Surgical Liver Disease
Max R. Langham Jr.
Alan W. Hemming
Division of Pediatric Surgery, University of Florida, Gainesville, Florida 32610-0286.
Division of Transplantation, University of Florida, Gainesville, Florida 32610-0286.
Surgical procedures form the basis of current treatment for a number of disorders affecting the liver. The pathophysiology of many of these disorders is incompletely understood, and effective medical therapy for most has not become a reality. Until such treatments are developed, resection of discrete lesions or replacement of the entire liver for diffuse disease will remain the cornerstone of effective treatment in conjunction with procedures designed to lessen the impact of portal hypertension. Improved operative techniques, coupled with significant advances in preoperative imaging, anesthesia, and intensive care, have created a golden age in liver surgery. This chapter briefly reviews the surgical anatomy of the liver and relevant hepatic physiology, and discusses technical aspects of liver resection, emphasizing preoperative and intraoperative decision making and postoperative care.
HISTORY
The history of surgical therapy for liver disease in children is short. Ladd and Gross only devoted slightly more than a page for the entire subject of liver tumors in their seminal text entitled Abdominal Surgery of Infancy and Childhood (1). Surgical exploration was advised with resection of benign lesions, but only one of the nine patients described had such a resection. Willis Potts, in his text The Surgeon and the Child (2) published in 1959, did not even mention liver resections. In 1974, Exelby and others surveyed the Surgical Section of the American Academy of Pediatrics and reported 227 children with malignant liver lesions (3). Fifty-two percent were resected for cure, with a 19% operative mortality. This compared favorably with the 33% operative mortality he had previously reported from a single institution (4). Operative mortality has fallen sharply since the 1980s, with several large contemporary series reporting operative mortality of 5% or less (5,6). This dramatic improvement is due to improved anesthesia support, coupled with a better understanding of the segmental anatomy of the liver, with subsequent abandonment of most nonanatomic resections.
In the 1970s, cure rates with resection alone and no chemotherapy were 60% for hepatoblastoma and 33% for hepatocellular carcinoma. King has nicely outlined the advent in the late 1970s of multiinstitutional cooperative studies of chemotherapy for these lesions (7). Preoperative chemotherapy became increasingly popular during the 1980s for children with potentially unresectable lesions (8,9,10,11,12). Initially reported as a method to improve resection rates for stage III or IV tumors, some authors now advocate preoperative chemotherapy for potentially resectable lesions (13,14). No properly controlled data have been reported that compare preoperative with postoperative chemotherapy for resectable lesions. Perioperative morbidity, mortality, cure rates, and long-term quality of life would be appropriate outcome measures of such a study. A series of more recent trials has confirmed improved cure rates for hepatoblastoma (5,15), but not hepatocellular carcinoma (16,17) with chemotherapy either before or after resection. Finally, since the 1990s, a resurgent interest has been seen in liver transplantation for malignant hepatic tumors (18,19).
ANATOMY
The liver is approximately 5% of total body weight in neonates and young infants (20), declining to 2% of total body weight in adolescents and adults. The anatomy of the liver has been described using various and different methods; however, surgical anatomy is based on the segmental nature of vascular and bile duct distribution (21,22,23,24).
The liver receives a dual blood supply from both the portal vein and the hepatic artery that run, along with the bile duct, within the Glissonian sheath or main portal pedicle.
The portal pedicle divides into right and left branches and then supplies the liver in a segmental fashion. Venous drainage is via the hepatic veins, which drain directly into the inferior vena cava. Hepatic segmentation is based on the distribution of the portal pedicles and their relation to the hepatic veins. Most historic depictions of the anatomy accurately locate the segments on the surface of the liver, but fail to provide a three-dimensional appreciation of the vascular anatomy, which is critical to the surgeon (Fig. 91-1). More recent depictions of the anatomy have emphasized these relationships and are therefore much more useful (Fig. 91-2). The three hepatic veins run in the portal scissurae and divide the liver into four sectors, which are in turn divided by the portal pedicles running in the hepatic scissurae. The liver is divided into right and left hemilivers by the middle hepatic vein. The right hepatic vein divides the right hemiliver into anterior and posterior sectors. The plane of the portal pedicle divides the anterior sector into an inferior segment 5 and a superior segment 8, and the posterior sector into an inferior segment 6 and a superior segment 7. The left hemiliver lies to the left of the middle hepatic vein and is divided into anterior and posterior sectors by the left hepatic vein. The anterior sector is divided by the umbilical fissure into segment 4 medially and segment 3 laterally. The segment posterior to the left hepatic vein is segment 2. The plane of the portal pedicle divides segment 4 into a superior segment 4a and an inferior segment 4b. Segment 1 is the caudate lobe, which lies between the inferior vena cava and the hepatic veins. The caudate lobe has variable portal venous, hepatic arterial, and biliary anatomy, and is essentially independent of the portal pedicle divisions and hepatic venous drainage. Segmental anatomy is important in considering hepatic resection because essentially any segment or combination of segments can be resected if attention is paid to maintaining vascular and biliary continuity to remaining segments, and to preserving an adequate residual mass of functional liver.
The portal pedicle divides into right and left branches and then supplies the liver in a segmental fashion. Venous drainage is via the hepatic veins, which drain directly into the inferior vena cava. Hepatic segmentation is based on the distribution of the portal pedicles and their relation to the hepatic veins. Most historic depictions of the anatomy accurately locate the segments on the surface of the liver, but fail to provide a three-dimensional appreciation of the vascular anatomy, which is critical to the surgeon (Fig. 91-1). More recent depictions of the anatomy have emphasized these relationships and are therefore much more useful (Fig. 91-2). The three hepatic veins run in the portal scissurae and divide the liver into four sectors, which are in turn divided by the portal pedicles running in the hepatic scissurae. The liver is divided into right and left hemilivers by the middle hepatic vein. The right hepatic vein divides the right hemiliver into anterior and posterior sectors. The plane of the portal pedicle divides the anterior sector into an inferior segment 5 and a superior segment 8, and the posterior sector into an inferior segment 6 and a superior segment 7. The left hemiliver lies to the left of the middle hepatic vein and is divided into anterior and posterior sectors by the left hepatic vein. The anterior sector is divided by the umbilical fissure into segment 4 medially and segment 3 laterally. The segment posterior to the left hepatic vein is segment 2. The plane of the portal pedicle divides segment 4 into a superior segment 4a and an inferior segment 4b. Segment 1 is the caudate lobe, which lies between the inferior vena cava and the hepatic veins. The caudate lobe has variable portal venous, hepatic arterial, and biliary anatomy, and is essentially independent of the portal pedicle divisions and hepatic venous drainage. Segmental anatomy is important in considering hepatic resection because essentially any segment or combination of segments can be resected if attention is paid to maintaining vascular and biliary continuity to remaining segments, and to preserving an adequate residual mass of functional liver.
![]() FIGURE 91-1. Segmental anatomy of the liver. Historically in the United States, the surgical anatomy of the liver was based on a division into the right and left hemilivers along Cantlies line. With improved understanding of the segmental anatomy of the liver and its variations, this has become oversimplified and should be abandoned. Obvious sources of confusion include the diagrammed terminology listing a right hepatectomy as including segments V, VI, VII, and VIII, whereas a right lobectomy includes these segments and segments I and IV [in common usage a right trisegmentectomy, although this terminology has also been criticized by Strasberg and others (23).] |
LIVER PHYSIOLOGY
Protecting and preserving a minimum of two segments of normal liver is necessary to preserve the liver’s central role in homeostasis. If parenchymal liver disease is present, a careful evaluation of the disease severity is imperative because resection of relatively small volumes of liver can result in morbidity, which is most commonly related to disruption of several of the wide variety of vital physiologic functions served by the liver. Complications and mortality are related more to the amount of functional liver left behind than to the amount resected. In normal liver, total hepatic function is related to the volume of
liver. Unfortunately, in patients with cirrhosis the histologic severity does not correlate with liver function. A variety of methods have been used to assess the function and correlate this with the amount of liver that can be safely resected (25,26,27). Indocyanine green clearance (ICG) is the best method tested in cirrhotic adults. An ICG residual at 15 minutes (ICG R15) of less than 15% suggests adequate function to allow safe hepatectomy, whereas a value of more than 25% suggests that no resection will be tolerated (28). The vital metabolic and physiologic role of the liver is discussed briefly as it relates to surgical resection.
liver. Unfortunately, in patients with cirrhosis the histologic severity does not correlate with liver function. A variety of methods have been used to assess the function and correlate this with the amount of liver that can be safely resected (25,26,27). Indocyanine green clearance (ICG) is the best method tested in cirrhotic adults. An ICG residual at 15 minutes (ICG R15) of less than 15% suggests adequate function to allow safe hepatectomy, whereas a value of more than 25% suggests that no resection will be tolerated (28). The vital metabolic and physiologic role of the liver is discussed briefly as it relates to surgical resection.
Arterial flow to the liver supplies 25% of the blood flow, but more than 50% of the oxygen delivery to the organ, and provides the sole blood supply to the major bile ducts (29). Ligation or embolization of the hepatic artery rarely causes hepatic necrosis in humans. Thrombosis of the artery after liver transplantation may cause an ischemic cholangiopathy (30,31,32) that can also be seen after devascularization of the bile duct during laparoscopic cholecystectomy (33). Hepatic arterial flow is regulated by both sympathetic and parasympathetic fibers derived from the vagus nerves, the celiac plexus, the lower thoracic ganglia, and the right phrenic nerves (34). Hepatic blood flow and metabolic function appear normal even after complete denervation of the liver associated with orthotopic liver transplantation (35). The impact of partial hepatic resection and hilar dissection on functions mediated by these neural plexi is largely unknown. A number of pharmacologic agents that may alter hepatic blood flow are currently being used after hepatic resection to improve liver regeneration. These include prostaglandin E1, N-acetyl cysteine, and others (36,37,38,39). There is little evidence that supports their use, and the precise mechanisms of action of the drugs are not well understood. However, we continue to use these agents in patients with marginal liver function after resection.
Venous blood flows from the gut and spleen via the portal vein into the liver, supplying about 75% to 80% of the total hepatic blood flow. There is usually little resistance to flow in this system, with the portal vein pressure within 5 mm Hg of that measured in the hepatic veins. Visceral nerves and enteric hormones regulate splanchnic blood flow, which increases during digestion (40,41), but
large changes in blood flow to the gut typically produce little or no change in total blood flow to the normal liver (42) because of regulation of hepatic arterial flow. There is some evidence that cirrhosis can disrupt this autoregulation, contributing to elevation in portal pressures (43).
large changes in blood flow to the gut typically produce little or no change in total blood flow to the normal liver (42) because of regulation of hepatic arterial flow. There is some evidence that cirrhosis can disrupt this autoregulation, contributing to elevation in portal pressures (43).
Acute portal hypertension is uncommon after uncomplicated anatomic liver resections, which usually result in a rise in portal pressure of only 2 to 3 mm Hg. Major hepatic resections in the face of preexisting portal hypertension are contraindicated because further increase in portal pressure causes congestion of the intestine and increased intraabdominal pressure, and can be associated with hepatorenal syndrome or bowel necrosis (44). Patients with long-standing portal hypertension are less likely to develop intestinal necrosis, but are at risk for variceal bleeding in the early postoperative period. Portal hypertension may negatively affect hepatic regeneration, with some experimental evidence suggesting that partial portal vein ligation before resection increases hepatic ornithine decarboxylase activity and thymidine kinase activity after resection (45). Separate studies suggest that portosystemic shunting may improve survival in rats after massive hepatectomy (46). Portal hypertension can be inferred preoperatively based on liver biopsy and physical examination, and can be definitely determined using hepatic vein wedge pressures, splenic pulp pressures, or transhepatic portal vein cannulation. There is no widely accepted method of predicting postresectional portal pressures preoperatively. Judgment even of an experienced hepatobiliary surgeon is unreliable. In children, we have frequently performed liver resections in the face of some portal hypertension with limited mortality, but significant morbidity. Results of total hepatectomy and liver transplantation in children with significant hepatic parenchymal disease are excellent, making it preferred to major hepatic resection in such children.
The effects of limited portal vein blood flow on the liver have been less well characterized. Portal vein stenosis or thrombosis is a serious postoperative problem that may lead to acute hepatic necrosis and death. Chronic partial obstruction of portal venous flow, such as that seen in cavernous transformation of the portal vein may be associated with inhibition of hepatic growth that may be reversible by establishing more normal portal flow with a Rex shunt (superior mesenteric vein or portal vein collateral shunted for intrahepatic left portal vein). Portal vein stenosis after complex hepatic resections may be associated with impaired hepatic regeneration through similar mechanisms, but paucity of data renders this observation speculative.
Liver regeneration after partial hepatectomy appears to be dependent on tumor necrosis factor alpha (TNF-α). Animals treated with anti TNF-α antibodies do not regenerate their liver after partial hepatectomy (47). Regenerative liver failure occurs in mice lacking type 1 TNF receptors (48). Cellular signaling begins rapidly with increased expression of the antiapoptotic transcription factor, nuclear factor kB (NF-kB), in a TNF-α–dependent manner within 15 minutes of partial hepatectomy (48,49). In healthy animals, DNA synthesis is detectable 24 hours after partial hepatectomy and reaches a peak within 72 hours posthepatectomy (50). Poor nutritional status, sepsis, and chemotherapy may negatively affect hepatic regeneration after resection, which is in part regulated by insulin (51,52). Ischemic preconditioning has been proposed as a way to make use of these cellular changes to improve tolerance to hepatic ischemia during resection.
Bile is produced by active transport across the canalicular membranes of the hepatocyte. Bile flow is in part dependent on bile salts secreted by the hepatocytes and in part independent of this secretion, and related to glutathione and bicarbonate secretion (53,54). The composition of bile is altered in the bile ductules and ducts. Both hormones and second messengers regulate bile flow in response to various stimuli. Preoperative cholestasis in children who are candidates for liver resection is most often seen in patients with chronic hepatitis, and is relatively rare in children living in the United States. Obstruction of the bile ducts due to tumors is also uncommon in children, but has been reported in patients with non-Hodgkin’s lymphoma (55), neuroblastoma (56), rhabdomyosarcoma (57), and pancreatoblastoma (58), and from benign inflammatory masses (59,60). Significant preoperative cholestasis should be carefully evaluated before any major liver resection because it implies diminished hepatic function and an increased risk of complications or death.
Cholestasis may complicate liver resection and may be due to an inadequate residual functional liver mass, devascularization of the bile duct during resection, decreased hepatic perfusion, sepsis, mechanical obstruction of the bile ducts, or a bile leak. Although bilirubin commonly increases slightly after an uncomplicated resection, any sustained increase in serum bilirubin should prompt an evaluation of the hepatobiliary tree. Cholestasis is hepatotoxic and is a potent stimulant of hepatic fibrosis. This may rapidly become clinically important, emphasizing the necessity of prompt intervention (61,62).
The liver produces a large volume of lymph, estimated in normal adults to be as high as 1 to 3 L per day. Increased sinusoidal pressure seen in cirrhosis or Budd Chiari syndrome may cause dramatic increases in lymphatic flow (63). The effect of hepatic resection on lymph flow has not been studied to our knowledge. Ascites formation after liver resection may be due to increased sinusoidal pressure as portal pressures rise. Lymph leaks due to complete or incomplete node dissections are usually self-limited. Treatment of ascites in either case is dependent on reducing sodium intake and limiting total daily fluids and the use of diuretics.
The liver plays a central role in glucose homeostasis. Hepatic glycogen is a primary substrate for glucose
production via glycogenolysis during fasting and is a major site of gluconeogenesis. Net glucose production by the liver is stimulated by catecholamines, glucagon, and parasympathetic (64) stimulation. Insulin inhibits hepatic production of glucose. After a major liver resection, serum glucose should be monitored. Hypoglycemia, although unusual, occurs more commonly in children than adults undergoing equivalent liver resections. This is particularly true in children younger than 2 years of age because hepatic glycogen stores are small. Profound hypoglycemia suggests significant hepatic insufficiency and is an ominous finding.
production via glycogenolysis during fasting and is a major site of gluconeogenesis. Net glucose production by the liver is stimulated by catecholamines, glucagon, and parasympathetic (64) stimulation. Insulin inhibits hepatic production of glucose. After a major liver resection, serum glucose should be monitored. Hypoglycemia, although unusual, occurs more commonly in children than adults undergoing equivalent liver resections. This is particularly true in children younger than 2 years of age because hepatic glycogen stores are small. Profound hypoglycemia suggests significant hepatic insufficiency and is an ominous finding.
Acute hepatic failure results in a stereotypical neurohormonal response that leads to water and sodium retention that also occurs after major resectional liver surgery and represents the most common fluid and electrolyte problem the hepatobiliary surgeon faces in practice. Sodium retention occurs due to an increased reabsorption of sodium in the proximal and distal tubules (65). This is mediated by the renin, angiotensins, and aldosterone system with possible contribution of the sympathetic nervous stimulation, but not atrial natriuretic factor (66). It results in ascites and edema. Occasionally, the ascites and fluid overload will be severe enough to result in respiratory difficulty, pulmonary edema, and pleural effusions. Concomitant retention of free water and an exaggerated antidiuretic hormone response after surgery frequently results in hyponatremia that can be profound. Water retention can be linked to (1) reduced delivery of filtrate to the ascending limb of the loop of Henle, (2) reduced renal synthesis of prostaglandins, and (3) increased secretion of arginine vasopressin (67). Failure to recognize sodium retention as one of the causes of the water retention can be problematic. Hyponatremia in these patients is almost always due to excess water retention and is not due to sodium depletion. Administration of sodium in this setting makes matters worse, not better. Those patients with severely compromised liver function and portal hypertension can suffer renal artery vasoconstriction and acute tubular necrosis (hepatorenal syndrome) (68).
Severe liver disease or massive hepatic resection can affect the child’s ability to metabolize narcotics and other drugs. In the setting of a major liver resection, the half-life of these drugs is likely to be prolonged, although specific data of clinical relevance is difficult to find. The surgeon should be aware that drug toxicity may be increased after liver resection; thus, drug levels should be monitored where appropriate, and drug doses modified if necessary.
LIVER PATHOLOGY AND PREOPERATIVE EVALUATION
Primary tumors of the liver constitute the most common indication for liver resection in children. A more complete discussion of these tumors is presented in Chapter 38. Table 91-1 lists the pathologic type of liver tumors reported by Weinberg and Finegold (69) as well as those seen at our institution. Malignant hepatic lesions are more common than benign liver tumors in children. In children, approximately two-thirds of liver masses are malignant with hepatoblastoma accounting for 65%, hepatocellular carcinoma 25%, and sarcomas 10% (69). Benign lesions comprise about one-third of hepatic masses in children and include hemangiomas, hemangioendotheliomas, mesenchymal hamartomas, inflammatory pseudotumors, and adenomas. Focal nodular hyperplasia is rare, but has been reported in children (71,72). A number of points present in the history, and physical examination can be helpful in appropriately evaluating a child with a mass in the liver.
TABLE 91-1 Pathologic Types of Primary Liver Tumors in Children.a | ||||||||||||||||||||||||||||||||||||||||||||||||||||||
---|---|---|---|---|---|---|---|---|---|---|---|---|---|---|---|---|---|---|---|---|---|---|---|---|---|---|---|---|---|---|---|---|---|---|---|---|---|---|---|---|---|---|---|---|---|---|---|---|---|---|---|---|---|---|
|
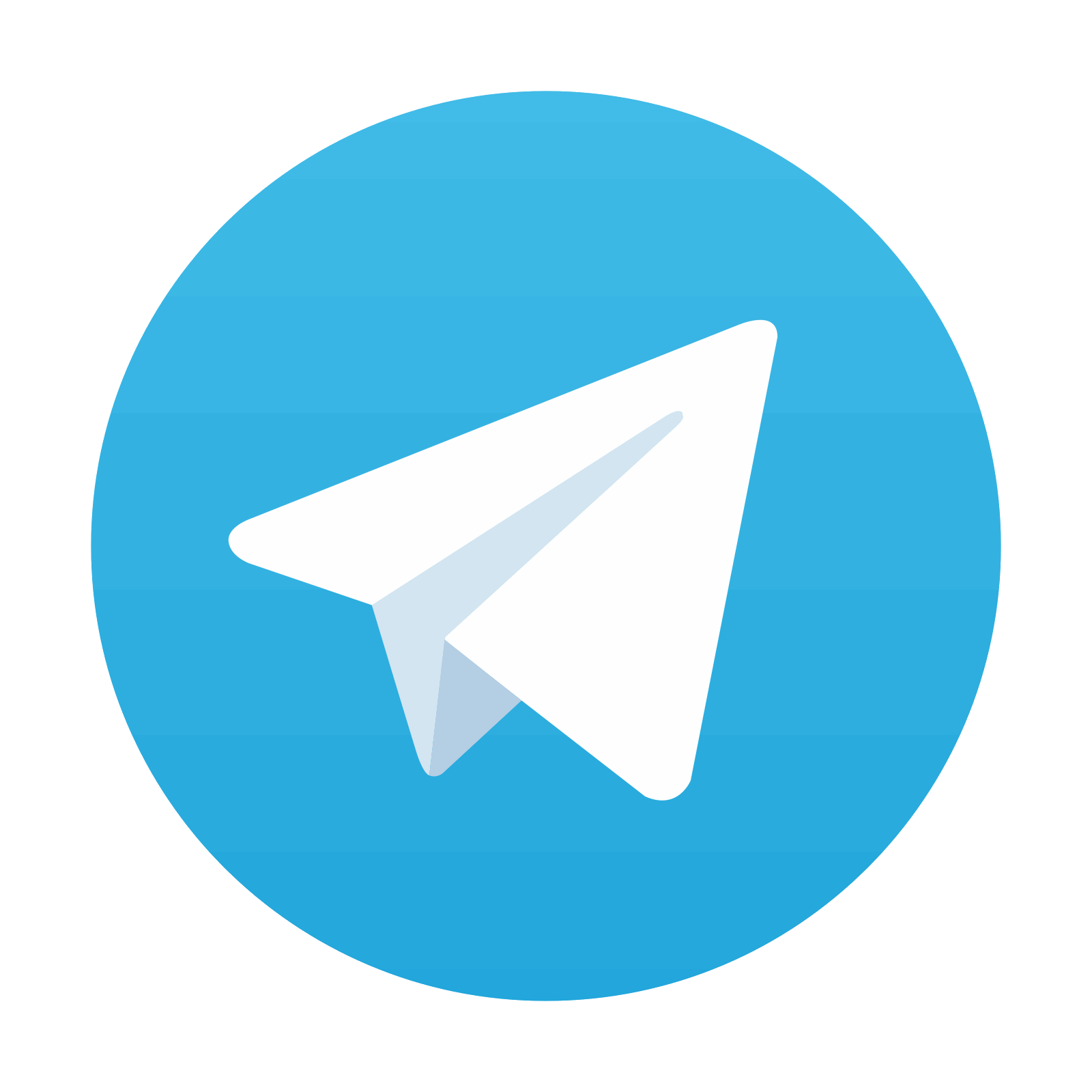
Stay updated, free articles. Join our Telegram channel

Full access? Get Clinical Tree
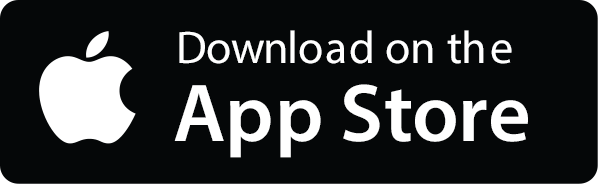
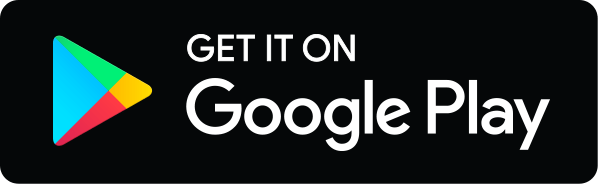