-derived neurotropic factor (BDNF), neurotrophin-3 (NT-3), and neurotrophin 4/5 (NT-4/5), all of which exert their actions via binding to high-affinity trans-membrane tyrosine kinase receptors encoded by members of the trk proto-oncogene family (NGF to TrkA, BDNF and NT-4/5 to TrkB, and NT-3 to TrkC).11 Observations in NGF- and TrkA-null mice indicate that NGF stimulates the proliferation of ovarian mesenchymal cells during the early stages of follicular assembly and promotes differentiation and synthesis of FSH receptors in granulosa cells. Similar experiments with TrkB-null mice suggest that TrkB signaling is required for oocyte survival after follicular assembly and for preantral follicular development.11 The specific signaling mechanisms that mediate the effects of activins, inhibins, BMPs and neurotrophins remain to be established.
BMPs exert the opposite effect.10 Neurotrophins and their receptors are essential for the differentiation and survival of various neuronal populations in the central and peripheral nervous systems, but their presence in the developing ovary suggests they also play a role in ovarian development. Four mammalian neurotrophins have been identified, including nerve growth factor (NGF), brain-derived neurotropic factor (BDNF), neurotrophin-3 (NT-3), and neurotrophin 4/5 (NT-4/5), all of which exert their actions via binding to high-affinity trans-membrane tyrosine kinase receptors encoded by members of the trk proto-oncogene family (NGF to TrkA, BDNF and NT-4/5 to TrkB, and NT-3 to TrkC).11 Observations in NGF- and TrkA-null mice indicate that NGF stimulates the proliferation of ovarian mesenchymal cells during the early stages of follicular assembly and promotes differentiation and synthesis of FSH receptors in granulosa cells. Similar experiments with TrkB-null mice suggest that TrkB signaling is required for oocyte survival after follicular assembly and for preantral follicular development.11 The specific signaling mechanisms that mediate the effects of activins, inhibins, BMPs and neurotrophins remain to be established.
Other paracrine factors mediate a bi-directional communication between oocytes and their surrounding granulosa cells. Oocytes are linked to their investment of granulosa cells via gap junctions which allow passage of small molecules such as ions (e.g., calcium), metabolites (e.g., pyruvate, nucleic acids, inositol), amino acids (e.g., L-alanine), cholesterol, and intracellular signaling molecules (e.g., cyclic adenosine monophosphate, cAMP) between granulosa cells and oocytes. In mice, targeted deletions of gap junction proteins (known as connexins), disrupt follicular and oocyte development.12 Oocytes are unable to use glucose as an energy source to support meiotic maturation, cannot transport certain amino acids, and lack both the enzymes necessary for cholesterol synthesis and the receptors for its uptake from carrier-borne sources. Consequently, oocytes are dependent on adjacent granulosa cells to metabolize glucose into a usable energy substrate, such as pyruvate, for transport of essential amino acids, such as L-alanine, and for synthesis and transfer of cholesterol.13 To meet their needs, oocytes stimulate glycolysis, amino acid transport, and cholesterol synthesis in granulosa cells via paracrine and juxtacrine signals that promote expression of transcripts involved in these metabolic processes, at least in some species.13 Candidate signaling molecules include closely related members of the TGF-β family, growth differentiation factor 9 (GDF9) and BMP15; both are expressed robustly in oocytes and appear crucial for normal ovarian follicle development in mammalian species.14
Mice that are genetically deficient in growth differentiation factor-9 (GDF-9), a peptide synthesized only in the oocyte after the primordial follicle becomes a preantral follicle, are infertile because follicular development cannot proceed beyond the primordial follicle stage.15,16 Mutations in GDF-9 are rare causes of ovarian failure, but confirmed BMP-15 mutations have not been reported.17,18
Mutations in FOXL2, a gene encoding a transcription factor, cause blepharophimosis/ptosis/ epicanthus inversus syndrome that is a disorder affecting the eyelid and producing premature ovarian failure.19,20 This transcription factor has been demonstrated to be essential for granulosa cell differentiation; indeed, mutations are associated with an absence of the very first sign of follicular development, the change to a cuboidal shape by the granulosa cells.21
The gap junction is composed of channels formed by an arrangement of proteins known as connexins, and more recently as GJAs. The connexin gap junctions are essential for growth and multiplication of the granulosa cells, and for the nutrition and regulation of oocyte development.22 Connexin expression in ovarian follicles is up-regulated by FSH and down-regulated by LH.23 In addition, FSH maintains an open channel in the gap junctions, a pathway that is closed by LH.24 After ovulation, the gap junctions are important again in the corpus luteum, when their function is regulated by locally produced oxytocin.25
With multiplication of the cuboidal granulosa cells (to approximately 15 cells), the primordial follicle becomes a primary follicle. The granulosa layer is separated from the stromal
cells by a basement membrane called the basal lamina. The surrounding stromal cells differentiate into concentric layers designated the theca interna (closest to the basal lamina) and the theca externa (the outer portion). The theca layers appear when granulosa proliferation produces 3-6 layers of granulosa cells.6
cells by a basement membrane called the basal lamina. The surrounding stromal cells differentiate into concentric layers designated the theca interna (closest to the basal lamina) and the theca externa (the outer portion). The theca layers appear when granulosa proliferation produces 3-6 layers of granulosa cells.6
The belief that the initiation of follicular growth is independent of gonadotropin stimulation is supported by the persistence of this initial growth in gonadotropin-deficient mutant mice and in anencephalic fetuses.26,27 In the vast majority of instances this growth is limited and rapidly followed by atresia. In studies of human ovarian follicles, expression of the gene for the FSH receptor could not be detected until after primordial follicles began to grow.28 Furthermore, in a woman with an inactivating mutation in the beta subunit FSH gene, antral follicular activity was present, although successful growth and ovulation were impossible.29 Treatment of FSH-deficient women with exogenous FSH results in follicular growth, ovulation, and pregnancy, demonstrating that oocytes and growth of follicules until the appearance of FSH are normal.29,30
The general pattern of limited growth and quick atresia is interrupted at the beginning of the menstrual cycle when a group of follicles (after approximately 70 days of development) responds to a hormonal change and is propelled to grow. In young women, this cohort numbers 3-11 per ovary.31 The decline in luteal phase steroidogenesis and inhibin-A secretion allows a rise in FSH, beginning a few days before menses.32,33 The timing of this important event was based on data derived from the immunoassay of FSH. Using a sensitive measurement of FSH bioactivity, it has been suggested that increasing bioactivity of FSH begins in the mid- to late luteal phase.34
The Preantral Follicle
Once growth is accelerated, the follicle progresses to the preantral stage as the oocyte enlarges and is surrounded by a membrane, the zona pellucida. The granulosa cells undergo a multilayer proliferation as the theca layer continues to organize from the surrounding stroma. This growth is dependent upon gonadotropins and is correlated with increasing production of estrogen. Molecular studies indicate that all of the granulosa cells in mature follicles are derived from as few as three precursor cells.35
The granulosa cells of the preantral follicle have the ability to synthesize all three classes of steroids; however, significantly more estrogens than either androgens or progestins are produced. An aromatase enzyme system acts to convert androgens to estrogens and is a factor limiting ovarian estrogen production. Aromatization is induced or activated through the action of FSH. The binding of FSH to its receptor and activation of the adenylate cyclase-mediated signal is followed by expression of multiple mRNAs, which encode proteins responsible for cell proliferation, differentiation, and function. Thus, FSH both initiates steroidogenesis (estrogen production) in granulosa cells and stimulates granulosa cell growth.36
Specific receptors for FSH are not detected on granulosa cells until the preantral stage,28 and the preantral follicle requires the presence of FSH in order to aromatize androgens and generate its own estrogenic microenvironment.37 Estrogen production is, therefore, limited by FSH receptor content. The administration of FSH will raise and lower the concentration of its own receptor on granulosa cells (up- and down-regulation) both in vivo and in vitro.38 This action of FSH is modulated by growth factors.39 FSH receptors quickly reach a concentration of approximately 1,500 receptors per granulosa cell.40
FSH operates through the G protein, adenylate cyclase system (described in Chapter 2), which is subject to down-regulation and modulation by many factors, including a calcium-calmodulin
intermediary. Although steroidogenesis in the ovarian follicle is mainly regulated by the gonadotropins, multiple signaling pathways are involved that respond to many factors besides the gonadotropins. Besides the adenylate cyclase enzyme system, these pathways include ion gate channels, tyrosine kinase receptors, and the phospholipase system of second messengers. These pathways are regulated by a multitude of factors, including growth factors, nitric oxide, prostaglandins, and peptides such as gonadotropin-releasing hormone (GnRH), angiotensin II, tissue necrosis factor-a, and vasoactive intestinal peptide. The binding of luteinizing hormone (LH) to its receptor in the ovary is also followed by activation of the adenylate cyclase-cyclic AMP pathway via the G protein mechanism.
intermediary. Although steroidogenesis in the ovarian follicle is mainly regulated by the gonadotropins, multiple signaling pathways are involved that respond to many factors besides the gonadotropins. Besides the adenylate cyclase enzyme system, these pathways include ion gate channels, tyrosine kinase receptors, and the phospholipase system of second messengers. These pathways are regulated by a multitude of factors, including growth factors, nitric oxide, prostaglandins, and peptides such as gonadotropin-releasing hormone (GnRH), angiotensin II, tissue necrosis factor-a, and vasoactive intestinal peptide. The binding of luteinizing hormone (LH) to its receptor in the ovary is also followed by activation of the adenylate cyclase-cyclic AMP pathway via the G protein mechanism.
FSH combines synergistically with estrogen to exert (at least in the nonprimate) a mitogenic action on granulosa cells to stimulate their proliferation. Together, FSH and estrogen promote a rapid accumulation of FSH receptors, reflecting in part the increase in the number of granulosa cells. The early appearance of estrogen within the follicle allows the follicle to respond to relatively low concentrations of FSH, an autocrine function for estrogen within the follicle. As growth proceeds, the granulosa cells differentiate into several subgroups of different cell populations. This appears to be determined by the position of the cells relative to the oocyte.
There is a system of communication that exists within follicles. Not every cell has to contain receptors for the gonadotropins. Cells with receptors can transfer a signal (by gap junctions), which causes protein kinase activation in cells that lack receptors.41 Thus, hormone-initiated action can be transmitted throughout the follicle despite the fact that only a subpopulation of cells binds the hormone. This system of communication promotes a coordinated and synchronous performance throughout the follicle, a system that continues to operate in the corpus luteum.
The role of androgens in early follicular development is complex. Specific androgen receptors are present in the granulosa cells.42 The androgens serve not only as substrate for FSH-induced aromatization but, in low concentrations, can further enhance aromatase activity. When exposed to an androgen-rich environment, preantral granulosa cells favor the conversion of androgens to more potent 5a-reduced androgens rather than to estrogens.43 These androgens cannot be converted to estrogen and, in fact, inhibit aromatase activity.44 They also inhibit FSH induction of LH receptor formation, another essential step in follicular development.45
![]() |
The fate of the preantral follicle is in delicate balance. At low concentrations, androgens enhance their own aromatization and contribute to estrogen production. At higher levels,
the limited capacity of aromatization is overwhelmed, and the follicle becomes androgenic and ultimately atretic.46 Follicles will progress in development only if emerging when FSH is elevated and LH is low. Those follicles arising at the end of the luteal phase or early in the subsequent cycle would be favored by an environment in which aromatization in the granulosa cell can prevail. The success of a follicle depends upon its ability to convert an androgen-dominated micro environment to an estrogen-dominated microenvironment.47,48
the limited capacity of aromatization is overwhelmed, and the follicle becomes androgenic and ultimately atretic.46 Follicles will progress in development only if emerging when FSH is elevated and LH is low. Those follicles arising at the end of the luteal phase or early in the subsequent cycle would be favored by an environment in which aromatization in the granulosa cell can prevail. The success of a follicle depends upon its ability to convert an androgen-dominated micro environment to an estrogen-dominated microenvironment.47,48
Summary of Events in the Preantral Follicle
Initial follicular development occurs independently of hormone influence.
FSH stimulation propels follicles to the preantral stage.
FSH-induced aromatization of androgen in the granulosa results in the production of estrogen.
Together, FSH and estrogen increase the FSH receptor content of the follicle.
The Antral Follicle
Under the synergistic influence of estrogen and FSH there is an increase in the production of follicular fluid that accumulates in the intercellular spaces of the granulosa, eventually coalescing to form a cavity, as the follicle makes its gradual transition to the antral stage. The accumulation of follicular fluid provides a means whereby the oocyte and surrounding granulosa cells can be nurtured in a specific endocrine environment. The granulosa cells surrounding the oocyte are now designated the cumulus oophorus. The differentiation of the cumulus cells is believed to be a response to signals originating in the oocyte.49 The follicular fluid, rich in hormones, growth factors, and cytokines, provides the milieu that is required for the orderly maturation and development of the oocyte and its surrounding cells.
In the presence of FSH, estrogen becomes the dominant substance in the follicular fluid. Conversely, in the absence of FSH, androgens predominate.50,51 LH is not normally present in follicular fluid until the midcycle. If LH is prematurely elevated in plasma and antral fluid, mitotic activity in the granulosa decreases, degenerative changes ensue, and intrafollicular androgen levels rise. Therefore, the dominance of estrogen and FSH is essential for sustained accumulation of granulosa cells and continued follicular growth. Antral follicles with the greatest rates of granulosa proliferation contain the highest estrogen concentrations and the lowest androgen/estrogen ratios, and are the most likely to house a healthy oocyte.52 An androgenic milieu antagonizes estrogen-induced granulosa proliferation and, if sustained, promotes degenerative changes in the oocyte.
The steroids present in follicular fluid can be found in concentrations several orders of magnitude higher than those in plasma and reflect the functional capacity of the surrounding granulosa and theca cells. The synthesis of steroid hormones is functionally compartmentalized within the follicle: the two-cell system.40,46,51,53,54
The Two-Cell, Two-Gonadotropin System
The aromatase activity of the granulosa far exceeds that observed in the theca. In human preantral and antral follicles, LH receptors are present only on the theca cells and FSH
receptors only on the granulosa cells.55,56 Theca interstitial cells, located in the theca interna, have approximately 20,000 LH receptors in their cell membranes. In response to LH, theca tissue is stimulated to produce androgens that can then be converted, through FSH-induced aromatization, to estrogens in the granulosa cells.
receptors only on the granulosa cells.55,56 Theca interstitial cells, located in the theca interna, have approximately 20,000 LH receptors in their cell membranes. In response to LH, theca tissue is stimulated to produce androgens that can then be converted, through FSH-induced aromatization, to estrogens in the granulosa cells.
The interaction between the granulosa and theca compartments, with resulting accelerated estrogen production, is not fully functional until later in antral development. Like preantral granulosa cells, the granulosa of small antral follicles exhibits an in vitro tendency to convert significant amounts of androgen to the more potent 5a-reduced form. In contrast, granulosa cells isolated from large antral follicles readily and preferentially metabolize androgens to estrogens. The conversion from an androgen microenvironment to an estrogen microenvironment (a conversion essential for further growth and development) is dependent upon a growing sensitivity to FSH brought about by the action of FSH and the enhancing influence of estrogen.
As the follicle develops, theca cells begin to express the genes for LH receptors, P450scc, and 3β-hydroxysteroid dehydrogenase.57 The separately regulated (by LH) entry of
cholesterol into mitochondria, utilizing internalization of LDL-cholesterol, is essential for steroidogenesis. Therefore, ovarian steroidogenesis is LH-dependent to a significant degree. Human ovarian granulosa cells, after luteinization and vascularization that occur following ovulation, can use HDL-cholesterol in a system that differs from the LDL-cholesterol pathway. The HDL lipoproteins are not internalized, but rather, the cholesteryl esters are extracted from the lipoproteins at the cell surface and then transferred into the cell.58
cholesterol into mitochondria, utilizing internalization of LDL-cholesterol, is essential for steroidogenesis. Therefore, ovarian steroidogenesis is LH-dependent to a significant degree. Human ovarian granulosa cells, after luteinization and vascularization that occur following ovulation, can use HDL-cholesterol in a system that differs from the LDL-cholesterol pathway. The HDL lipoproteins are not internalized, but rather, the cholesteryl esters are extracted from the lipoproteins at the cell surface and then transferred into the cell.58
![]() |
As the follicle emerges, the theca cells are characterized by their expression of P450c17, the enzyme step which is rate-limiting for the conversion of 21-carbon substrate to androgens.59 Granulosa cells do not express this enzyme and thus are dependent upon androgens from the theca in order to make estrogen. Increasing expression of the aromatization system (P450arom) is a marker of increasing maturity of granulosa cells. The presence of P450c17 only in theca cells and P450arom only in granulosa cells is impressive evidence confirming the two-cell, two-gonadotropin explanation for estrogen production.60
The importance of the two-cell, two-gonadotropin system in the primate is supported by the response of women with a deficiency in gonadotropins to treatment with recombinant (pure) FSH.61,62 and 63 Follicles developed in these women (confirming the essential role of FSH, and the lesser role for LH, in recruitment and initial growth), but estradiol production was limited. Some aromatization occurred, perhaps using androgens originating in the adrenal glands, producing early follicular phase estradiol levels, but the usual robust steroidogenesis was impossible without the presence of LH to provide theca production of androgen substrate. This same response has been observed in experiments that use a GnRH antagonist to produce LH-deficient monkeys, followed by the administration of recombinant, pure human FSH.64,65 These results indicate that only FSH is required for folliculogenesis, and that in the primate, autocrine-paracrine peptides have assumed the important intraovarian role of modulating gonadotropin response. However, the final stages of maturation are optimized by LH, increasing the amount of androgen substrate for estrogen production and promoting the growth of the dominant follicle while simultaneously hastening the regression of smaller follicles.66
Selection of the Dominant Follicle
The successful conversion to an estrogen dominant follicle marks the “selection” of a follicle destined to ovulate, the process whereby, with rare exception, only a single follicle succeeds.67 This selection process is to a significant degree the result of two estrogen actions: (1) a local interaction between estrogen and FSH within the follicle, and (2) the effect of estrogen on pituitary secretion of FSH. While estrogen exerts a positive influence on FSH action within the maturing follicle, its negative feedback relationship with FSH at the hypothalamic-pituitary level serves to withdraw gonadotropin support from the other less developed follicles. The fall in FSH leads to a decline in FSH-dependent aromatase activity, limiting estrogen production in the less mature follicles. Even if a lesser follicle succeeds in achieving an estrogen microenvironment, decreasing FSH support would interrupt granulosa proliferation and function, promote a conversion to an androgenic microenvironment, and thereby induce irreversible atretic change. Indeed, the first event in the process of atresia is a reduction in FSH receptors in the granulosa layer.
The loss of oocytes (and follicles) through atresia is a response to changes in many factors. Certainly gonadotropin stimulation and withdrawal are important, but ovarian steroids and autocrine-paracrine factors are also involved. The consequence of these unfavorable changes, atresia, in the process called apoptosis, programmed cell death, is heralded by alterations in mRNAs required for cell proteins that maintain follicle integrity.68 This type of “natural death” is a physiologic process, in contrast to the pathologic cell death of necrosis.
Once cells have entered the process of apoptosis, their response to FSH is modulated by local growth factors. Tumor necrosis factor (TNF), produced in the granulosa cells, inhibits FSH stimulation of estradiol secretion, except in the dominant follicle.69 An inverse relationship exists between TNF expression and gonadotropin stimulation of granulosa cells. Thus, as the successful follicle increases its response to gonadotropins, its TNF production decreases. Those follicles with a failing response to gonadotropins increase their TNF production, hastening their demise.
Once cells have entered the process of apoptosis, their response to FSH is modulated by local growth factors. Tumor necrosis factor (TNF), produced in the granulosa cells, inhibits FSH stimulation of estradiol secretion, except in the dominant follicle.69 An inverse relationship exists between TNF expression and gonadotropin stimulation of granulosa cells. Thus, as the successful follicle increases its response to gonadotropins, its TNF production decreases. Those follicles with a failing response to gonadotropins increase their TNF production, hastening their demise.
Although the principal function of anti-Müllerian hormone (AMH) is to cause Müllerian duct regression during male sexual differentiation, it is detected in the granulosa cells of early primordial follicles and reaches peak concentrations in small antral follicles.70 Its secretion appears to be regulated by the mature oocyte, and AMH decreases when FSHstimulated follicular growth and estrogen production occur.71,72 Studies with knock-out model mice have indicated that AMH inhibits the recruitment of primordial follicles.73 The paracrine activity of AMH inhibits FSH-stimulated follicle growth, thus suppressing the growth of lesser follicles and allowing the dominant follicle to emerge. Because of these activities, the circulating level of AMH reflects the number of growing follicles, and the blood concentration of AMH can be a measure of ovarian aging and prognosis for fertility.74 Because AMH levels are not affected by gonadotropins or the sex steroids, measurement of AMH is reliable on any day in an individual’s menstrual cycle, even in women on steroid contraception.75
An asymmetry in ovarian estrogen production, an expression of the emerging dominant follicle, can be detected in ovarian venous effluent on day 5 of the cycle, corresponding with the gradual fall of FSH levels observed at the midfollicular phase and preceding the increase in diameter that marks the physical emergence of the dominant follicle.76 This is a crucial time in the cycle. Exogenous estrogen, administered even after selection of the dominant follicle, disrupts preovulatory development and induces atresia by reducing FSH levels below the sustaining level. Because the lesser follicles have entered the process of atresia, loss of the dominant follicle during this period of time requires beginning over, with recruitment of another set of preantral follicles.77
The negative feedback of estrogen on FSH serves to inhibit the development of all but the dominant follicle. The selected follicle remains dependent upon FSH and must complete its preovulatory development in the face of declining plasma levels of FSH. The dominant follicle, therefore, must escape the consequences of FSH suppression induced by its own accelerating estrogen production. The dominant follicle has two significant advantages, a greater content of FSH receptors acquired because of a rate of granulosa proliferation that surpasses that of its cohorts and enhancement of FSH action because of its high intrafollicular estrogen concentration, a consequence of local autocrine-paracrine molecules. Thus, the dominant follicle is more sensitive to FSH, and as long as a critical duration of FSH exposure was initially present, the dominant follicle continues to develop.8 As a result, the stimulus for aromatization, FSH, can be maintained, while at the same time it is being withdrawn from among the less developed follicles. A wave of atresia among the lesser follicles, therefore, is seen to parallel the rise in estrogen.
The accumulation of a greater mass of granulosa cells is accompanied by advanced development of the theca vasculature. By day 9, theca vascularity in the dominant follicle is twice that of other antral follicles.78 This allows a preferential delivery of gonadotropins to the follicle, permitting the dominant follicle to retain FSH responsiveness and sustain continued development and function despite waning gonadotropin levels. The monkey ovary expresses a potent growth factor (vascular endothelial growth factor) that induces angiogenesis, and this expression is observed at the two development points when proliferation of capillaries is important: the emerging dominant follicle and the early corpus luteum.79,80
![]() |
In order to respond to the ovulatory surge and to become a successful corpus luteum, the granulosa cells must acquire LH receptors. FSH induces LH receptor development on the granulosa cells of the large antral follicles. Here again estrogen and local autocrineparacrine peptides (primate), serve as the chief coordinators. With increasing concentrations of estrogen within the follicle, FSH changes its focus of action, from up-regulating its own receptor to generation of the LH receptors.81 The combination of a capacity for continued response despite declining levels of FSH and a high local estrogen environment in the dominant follicle provides optimal conditions for LH receptor development. LH can induce the formation of its own receptor in FSH-primed granulosa cells, but the primary mechanism utilizes FSH stimulation and estrogen enhancement.82,83 The role for estrogen goes beyond synergism and enhancement; it is obligatory.
Evidence from ovarian stimulation for in vitro fertilization indicates that LH plays a critical role in the late stages of follicle development, providing support for the final maturation and function of the dominant follicle.66,84 At least one LH contribution in the late follicular phase is the LH stimulation of androgen production in the theca to provide for the large amounts of estrogen required at this point in the cycle. In addition, the theca androgens may have a direct beneficial effect on essential growth factors within the follicle. LH presence in the follicle prior to ovulation, therefore, is an important contributor to optimal follicular development that ultimately provides a healthy oocyte.85,86
The local action of estrogen within the ovarian follicle was questioned when initial studies failed to detect estrogen receptors in any of the significant ovarian compartments.87 Subsequently, it was discovered that human granulosa cells and primate oocytes contain only mRNA for estrogen receptor-β.88,89,90 and 91 The dynamic expression of estrogen receptor-β is consistent with an important local role for estrogen in ovarian follicle and corpus luteum growth and function.
Although prolactin is always present in follicular fluid, there is no evidence to suggest that prolactin is important during normal ovulatory cycles in the primate.
The Feedback System
Through its own estrogen and peptide production, the dominant follicle assumes control of its own destiny. By altering gonadotropin secretion through feedback mechanisms it optimizes its own environment to the detriment of the lesser follicles.
As reviewed in Chapter 5, gonadotropin-releasing hormone (GnRH) plays an obligatory role in the control of gonadotropin secretion, but the pattern of gonadotropin secretion observed in the menstrual cycle is the result of feedback modulation of steroids and peptides originating in the dominant follicle, acting directly on the hypothalamus and anterior pituitary.4 An increase in GnRH accompanying the LH surge, indicating that estrogen positive feedback operates at both pituitary and hypothalamic sites, has been reported in monkeys, but not in women.92,93 Positron emission tomography studies in women indicated that the positive feedback effects of estrogen on LH occur at the pituitary.94
Estrogen exerts its inhibitory effects in both the hypothalamus and the anterior pituitary, decreasing both GnRH pulsatile secretion and GnRH pituitary response.95 Progesterone also operates in two sites. Its inhibitory action is at the hypothalamic level, and its positive action is directly on the pituitary.96 As determined by positron emission tomography, the primary site of estrogen negative feedback on LH is the hypothalamus.94
The secretion of FSH is very sensitive to the negative inhibitory effects of estrogen even at low levels. At higher levels, estrogen combines with inhibin for a suppression of FSH that is profound and sustained. In contrast, the influence of estrogen on LH release varies with concentration and duration of exposure. At low levels, estrogen imposes a negative feedback relationship with LH. At higher levels, however, estrogen is capable of exerting a positive stimulatory feedback effect on LH release.
The transition from suppression to stimulation of LH release occurs as estradiol rises during the midfollicular phase. There are two critical features in this mechanism: (1) the concentration of estradiol and (2) the length of time during which the estradiol elevation is sustained. In women, the estradiol concentration necessary to achieve a positive feedback is more than 200 pg/mL, and this concentration must be sustained for approximately 50 hours.97 This level of estrogen essentially never occurs until the dominant follicle has reached a diameter of 15 mm.98 The estrogen stimulus must be sustained beyond the initiation of the LH surge until after the surge actually begins. Otherwise, the LH surge is abbreviated or fails to occur at all.
Within the well-established monthly pattern, the gonadotropins are secreted in a pulsatile fashion with a frequency and magnitude that vary with the phase of the cycle. The pulsatile pattern is directly due to a similar pulsatile secretion of GnRH, but amplitude and frequency modulation are the consequence of steroid feedback on both hypothalamus and anterior pituitary.99,100 and 101 Pulsatile secretion is more frequent but smaller in amplitude during the follicular phase compared to the luteal phase, with a slight increase in frequency observed as the follicular phase progresses to ovulation.
The pulsatile pattern of FSH is not easily discerned because of its relatively longer half-life compared to LH, but the experimental data indicate that FSH and LH are secreted simultaneously and that GnRH stimulates the secretion of both gonadotropins. Even as late as only 36-48 hours before menses, gonadotropin secretion is still characterized by infrequent LH pulses and low FSH levels typical of the late luteal phase.100 During the transition from the
previous luteal phase to the next follicular phase, GnRH and the gonadotropins are released from the inhibitory effects of estradiol, progesterone, and inhibin. A progressive and fairly rapid increase in GnRH pulse secretion is associated with a preferential secretion of FSH compared to LH. The frequency of GnRH and LH pulses increases 4.5-fold during this period of time, accompanied by a 3.5-fold increase in the circulating levels of FSH, and a lesser 2-fold increase in LH levels.102
previous luteal phase to the next follicular phase, GnRH and the gonadotropins are released from the inhibitory effects of estradiol, progesterone, and inhibin. A progressive and fairly rapid increase in GnRH pulse secretion is associated with a preferential secretion of FSH compared to LH. The frequency of GnRH and LH pulses increases 4.5-fold during this period of time, accompanied by a 3.5-fold increase in the circulating levels of FSH, and a lesser 2-fold increase in LH levels.102
The GnRH pulse frequency changes in the luteal phase correlate with duration of exposure to progesterone, while pulse amplitude changes appear to be influenced by changes in progesterone levels.99 Both estradiol and progesterone are required to achieve the low, suppressed secretory pattern of GnRH during the luteal phase.103 The evidence suggests that steroids influence the hypothalamic release of GnRH for frequency changes and the pituitary for action on amplitude of the gonadotropin pulses. The inhibitory action of luteal phase steroids appears to be mediated by an increase in hypothalamic endogenous opioid peptides. Both estrogen and progesterone can increase endogenous opiates, and administration of clomiphene (an estrogen antagonist) during the luteal phase increases the LH pulse frequency with no effect on amplitude.104 Thus, estrogen appears to enhance the stimulatory action of progesterone in the luteal phase on endogenous opioid peptides, creating relatively high levels of endogenous opiates during the luteal phase.
Plasma endorphin begins to rise in the 2 days before the LH peak, coinciding with the midcycle gonadotropin surge.105 The maximal level is reached just after the LH peak, coinciding with ovulation. Levels then gradually decline until the nadir is reached during menses and the early follicular phase. Monkeys have their highest beta-endorphin levels in the hypophyseal portal blood at midcycle.106 Normal cyclicity requires sequential periods of high (midcycle and luteal phase) and low (during menses) hypothalamic opioid activity.
There is another important action of estrogen. A disparity exists between the patterns of FSH and LH secretion as determined by immunoassay and bioassay, indicating that more biologically active gonadotropins are secreted at midcycle than at other times in the cycle.107,108 This quality, bioactivity vs immunoreactivity, is determined by the molecular structure of the gonadotropin molecule, a concept referred to in Chapter 2 as heterogeneity of the tropic hormones. There is a well-established relationship between the activity and half-life of glycoprotein hormones and their sialic acid content. The feedback effects of estrogen include modulation of sialylation and the size and activity of the gonadotropins subsequently released, as well as an augmentation of GnRH-stimulated secretory release of biologically active gonadotropin. It certainly makes sense to intensify the gonadotropin effect at midcycle. The positive feedback action of estrogen, therefore, increase both the quantity and the quality (the bioactivity) of FSH and LH. In addition to the change at midcycle that favors gonadotropin activity at the ovarian follicle, FSH isoforms with greater biologic activity also increase during the late luteal phase, a change that is appropriately geared toward propelling new ovarian follicle growth for the next cycle.109
There is a diurnal rhythm in FSH and LH secretion.110 In contrast to the nocturnal rise seen with ACTH, thyroid-stimulating hormone (TSH), growth hormone, and prolactin, FSH and LH exhibit nocturnal decline, probably mediated by endogenous opiates. This diurnal rhythm for LH is present only in the early follicular phase, while FSH maintains a circadian rhythm throughout the menstrual cycle (and thus it is not influenced by steroid hormone feedback) and even in the postmenopausal period of life.
Inhibin, Activin, Follistatin
This family of peptides is synthesized by granulosa cells in response to FSH and secreted into the follicular fluid and ovarian venous effluent.111,112,113 and 114 The expression of these peptides
is not limited to the ovary; they are present in many tissues throughout the body serving as autocrine-paracrine regulators. Inhibin is an important inhibitor of FSH secretion. Activin stimulates FSH release in the pituitary and augments FSH action in the ovary. Follistatin suppresses FSH activity, by binding activin.
is not limited to the ovary; they are present in many tissues throughout the body serving as autocrine-paracrine regulators. Inhibin is an important inhibitor of FSH secretion. Activin stimulates FSH release in the pituitary and augments FSH action in the ovary. Follistatin suppresses FSH activity, by binding activin.
Inhibin consists of two dissimilar peptides (known as alpha- and beta-subunits) linked by disulfide bonds. Two forms of inhibin (inhibin-A and inhibin-B) have been purified, each containing an identical alpha-subunit and distinct but related beta-subunits. Thus, there are three subunits for inhibins: alpha, beta-A, and beta-B. Each subunit is a product of different messenger RNA, each derived from its own precursor molecule.
The 2 Forms of Inhibin: | ||||
---|---|---|---|---|
|
FSH stimulates the secretion of inhibin from granulosa cells and, in turn, is suppressed by inhibin—a reciprocal relationship.115,116 Refinements in assay techniques have revealed that inhibin-B is the form of inhibin predominantly secreted by granulosa cells in the follicular phase of the cycle.117,118 The secretion of inhibin is further regulated by local autocrineparacrine control. GnRH and epidermal growth factor diminish FSH stimulation of inhibin secretion, whereas insulin-like growth factor-I enhances inhibin production. The inhibitory effects of GnRH and epidermal growth factor are consistent with their known ability to decrease FSH-stimulated estrogen production and LH receptor formation. The two forms of GnRH (GnRH-I and GnRH-II) along with their receptor are expressed in granulosa cells.119,120
![]() |
The secretion of inhibin-B into the circulation further amplifies the withdrawal of FSH from other follicles, another mechanism by which an emerging follicle secures dominance. Inhibin-B rises slowly but steadily, in a pulsatile fashion (60-70 minute periodicity) reaching peak levels in the early and midfollicular phases, and then decreasing in the late follicular phase before ovulation to reach a nadir in the midluteal phase.33,117,121,122 An inhibin-B peak the day after ovulation is probably the result of release from the ruptured follicle. This relationship of inhibin-B and FSH is supported by the demonstration that inhibin-B levels are lower and FSH levels are higher in the follicular phase in women 45-49 years old compared to younger women.121,123 An ovarian fibrothecoma secreting inhibin-B predictably was associated with secondary amenorrhea and infertility due to suppression of FSH secretion.124
With the appearance of LH receptors on the granulosa cells of the dominant follicle and the subsequent development of the follicle into a corpus luteum, inhibin expression comes under the control of LH, and expression changes from inhibin-B to inhibin-A.125 The circulating levels of inhibin-A rise in the late follicular phase to reach a peak level at the midluteal phase.33,126 Inhibin-A, therefore, contributes to the suppression of FSH to nadir levels during the luteal phase, and to the changes at the luteal-follicular transition.
Inhibin has multiple, diverse inhibitory effects on gonadotropin secretion. Inhibin can block the synthesis and secretion of FSH, prevent the up-regulation of GnRH receptors by GnRH, reduce the number of GnRH receptors present, and, at high concentrations, promote the intracellular degradation of gonadotropins.
Activin, derived from granulosa cells, but present as well in the pituitary gonadotropes, contains two subunits that are identical to the beta subunits of inhibins A and B. In addition, activins have been identified with variants of the beta subunits, designated as beta-C, beta-D, and beta-E.127 The activin beta-C and beta-E genes have been demonstrated to be nonessential in mouse knockout models.128 Activin augments the secretion of FSH and inhibits prolactin, ACTH, and growth hormone responses.129,130,131 and 132 Activin increases pituitary response to GnRH by enhancing GnRH receptor formation.133,134 The effects of activin are blocked by inhibin and follistatin.135 The structure of the activin genes is homologous to that of transforming growth factor-β, indicating that these products all come from the same gene family.136 Another important member of this family is the antimüllerian hormone, as well as a protein active during insect embryogenesis, and a protein active in frog embryos.
The 3 Forms of Activin: | ||||||
---|---|---|---|---|---|---|
|
Activin is present in many cell types, regulating growth and differentiation. In the ovarian follicle, activin increases FSH binding in granulosa cells (by regulating receptor numbers) and augments FSH stimulation of aromatization and inhibin production.113 Considerable evidence derived from human cells exists to indicate that inhibin and activin act directly on theca cells to regulate androgen synthesis.137,138 and 139 Inhibin enhances the stimulatory action of LH and or IGF-I, while activin suppresses this action. Inhibin in increasing doses can overcome the inhibitory action of activin. Prior to ovulation, activin suppresses granulosa progesterone production, perhaps preventing premature luteinization. There is a repertoire of cell transmembrane kinase receptors for activin, with differing binding affinities and domain structures.140 This receptor heterogeneity allows the many different responses elicited by a single peptide. Both activin A and inhibin-A have been demonstrated to be very potent in stimulating in vitro maturation of oocytes that subsequently yield a high rate of fertilization.141
In the male, activin inhibits and inhibin facilitates LH stimulation of androgen biosynthesis in Leydig cells. In addition, activin stimulates and inhibin decreases spermatogonial
proliferation; inhibin is produced in the Sertoli cell, the locus that has the principal role in modulating spermatogenesis. Thus, activin and inhibin play similar autocrine-paracrine roles in both the male and female gonads.
proliferation; inhibin is produced in the Sertoli cell, the locus that has the principal role in modulating spermatogenesis. Thus, activin and inhibin play similar autocrine-paracrine roles in both the male and female gonads.
The anterior pituitary expresses the inhibin-activin subunits, and locally produced activin B augments FSH secretion. Activin A has been demonstrated to directly stimulate the synthesis of GnRH receptors in pituitary cells.142 Follistatin is a glycopeptide secreted by a variety of pituitary cells, including the gonadotropes.143 This peptide has also been called FSH-suppressing protein because of its main action: inhibition of FSH synthesis and secretion and the FSH response to GnRH by binding to activin and in that fashion decreasing the activity of activin.144,145 Activin stimulates follistatin production, and inhibin prevents this response. Follistatin is also expressed by granulosa cells in response to FSH, and, therefore, follistatin, like inhibin and activin, functions locally in the follicle and in the pituitary.146 Circulating levels of activin increase in the late luteal phase to peak at menses; however, activin A is highly bound in the circulation, and it is not certain it has an endocrine role.147 Nevertheless, the timing is right for activin to contribute to the rise in FSH during the luteal-follicular transition.
In summary, the pituitary secretion of FSH can be significantly regulated by the balance of activin and inhibin, with follistatin playing a role by inhibiting activin and enhancing inhibin activity. Within the ovarian follicle, activin and inhibin influence growth and development by modulating theca and granulosa responses to the gonadotropins.
The inhibin-activin family of peptides (also including antimüllerian hormone and transforming growth factor-β) inhibits cell growth and can be considered as a class of tumorsuppressor proteins. Mice have been generated that are deficient in the inhibin-alpha-subunit gene.114 The mice that are homozygous and lack inhibin are susceptible to the development of gonadal stromal tumors that appear after normal sexual differentiation and development. Thus, the alpha-inhibin gene is a specific tumor-suppressor gene for the gonads. A contributing factor to this tumor development could be the high FSH levels associated with the deficiency in inhibin.
Growth Factors
Growth factors are polypeptides that modulate cell proliferation and differentiation, operating through binding to specific cell membrane receptors. They are not classic endocrine substances; they act locally and function in paracrine and autocrine modes. There are multiple growth factors, and most cells contain multiple receptors for the various growth factors.
Insulin-Like Growth Factors
The insulin-like growth factors (also called somatomedins) are peptides that have structural and functional similarity to insulin and mediate growth hormone action.148 Insulin-like growth factor-I (IGF-I) and insulin-like growth factor-II (IGF-II) are single chain polypeptides containing three disulfide bonds. IGF-I is encoded on the long arm of chromosome 12 and IGF-II on the short arm of chromosome 11 (which also contains the insulin gene). The genes are subject to a variety of promoters, and thus differential regulation can govern ultimate actions.
IGF-I mediates the growth-promoting actions of growth hormone. The majority of circulating IGF-I is derived from growth hormone-dependent synthesis in the liver. However, IGF-I is synthesized in many tissues where production can be regulated in conjunction with growth hormone or independently by other factors.
IGF-II has little growth hormone dependence. It is believed to be important in fetal growth and development. Both IGFs induce the expression of cellular genes responsible for cellular proliferation and differentiation.
Insulin-like Growth Factor Binding Proteins. There are six known nonglycosylated peptides that function as IGF binding proteins, IGFBP-1 to IGFBP-6.149 These binding proteins serve to carry the IGFs in serum, prolong half-lives, and regulate tissue effects of the IGFs. The regulating action appears to be due to binding and sequestering of the IGFs, preventing their access to the cell membrane surface receptors, and, thus, not permitting the synergistic actions that result when gonadotropins and growth factors are combined. The IGFBPs may also exert direct actions on cellular functions, independently of growth factor functions. IGFBP-1 is the principal BP in amniotic fluid; IGFBP-3 is the main BP in serum and its synthesis, primarily in the liver, is dependent on growth hormone. Circulating levels of IGFBP-3 reflect the total IGF concentration (IGF-I plus IGF-II) and carry at least 90% of the circulating IGFs. These BPs do not bind insulin. The BPs change with age (decreasing levels of IGFBP-3) and during pregnancy (decreasing IGFBP-3 due to a circulating protease unique to pregnancy).
The IGF Receptors. The Type I receptor preferentially binds IGF-1 and can be called the IGF-I receptor. The Type II receptor in a similar fashion can be called the IGF-II receptor. IGF-I also binds to the insulin receptor but with low affinity. Insulin binds to the IGF-I receptor with moderate affinity. The IGF-I receptor and the insulin receptor are similar in
structure: tetramers composed of two α-subunits and two β-subunits linked by disulfide bonds. The intracellular component of the β-subunit is a tyrosine kinase that is activated by autophosphorylation. The IGF-II receptor does not bind insulin. It is a single chain glycoprotein, with 90% of its structure extending extracellularly. This receptor functions as a receptor coupled to a G protein. The physiologic effects of IGF-I are mediated by its own receptor, but IGF-II can exert its actions via both receptors. Indeed, the IGF-I receptor binds IGF-I and IGF-II with equal affinity. In human cells, the IGF-I receptor and IGF-II receptor are present in theca and granulosa cells and in luteinized granulosa cells. Ovarian stromal tissue contains IGF-I receptors.
structure: tetramers composed of two α-subunits and two β-subunits linked by disulfide bonds. The intracellular component of the β-subunit is a tyrosine kinase that is activated by autophosphorylation. The IGF-II receptor does not bind insulin. It is a single chain glycoprotein, with 90% of its structure extending extracellularly. This receptor functions as a receptor coupled to a G protein. The physiologic effects of IGF-I are mediated by its own receptor, but IGF-II can exert its actions via both receptors. Indeed, the IGF-I receptor binds IGF-I and IGF-II with equal affinity. In human cells, the IGF-I receptor and IGF-II receptor are present in theca and granulosa cells and in luteinized granulosa cells. Ovarian stromal tissue contains IGF-I receptors.
![]() |
The Ovarian Actions of IGFs. IGF-I has been demonstrated to stimulate the following events in ovarian theca and granulosa cells: DNA synthesis, steroidogenesis, aromatase activity, LH receptor synthesis, and inhibin secretion. IGF-II stimulates granulosa mitosis. In human ovarian cells, IGF-I, in synergy with FSH, stimulates protein synthesis and steroidogenesis. After LH receptors appear, IGF-I enhances LH-induced progesterone synthesis and stimulates proliferation of granulosa-luteal cells. IGF-I, in synergy with FSH, is very active in stimulating aromatase activity in preovulatory follicles. Thus, IGF-I can be involved in both estradiol and progesterone synthesis.
![]() |
In animal experiments, the synthesis of IGF-I by granulosa cells is dependent upon FSH but enhanced by estradiol. Growth hormone also acts synergistically with FSH and estradiol to increase IGF synthesis. The story becomes confused when various growth factors and regulators are studied, because of their various stimulating and inhibiting effects. In the rat, the granulosa cell is the major site for IGF-I gene expression, which is active only prior to ovulation. It is not detected in atretic follicles or in corpora lutea. Again in the rat, IGF-II gene expression appears to be limited to the theca and interstitial cells. However, the site of IGF expression is different in primates.
In studies with human ovarian tissue, IGF-II is highly expressed in both theca cells and granulosa cells; however, the level is highest in the granulosa and increases with growth of the follicle.150,151 IGF-II is also synthesized by luteinized granulosa and appears to function locally in an autocrine fashion.152 These findings indicate that IGF-II is the primary IGF in the human ovary. Nevertheless, IGF-I is still a significant product of human theca cells.153
Human theca cells express mRNA transcripts that encode receptors for both IGF-I and insulin.154 Because insulin and IGF-II can both activate the receptor for IGF-I, this pathway provides a method for the exertion of a paracrine influence on granulosa cells and autocrine activity in the theca (augmenting LH stimulation of androgen production). In vitro studies confirm that IGF-II is capable of stimulating steroidogenesis and proliferation in human theca and granulosa cells.155,156 and 157 These actions are augmented by growth hormone, which increases IGF production and, thus, indirectly enhances gonadotropin stimulation of ovarian follicles.158
This primate scenario is supported by finding higher levels of IGF-II, but not IGF-I, in the follicular fluid of developing follicles, with the highest levels present in dominant follicles.159 The IGF levels in follicular fluid correlate with estradiol levels and undergo a further short increase after the LH surge. There are no menstrual cycle changes in the circulating levels of IGF-I, IGF-II, IGFBP-1, or IGFBP-3; high levels in the dominant follicle are not associated with an increase in circulating levels.160
In studies with human tissue, IGFBP-1 inhibits IGF-1 mediated steroidogenesis and proliferation of luteinized granulosa cells. The synthesis of IGFBPs by human granulosa is inhibited by FSH, IGF-I, and IGF-II.161,162 These findings fit with the overall idea that the BPs counteract the synergistic results of gonadotropins and growth factors. In general, IGFBP-1 expression is found in granulosa cells of growing follicles; IGFBP-3 in theca cells and the granulosa of the dominant follicle; IGFBP-2, -4, and -5 in theca and granulosa of antral and atretic follicles; and IGFBP-6 has not been found in the ovary.150 The predominant binding protein in preovulatory follicles is IGFBP-2 in the granulosa and IGFBP-3
in the theca, that increase progressively in the follicle that emerges as the dominant follicle, and then decrease in the late follicular phase.151,163,164 This suggests that -1, -2, and -3 play a role in growing follicles, -2, -4, and -5 in atretic and failing follicles. IGFBP expression in polycystic ovaries is similar to that seen in atretic follicles. The decrease in IGFBP-3 that occurs in dominant follicles should allow an increase in IGF levels and activity. The increase in IGFBP-2 in failing follicles probably correlates with sequestering of IGF, depriving the follicle of an important force in gonadotropin augmentation.
in the theca, that increase progressively in the follicle that emerges as the dominant follicle, and then decrease in the late follicular phase.151,163,164 This suggests that -1, -2, and -3 play a role in growing follicles, -2, -4, and -5 in atretic and failing follicles. IGFBP expression in polycystic ovaries is similar to that seen in atretic follicles. The decrease in IGFBP-3 that occurs in dominant follicles should allow an increase in IGF levels and activity. The increase in IGFBP-2 in failing follicles probably correlates with sequestering of IGF, depriving the follicle of an important force in gonadotropin augmentation.
![]() |
Circulating levels of IGFBP-1 decrease in response to insulin, and thus circulating levels are decreased in women with anovulation and polycystic ovaries who have elevated levels of insulin.165 These patients also have increased circulating levels of IGF-I, probably a consequence of LH-stimulated synthesis and secretion in theca cells. The level of IGFBP-1 in follicular fluid from polycystic ovaries is decreased; thus this BP is not playing a role inhibiting the action of IGF-I in polycystic ovaries. The levels of IGFBPs -2 and -4 in the follicular fluid from follicles in anovulatory patients are increased (as in atretic follicles).150, 166 Even though these changes may play a role in anovulatory pathophysiology, they are consistent with failure in development and thus may not be etiologic factors.
IGF activity may also be modulated by the proteases that regulate the activity of the IGF binding proteins.167 Estrogen-dominant follicular fluid contains very low levels of IGFBP-4, in contrast to the high levels present in androgen-dominant follicular fluid. The low level of IGFBP-4 in estrogen-dominant follicular fluid is associated with the presence of an IGFBP-4 specific protease. This protease would decrease IGFBP activity and enhance IGF activity, another mechanism for ensuring the success of the dominant follicle.
The insulin-like growth factor story is at once complex, fascinating, and compelling. However, its contribution may be facilitatory and not essential. Laron-type dwarfism is characterized by a deficiency in IGF-I due to an abnormality in the growth hormone receptor. Despite low levels of IGF-I and high levels of IGFBP, a woman with Laron-type dwarfism responded to exogenous gonadotropin stimulation with the production of multiple, mature follicles with good estrogen production and fertilizable oocytes.168 Another explanation for this observation is that IGF-II, rather than IGF-I, is the important factor in the human dominant follicle. This possibility is supported by evidence indicating that IGF-II is the most abundant IGF in human ovarian follicles.150,151 Another possibility is that the Laron-type dwarf is deficient only in growth hormone-dependent IGF-I, and ovarian IGFs are not totally dependent on growth hormone.
Summary of Insulin-Like Growth Factor Action in the Ovary
IGF-II stimulates granulosa cell proliferation, aromatase activity, and progesterone synthesis.
IGF-II is produced in theca cells, granulosa cells, and luteinized granulosa cells In the pig and rat, the primary IGF is IGF-I.
Gonadotropins stimulate IGF production, and in animal experiments, this stimulation is enhanced by estradiol and growth hormone.
IGF-I receptors are present in theca and granulosa cells, and only IGF-II receptors are present in luteinized granulosa. IGF-II activates both IGF-I and IGF-II receptors.
The most abundant IGF in human follicles is IGF-II.
FSH inhibits binding protein synthesis, and thus maximizes growth factor availability.
Epidermal Growth Factor
Epidermal growth factor is a mitogen for a variety of cells, and its action is potentiated by other growth factors. Granulosa cells, in particular, respond to this growth factor in a variety of ways related to gonadotropin stimulation, including proliferation. Epidermal growth factor suppresses the up-regulation of FSH on its own receptor.39 Amphiregulin and Epiregulin, ligands that are similar to epidermal growth factor, are produced in luteinized granulosa cells in response to LH and induce progesterone synthesis in the corpus luteum.169
Transforming Growth Factor
TGF-α is a structural analog of epidermal growth factor and can bind to the epidermal growth factor receptor. TGF-β utilizes a receptor distinct from the epidermal growth factor receptor. These factors are thought to be autocrine growth regulators. Inhibin and activin are derived from the same gene family. TGF-β, secreted by theca cells, enhances FSH induction of LH receptors on granulosa cells, an action which is opposite that of epidermal growth factor.170 While this action can be viewed as a positive impact on granulosa cells, in the theca, TGF-β has a negative action, inhibiting androgen production.171 Growth differentiation factor 9 (GDF-9) is a member of the TGF-β family that originates in the oocyte and is essential for normal growth and development of the ovarian follicle.16
Fibroblast Growth Factor
This factor is a mitogen for a variety of cells and is present in all steroid-producing tissues.
Important roles in the ovarian follicle include stimulation of mitosis in granulosa cells, stimulation of angiogenesis, stimulation of plasminogen activator, inhibition of FSH up-regulation of its own receptor, and inhibition of FSH-induced LH receptor expression and estrogen production.39,172 These actions are opposite of those of transforming growth factor-β.
Platelet-Derived Growth Factor
This growth factor modifies cyclic AMP pathways responding to FSH, especially those involved in granulosa cell differentiation. Both platelet-derived growth factor and epidermal growth factor may also modify prostaglandin production within the follicle.
Angiogenic Growth Factors
Vascularization of the follicle is influenced by peptides in the follicular fluid, especially
vascular endothelial growth factor (VEGF), a cytokine produced in granulosa cells in response to LH.173,174 Luteal cells respond to human chorionic gonadotropin (hCG) with greater VEGF output, a probable mechanism contributing to the increased vascular permeability associated with ovarian hyperstimulation that can occur with exogenous gonadotropin administration (Chapter 31).175 Angiopoietins bind to an endothelial receptor (Tie-2) and provide an inhibitory influence on angiogenesis. Angiopoietin-1 is the active agent, opposed by angiopoietin-2, which competes for the Tie-2 receptor on endothelial cells. Differential expression of these angiogenic factors is involved in the coordinated growth and regression of follicles and the corpus luteum.176,177 and 178 The injection of VEGF and angiopoietin antagonists directly into dominant follicles in the monkey interferes with both the physical process of ovulation and the subsequent function of the corpus luteum.179
The Interleukin-1 System
Leukocytes are a prominent component of the ovarian follicle and a major source of interleukins. Interleukin-1 is a member of the cytokine family of immunomediators. The human ovary contains the complete interleukin-1 system (ligand and receptor). In the rat, interleukin-1 stimulates ovarian prostaglandin synthesis and perhaps plays a role in ovulation.180
Tumor Necrosis Factor-a (TNF-a)
TNF-α is also a product of leukocytes (macrophages). It very likely is a key player in the process of apoptosis, a feature of follicular atresia as well as luteolysis of the corpus luteum.
Other Peptides
The follicular fluid is a veritable protein soup! It is composed of exudates from plasma
and secretions from follicular cells. A variety of hormones can be found in the follicular fluid, as well as enzymes and peptides, which play important roles in follicular growth and development, ovulation, and modulation of hormonal responses.
Follicular fluid containsprorenin, the inactive precursor of renin, in a concentration that is about 12 times higher than plasma levels.181 LH stimulates its synthesis in the follicle, and there is a midcycle peak in prorenin plasma levels. The circulating levels of prorenin also increase (10-fold) during the early stages of pregnancy, the result of ovarian stimulation by the rise in human chorionic gonadotropin (hCG). These increases in prorenin from the ovary are not responsible for any significant changes in the plasma levels of the active form, renin. Possible roles for this ovarian prorenin-renin-angiotensin system include stimulation of steroidogenesis to provide androgen substrate for estrogen production, regulation of calcium and prostaglandin metabolism, and stimulation of angiogenesis. This system may affect vascular and tissue functions both within and outside the ovary.
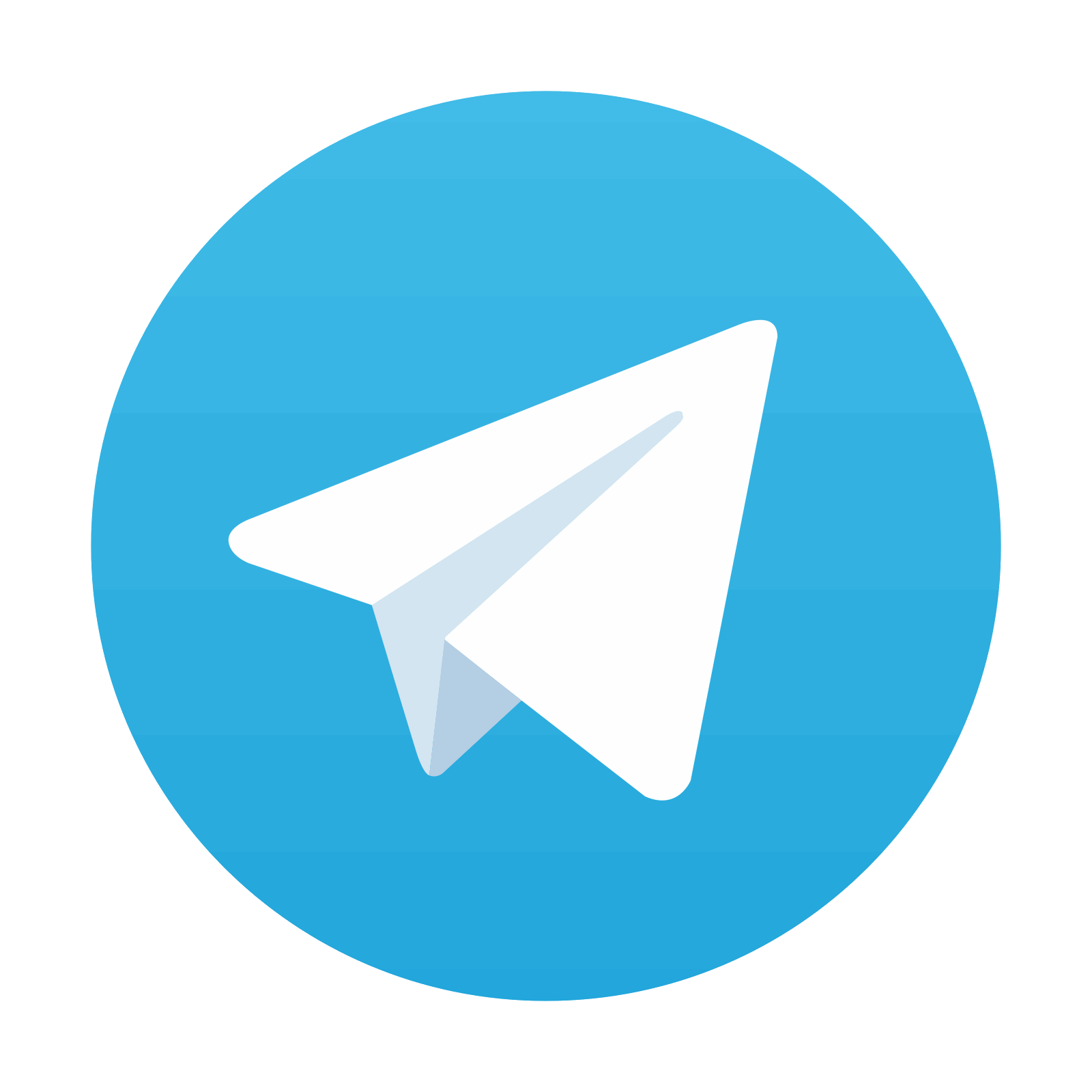
Stay updated, free articles. Join our Telegram channel

Full access? Get Clinical Tree
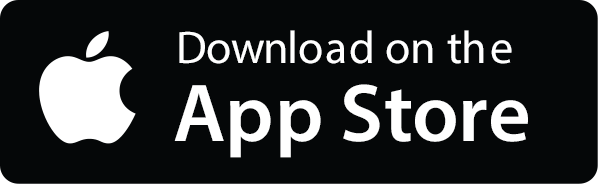
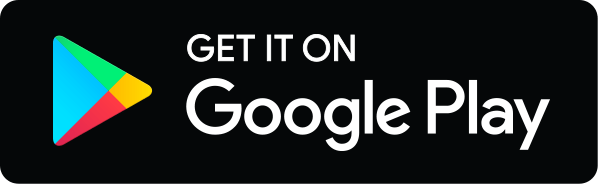
