Sperm and Egg Transport, Fertilization, and Implantation
![]() |
Among his many accomplishments, Galileo Galilei gave to science, in 1609, two important instruments, the telescope and the microscope.1 Anton van Leeuwenhoek of Delft, Holland, was fascinated by Galileo’s microscope. Leeuwenhoek was a draper and had no medical or scientific training, yet he became a fellow of the Royal Society of London to which he submitted 375 scientific papers. In 1677, Leeuwenhoek described (fairly accurately) the “little animals of the sperm.” It was another 198 years before Wilhelm August Oscar Hertwig, in Germany, demonstrated the union of sperm and egg, fertilization, in the sea urchin.
The coming together of sperm and egg is one of the essentials of reproduction; however, the remote site of this event and the enclosed origins of the participants made fertilization a difficult subject for study. This changed with the advent of in vitro fertilization. Greater understanding of sperm and egg development and union is one of the major benefits of the clinical application of the assisted reproductive technologies. This chapter examines the mechanisms involved in sperm and egg transport, fertilization, and implantation.
Sperm Transport
The evolution of scrotal mammals and the adoption of internal fertilization are associated with sperm maturation that occurs outside of the testes. This includes epididymal maturation in the male and capacitation in the female before fertilization. The need for capacitation (the final step required to acquire the ability to fertilize) may be an evolutionary consequence of the development of a storage system for inactive sperm in the caudal epididymis.2
![]() |
The epididymis is divided into four segments, the initial segment, the caput where the sperm begin their process of maturation, the corpus where maturation continues, and the cauda, the site of final maturation and storage.3 The sperm reach the caudal epididymis approximately 72 days after the initiation of spermatogenesis. At this time, the head of the sperm contains a membrane-bound nucleus capped by the acrosome, a large vesicle of proteolytic enzymes. The inner acrosomal membrane is closely apposed to the nuclear membrane, and the outer acrosomal membrane is next to the surface plasma membrane. The flagellum is a complex structure of microtubules and fibers, surrounded at the proximal end by mitochondria. Motility and the ability to fertilize are acquired gradually as the sperm pass into the epididymis.
The caudal epididymis stores sperm available for ejaculation. The ability to store functional sperm provides a capacity for repetitive fertile ejaculations. Preservation of optimal sperm function during this period of storage requires adequate testosterone levels in the circulation and maintenance of the normal scrotal temperature.4 The importance of temperature is emphasized by the correlation of reduced numbers of sperm associated with episodes of body fever. The evolution of the scrotum served the purpose of achieving the cooler temperatures required for effective sperm storage.
Surface proteins are acquired by the sperm in the epididymis, proteins that must be removed in the process of capacitation, discussed later. It can be argued that the epididymis is limited to a storage role because sperm that have never passed through the epididymis and that have been obtained from the vasa efferentia in men with a congenital absence of the vas deferens can fertilize the human oocyte in vitro and result in pregnancy with live birth.5 Indeed, the injection of sperm obtained by testicular biopsy directly into an oocyte (intracytoplasmic sperm injection) is very successful in achieving fertilization and pregnancy.6 Epididymal functions should not be dismissed, however, because with direction injection into the oocyte, surface protein action at the oocyte membrane is bypassed.
The use of sperm from men with sperm abnormalities should be pursued with some caution. Sperm obtained from men with Y chromosome microdeletions involving the AZFc region of Yq11 can transmit the deletion to male children, who then will also likely be infertile. In addition, men with certain Y microdeletions, including a portion of AZFc, may have an increased susceptibility for developing testicular germ cell tumors. The outcome in subsequent generations must be assessed and appropriate genetic screening must be developed to avoid the transmission of subtle but important genetic alterations. Meanwhile, men with severe oligospermia or azoospermia should receive appropriate genetic counseling and should be offered testing for Y microdeletions before their sperm are used for intracytoplasmic sperm injection (ICSI).
Semen forms a gel almost immediately following ejaculation but then is liquefied in 20-30 minutes by enzymes derived from the prostate gland. The alkaline pH of semen provides protection for the sperm from the acid environment of the vagina. This protection is transient, and most sperm left in the vagina are immobilized within 2 hours. The more fortunate sperm, by their own motility, gain entrance into the tongues of cervical mucus that layer over the ectocervix. These are the sperm that enter the uterus; the seminal plasma is left behind in the vagina. This entry is rapid, and sperm have been found in mucus within 90 seconds of ejaculation.7 The destruction of all sperm in the vagina 5 minutes after ejaculation does not interfere with fertilization in the rabbit, further attesting to the rapidity of transport.8
Contractions of the female reproductive tract occur during coitus, and these contractions may be important for entry of sperm into the cervical mucus and further transport. Presumably successful entry is the result of combined female and male forces (the flagellar activity of the sperm). The success of therapeutic insemination, however, indicates that coitus and female orgasm are not essential for sperm transport.
![]() |
The sperm swim and migrate through pores in the cervical mucus that are smaller than the sperm head; therefore, the sperm must actively push their way through the mucus.9 One cause of infertility, presumably, is impaired sperm movement that prevents this transport through the mucus. This movement is probably also influenced by the interaction between the mucus and the surface properties of the sperm head; for example, sperm antibodies on the sperm head inhibit sperm movement in the mucus.10 Abnormal morphology of the sperm head is often associated with impaired flagellar function; however, abnormal head morphology alone can be a cause of poor mucus penetration.11,12 A sperm coating protein (beta-defensin 126), acquired in the caudal epididymis, carries a high negative charge and is critical for movement through the cervical mucus.13 It is generally believed that the cervical mucus has a filtering action; abnormal and less “capable” sperm have difficulty getting through.14
Uterine contractions and sperm motility propel the sperm upward, and in the human, sperm can be found in the tube 5 minutes after insemination.15 Labeled albumin is present in the tubes within 30 seconds after intrauterine instillation.16 It is possible that the first sperm to enter the tube are at a disadvantage. In the rabbit these early sperm have only poor motility, and there is frequent disruption of the head membranes.17 The sperm in this vanguard are unlikely to achieve fertilization. Other sperm that have colonized the cervical mucus and the cervical crypts then make their way more slowly to the ampulla of the tube in order to meet the egg. The number of sperm in the cervical mucus is relatively constant for 24 hours after coitus, and after 48 hours there are relatively few remaining in the mucus.18 Although the isthmic region of the tube functions as a sperm reservoir in many species, this does not appear to be the case in human fallopian tubes.19
Human sperm have been found in the fallopian tube as long as 80 hours after intercourse, and these sperm can still perform normally with zona-free hamster oocytes.20 In animals, the fertilizable lifespan is usually one-half the motile lifespan.
The attrition in sperm numbers from vagina to tube is substantial.21 Of an average of 200 to 300 million sperm deposited in the vagina, at most only a few hundred (rarely reaching 1,000), and often less, achieve proximity to the egg.19 Greater numbers are observed in the tubal ampulla at the time of ovulation. The major loss occurs in the vagina, with expulsion of semen from the introitus playing an important role. Other causes for loss are digestion of sperm by vaginal enzymes and phagocytosis of sperm along the reproductive tract. There are also reports of sperm burrowing into or being engulfed by endometrial cells. Many sperm continue past the oocyte to be lost into the peritoneal cavity. The cervix serves as a reservoir, providing a supply of sperm for up to 72 hours.
![]() |
Within the fallopian tube, sperm that are not yet capacitated are bound to the epithelial cells. When these sperm release and undergo capacitation, they display a new pattern of movement that has been called hyperactivated motility.22,23,23 This motility may be influenced by an interaction with the tubal epithelium that results in greater speed and better direction as well as prevention of attachment and entrapment.
Structure of the Cervical Mucus
The cervical mucus is a complex structure that is not homogeneous.24 The mucus is secreted in granular form, and a networked structure of the mucus is formed in the cervical canal. Thus, not all areas of the cervical mucus are equally penetrable by the sperm. It is proposed, based on animal studies, that the outward flow of the cervical mucus establishes a linear alignment of parallel strands that direct the sperm upward. Pressurization of the mucus by contractions of the uterus further aid this alignment and may contribute to the speed of sperm transport. Responding to the midcycle estrogen peak, cervical mucus production, water content, and space between its large glycoproteins reach a maximum in the immediate preovulatory period. The process of capacitation is initiated, but not completed during the sperm’s passage through the cervix.
Capacitation
The discovery, in 1951, that rabbit and rat spermatozoa must spend some hours in the female tract before acquiring the capacity to penetrate ova stimulated intensive research efforts to delineate the environmental conditions required for this change in the sperm to occur.25,26 The process by which the sperm were transformed was called capacitation, the cellular changes that ejaculated spermatozoa must undergo in order to fertilize.27 Attention was focused on the hormonal and time requirements and the potential for in vitro capacitation. Capacitation occurs while the sperm are in the fallopian tube and is characterized by three accomplishments:
-
The ability to undergo the acrosome reaction.
-
The ability to bind to the zona pellucida.
-
The acquisition of hypermotility.
Capacitation changes the surface characteristics of sperm, as exemplified by removal of seminal plasma factors that coat the surface of the sperm, modification of the surface charge, and restriction of receptor mobility. The protein beta-defensin 126 (DEFB126), derived from the epididymis, is the major coating protein of sperm that facilitates movement through the cervical mucus; its release from the sperm head is essential in order for the sperm to bind to the zona pellucida of the ovum.13,28 Sperm proteomics have demonstrated a huge collection of receptors coated on the surface of sperm.29,30 The purpose of the coating proteins may be to produce a reservoir of sperm in the fallopian tubes by promoting the binding of sperm to tubal epithelial cells.31
![]() |
The surface changes are associated with modifications of sperm cell membrane sterols, lipids, and glycoproteins that cause decreased stability of the plasma membrane and the membrane lying immediately under it, the outer acrosomal membrane. The membranes undergo further, more striking modifications when capacitated sperm reach the vicinity of an oocyte or when they are incubated in follicular fluid. There is a breakdown and merging of the plasma membrane and the outer acrosomal membrane, the acrosome reaction.32 This allows egress of the enzyme contents of the acrosome, the caplike structure that covers the sperm nucleus. These enzymes, which include hyaluronidase, a neuraminidase-like factor, cumulus-dispersing enzyme, and a protease called acrosin, are all thought to play roles in sperm penetration of the egg investments. The changes in the sperm head membranes also prepare the sperm for fusion with the egg membrane. It is the inner acrosomal membrane that fuses with the oocyte plasma membrane. The acrosome reaction can be induced by zona pellucida proteins of the oocyte and by human follicular fluid in vitro.33,34 In addition, capacitation endows the sperm with hypermotility, and the increased velocity of the sperm is a very critical factor in achieving zona penetration.22
The events that constitute the process of capacitation are regulated by the redox status of the sperm cell.35,36 Redox reactions induce tyrosine phosphorylation, an absolute requirement for capacitation. These reactions are dependent on a critical increase in intracellular calcium concentrations due to an influx of extracellular calcium, believed to be induced by progesterone. Sperm are stimulated to undergo capacitation when they encounter the alkaline change in pH at the time of ovulation, a response of the fallopian tubes to the midcycle hormonal changes.
Although capacitation classically has been defined as a change sperm undergo in the female reproductive tract, specifically in the fallopian tubes, it is apparent that sperm of some species, including the human, can acquire the ability to fertilize after a short incubation in defined media and without residence in the female reproductive tract. Therefore, success with assisted reproductive technologies is possible. In vitro capacitation requires a culture medium that is a balanced salt solution containing energy substrates such as lactate, pyruvate, and glucose and a protein such as albumin, or a biologic fluid such as serum or follicular fluid. Sperm-washing procedures probably remove factors that coat the surface of the sperm, one of the initial steps in capacitation. The removal of cholesterol from the sperm membrane is believed to prepare the sperm membrane for the acrosome reaction.37 The loss of cholesterol regulates the expression of sperm cell membrane surface lectins that are involved in sperm surface receptors for the zona pellucida.38 The time required for in vitro capacitation is approximately 2 hours.39
The final dash to the oocyte is aided by the increased motility due to the state of hyperactivity. This change in motility can be measured by an increase in velocity and flagellar beat amplitude. Perhaps the increase in thrust gained by this hyperactivity is necessary for avoiding attachment to tubal epithelium and achieving penetration of the cumulus and zona pellucida.
Key Steps in Sperm Transport
-
Approximately 72 days are required to produce spermatozoa, a time period followed by storage in the epididymis prior to ejaculation.
-
Sperm enter the cervical mucus and then the fallopian tubes within minutes, but only a few hundred sperm or less reach the oocyte. The cervix serves as a reservoir of sperm for up to 72 hours.
-
Capacitation, a process initiated during the sperm’s passage through the cervix and completed in the fallopian tube or during in vitro incubation in an appropriate medium, is characterized by the acquired ability of sperm to undergo the acrosome reaction, to bind to the zona pellucida, and to acquire hyperactivated motility.
-
The acrosome reaction is due to the modification and breakdown, followed by a merger, of the sperm cell membrane and the outer acrosomal membrane, allowing the release of enzymes and changes in the inner acrosomal membrane, necessary for fusion with the oocyte cell membrane.
Egg Transport
The oocyte, at the time of ovulation, is surrounded by granulosa cells (the cumulus oophorus) that attach the oocyte to the wall of the follicle. The zona pellucida, a noncellular porous layer of glycoproteins secreted by the oocyte, separates the oocyte from the granulosa cells. The granulosa cells communicate metabolically with the oocyte by means of gap junctions between the oocyte plasma membrane and the cumulus cells. In response to the midcycle surge in luteinizing hormone (LH), maturation of the oocyte proceeds with the resumption of meiosis as the oocyte completes the first meiotic division, enters into the second meiotic division, and arrests in the second metaphase. Just before ovulation, the cumulus cells retract their cellular contacts from the oocyte. The disruption of the gap junctions induces maturation and migration of the cortical granules to the outer cortex of the oocyte.40 Prior to ovulation, the oocyte and its cumulus mass of cells prepare to leave their long residence in the ovary by becoming detached from the follicular wall.
Egg transport encompasses the period of time from ovulation to the entry of the morula into the uterus. The egg can be fertilized only during the early stages of its sojourn in the fallopian tube. Within 2-3 minutes of ovulation in some animals, the cumulus and oocyte are in the ampulla of the fallopian tube. It takes longer in humans.
In rats and mice the ovary and distal portion of the tube are covered by a common fluidfilled sac. Ovulated eggs are carried by fluid currents to the fimbriated end of the tube. In contrast, in primates, including humans, the ovulated eggs adhere with their cumulus mass of follicular cells to the surface of the ovary. The fimbriated end of the tube sweeps over the ovary in order to pick up the egg. Entry into the tube is facilitated by muscular movements that bring the fimbriae into contact with the surface of the ovary. Variations in this pattern surely exist, as evidenced by women who achieve pregnancy despite having only one ovary and a single tube located on the contralateral side. Furthermore, eggs deposited in the cul-de-sac by transvaginal injection are picked up by the tubes.41
Although there can be a small negative pressure in the tube in association with muscle contractions, oocyte pickup is not dependent on a suction effect secondary to this negative pressure. Ligation of the tube just proximal to the fimbriae does not interfere with pickup.42
The fallopian tubes are lined by an epithelium that undergoes cyclic changes comparable to the endometrium, in response to the hormonal changes of the menstrual cycle.43 The epithelium is composed of nonciliated cells and ciliated cells. The nonciliated cells are characterized by major secretory activity during the follicular phase of the cycle, culminating in the release of cytoplasmic components during the passage of the egg, perhaps providing important metabolic factors for transport and implantation. The cilia on the surface of the fimbriae (where they are present in greater concentrations) display adhesive sites, and these seem to have prime responsibility for the initial movement of the egg into the tube. This movement is dependent on the presence of follicular cumulus cells surrounding the egg, because removal of these cells prior to egg pickup prevents effective egg transport.
In the ampulla of the tube the many cilia beat synchronously in the direction of the uterus, and in the fimbria the ciliary beat is faster in the secretory phase of the menstrual cycle.44 In women and monkeys, this unidirectional beat is also found in the isthmus of the tube. The specific contribution of the cilia to egg transport in the ampulla and isthmus is an unresolved question. Most investigators have credited muscular contractions of the tubes as the primary force for moving the egg.45 However, interference with muscle contractility in the rabbit did not block egg transport.46 Reversing a segment of the ampulla of the tube so that the cilia in this segment beat toward the ovary interferes with pregnancy in the rabbit without blocking fertilization. The fertilized ova are arrested when they come in contact with the transposed area.47 This suggests that ciliary beating is crucial for egg transport. Spontaneous pregnancies have been reported in women who suffer from Kartagener’s syndrome in which there is a congenital absence of dynein arms (a protein structure associated with motility) in all bodily cilia, and thus the cilia do not beat.48 However, motility of cilia in the tube may be disordered and not totally absent. Nevertheless, the pregnancies in women with Kartagener’s syndrome indicate the importance of uterine and fallopian tube muscular peristalsis.49 Human fallopian tube muscular contractions are stimulated by prostaglandins E2 and F2α, and decreased by progestins, hCG, and oxytocin.50
Transvaginal endoscopic observation of actual ovum and cumulus oophorous pickup in women revealed that the process is relatively slow (more than 15 minutes), the fimbriae on the ovulating side are distinguished by being erect (probably due to engorged blood vessels and suggesting a local ovarian influence), and the only observable active mechanism involved ciliary movement.51,52 It is probable, therefore, that in normal circumstances, smooth muscle contractions and the flow of secretory fluid in response to ciliary activity work together to accomplish egg transport.
In most species, transport of the ovum (the fertilized oocyte) through the tube requires approximately 3 days.53 The time spent within the various parts of the tube varies from one species to another. Transport through the ampulla is rapid in the rabbit, whereas in women the egg spends about 80 hours in the tube, 90% of which is in the ampulla at the junction of the ampulla with the isthmus. It is in this location that fertilization and dispersion of the cumulus cells are completed.
Attempts to modify tubal function as a method for understanding its physiology have involved three major pharmacologic approaches: (1) altering levels of steroid hormones, (2) interference with or supplementation of adrenergic stimuli, and (3) treatment with prostaglandins. Although there is abundant literature on the effects of estrogen and progesterone on tubal function, it is clouded by the use of different hormones, different doses, and different timing of injections. Because of these variations, it is difficult to obtain a coherent picture and to relate the experimental results to the in vivo situation. In general, pharmacologic doses of estrogen favor retention of eggs in the tube. This “tube-locking” effect of estrogen can be partially reversed by treatment with progesterone.
![]() |
The isthmus of the tube has an extensive adrenergic innervation. Surgical denervation of the tube, however, does not disrupt ovum transport. Prostaglandins (PG) of the F series stimulate muscle activity of the tube. Although PGF2α stimulates human oviductal motility in vivo, it does not cause acceleration of ovum transport.
Is there an essential anatomic segment of the tube? Excision of the ampullary-isthmus junction in rabbits does not prevent fertility.54 This is equally true if small segments of the ampulla are removed, and pregnancy can occur even if the entire isthmus and uterotubal junction are excised. Although the fimbriae are thought to play a crucial role in fertility, spontaneous pregnancies have been reported following sterilization by fimbriectomy or following surgical repair of tubes whose fimbriated ends had been excised.55,56 The fallopian tube appears to readily adapt to anatomic changes and restrictions.
In most species, a period of residence in the tube appears to be a prerequisite for full development. Rabbit eggs can be fertilized in the uterus, but they do not develop unless transferred to the tubes within 3 hours of fertilization.57 This implies that there may be a component in uterine fluid during the first 48 hours following ovulation that is toxic to the egg.57 Indirect evidence of an inhospitable environment is also provided by studies indicating that there must be synchrony between development of the endometrium and the egg for successful pregnancy to occur.58,59 If the endometrium is in a reduced or advanced stage of development compared with the egg, fertility is compromised. In addition, the blastocyst must undergo cleavage and development in order to gain the capability to implant in the uterus. Thus, it is conceptually useful to view the fallopian tube not as an active transport mechanism, but as a structure that provides an important holding action. This functional behavior is coordinated by the increasing estrogen and progesterone levels after ovulation, although local embryonic signals may also be involved.
Successful pregnancies have occurred in the human following the Estes procedure, in which the ovary is transposed to the uterine cornua.60 Eggs are ovulated directly into the uterus, completely bypassing the tube. Moreover, when fertilized donor eggs are transferred to women who are receiving hormone supplementation, there are several days during the treatment cycle when the blastocysts will implant. This crucial difference between animal and human physiology is of more than academic importance. There has been speculation about the use of drugs that could accelerate tubal transport as a means of providing contraception by ensuring that the egg would reach the uterus when it was in an unreceptive state. Although this may work in animals, it is of doubtful value in the human because perfect synchrony is not required.
Animal and human reproduction also differ in the occurrence of ectopic pregnancy. Ectopic pregnancies are rare in animals, and in rodents they are not induced even if the uterotubal junction is occluded immediately following fertilization. The embryos reach the blastocyst stage and then degenerate.
![]() |
Key Steps in Egg Transport
-
After ovulation, the oocyte and its surrounding cumulus are in the ampulla of the fallopian tube within 15-20 minutes.
-
Tubal transport depends on smooth muscle contractions and ciliary-induced flow of secretory fluid.
-
The fallopian tube provides an important holding action to allow time for the endometrium to become receptive and the blastocyst to become capable of implantation, a time period of approximately 80 hours, 90% of which is in the ampulla.
Oocyte Maturation
Oocyte maturation, as reviewed in Chapter 6, is regulated by the sex hormones and the complex interaction among an array of growth factors and cytokines in the follicular fluid. In nonmammalian species, a nongenomic action of progesterone causes an increase in intracellular calcium concentrations. In human oocytes, an influx of extracellular calcium occurs in response to estradiol, followed by secondary rises in calcium ions from intracellular stores, characterized by wavelike oscillations.61 This is a nongenomic response to estradiol at the cell surface, and the transient increases in intracellular calcium improve the quality of the oocyte and contribute to the capability for fertilization.
Calcium oscillations are a property common to mammalian oocytes and are also an early reaction to the fertilizing spermatozoan.62 Neither the presence of estradiol nor the calcium oscillations are required for oocytes to resume meiosis. However, improved fertilization following estradiol-induced calcium increases indicates an important role for intrafollicular estradiol in overall oocyte maturation.
Fertilization
The fertilizable life of the human oocyte is unknown, but estimates range between 12 and 24 hours. However, immature human eggs recovered for in vitro fertilization can be fertilized even after 36 hours of incubation. Equally uncertain is knowledge of the fertilizable lifespan of human sperm. The most common estimate is 48-72 hours, although motility can be maintained after the sperm have lost the ability to fertilize. The extreme intervals that have achieved pregnancy documented after a single act of coitus are 6 days prior to and 3 days after ovulation.63 The great majority of pregnancies occur when coitus takes place within the 3-day interval just prior to ovulation.64
Contact of sperm with the egg, which occurs in the ampulla of the tube, may not be random; there is some evidence for sperm-egg communication that attracts sperm to the oocyte.65,66 and 67 This chemotactic responsiveness of sperm requires the changes that take place in the capacitation process.68 Thus, this may be a system to select a sperm that is fully capable of fertilization.
The cumulus oophorus undergoes a preovulatory expansion that has at least two important roles. The ampullary space of the human fallopian tube is relatively large (compared with the oocyte), and the expanded cumulus may serve to increase the chances of an encounter with one of the few spermatozoa that have reached the far section of the tube. In addition, this change may facilitate sperm passage through the cumulus. Sperm pass through the cumulus without the release of acrosomal enzymes.69 It has been suggested, based on in vitro experiments, that the cumulus is essential for full development of the fertilizing ability of sperm; however, removal of the cumulus does not prevent sperm penetration and fertilization.
Despite the evolution from external to internal fertilization over a period of about 100 million years, many of the mechanisms have remained the same.70,71 and 72 The acellular zona pellucida that surrounds the egg at ovulation and remains in place until implantation has two major functions in the fertilization process:
-
The zona pellucida contains ligands for sperm, which are, with some exceptions, relatively species-specific.
-
The zona pellucida undergoes the zona reaction in which the zona becomes impervious to other sperm once the fertilizing sperm penetrates, and thus it provides a bar to polyploidy.73
Sperm bind to the zona pellucida for about a minute and then penetrate it rapidly, mediated by acrosin, a trypsin-like proteinase that is bound to the inner acrosomal membrane of the sperm.72,74,75 The pivotal role assigned to acrosin has been disputed. For example, manipulations that increase the resistance of the zona to acrosin do not interfere with sperm penetration, and thus sperm motility may be the critical factor. The zona pellucida is a porous structure due to the many glycoproteins assembled into long, interconnecting filaments. Nevertheless, a preponderance of evidence favors tenacious binding of capacitated spermatozoa to the zona pellucida as a requirement for penetration, although it is clear that penetration requires physical thrust with active motility not only of the tail but of the head as well. Indeed, sperm undergo rapid lateral oscillations of the head about a fulcrum at the head-tail junction, suggesting a scythe-like action on the zona.2,72
The acrosome is a ly sosome-like organelle in the anterior region of the sperm head, lying just beneath the plasma membrane like a cap over the nucleus. The lower part of the two arms is called the equatorial segment. The acrosome contains many enzymes that are exposed by the acrosome reaction, the loss of the acrosome immediately before fertilization. This reaction is one of exocytosis, the fusion of an intracellular storage vesicle with the inner surface of the cell membrane, followed by release of the vesicle contents. The acrosome reaction requires an influx of calcium ions, the efflux of hydrogen ions, an increase in pH, and fusion of the plasma membrane with the outer acrosomal membrane, leading to the exposure and escape of the enzymes contained on the inner acrosomal membrane. Binding to the zona pellucida is required to permit a component of the zona to induce the acrosomal reaction. This component is believed to be a glycoprotein sperm receptor, which thus serves dual functions: binding of sperm and induction of the acrosomal reaction.
The initial contact between the sperm and the oocyte is a receptor-mediated process. The zona pellucida is composed of glycoproteins secreted by the oocyte, known as ZP1, ZP2, ZP3, and ZP4, with ZP3 being the most abundant.76,77,78 and 79 In humans, ZP3 and ZP4 are the primary ligands for sperm and ZP2 binding occurs after the acrosome reaction, participating in the zona reaction to prevent polyspermy.80 Structural alteration of these glycoproteins leads to a loss of activity; inactivation of these ligands after fertilization is probably accomplished by one or more cortical granule enzymes. The ZP gene is expressed only in growing oocytes. DNA sequence similarities of the ZP3 gene in various mammals indicate that this gene has been evolutionarily conserved and that the sperm-ligand interaction is a common mechanism among mammals.81 Mice with a disrupted ZP3 gene produce oocytes lacking a zona pellucida and are unable to become pregnant.82,83 A vaccine against zona pellucida glycoproteins from pigs is used to control reproduction in a variety of female animals, including female elephants and deer.84 Human use of such a vaccine has been hampered by the difficulty in preparing pure glycoproteins, but this is now possible using recombinant techniques.85
![]() |
![]() |
The initial binding of the sperm to the zona requires recognition on the part of the sperm of the carbohydrate component of the species-specific glycoprotein ligand molecule.79,86 Once binding is accomplished, the acrosome reaction is triggered by the peptide chain component of the receptor glycoprotein. At least one receptor on the sperm head is a tyrosine kinase that is activated by binding to the ZP3 glycoprotein and is an initiator of the acrosome reaction.87,88 This interaction is analogous to the general principle of behavior for hormone-receptor binding and activity. In the case of sperm and oocyte, recognition of the oocyte zona ligand involves an enzyme on the surface of the sperm that becomes exposed during capacitation. Formation of the ZP3-enzyme complex, therefore, not only produces binding but also induces the acrosome reaction. The G protein signaling system is present on sperm heads, and activation at this point in time by progesterone, in an extragenomic mechanism, opens calcium channels to increase intracellular levels of calcium ions, a requirement for the acrosome reaction.89,90 and 91 Thus, the initial sperm-zona interaction depends on binding of acrosome-intact spermatozoa, followed by a process mediated by the enzymes released by the zona-induced acrosome reaction. Protein kinase C activation is an important step in the acrosomal reaction, leading to phosphorylation of sperm proteins involved in the process.92,93
Glycodelin is a glycoprotein with many isoforms, found in endometrium, the fallopian tubes, follicular fluid, and in seminal fluid. The various forms of glycodelin modulate sperm function and fertilization by maintaining sperm in the uncapacitated state and inhibiting binding of sperm to the zona pellucida by competing for zona receptors. A specific receptor for glycodelin is present on sperm, and thus it makes sense that down-regulation of glycodelin expression would be associated with the hormonal changes at ovulation coinciding with the opening of the fertilization window.94
Spermatozoa enter the perivitelline space at an angle. The oocyte is a spherical cell covered with microvilli. The sperm head is like a flat dish, and the thickness of the head is a little less than the distance between the oocyte microvilli.95 The region of the equatorial segment of the sperm head, the distal portion of the acrosome, makes initial contact with the vitelline membrane (the egg plasma membrane or oolemma). At first, the egg membrane engulfs the sperm head, and, subsequently, there is fusion of egg and sperm membranes. Bedford has opined that the tangential trajectory of this process allows closure with expansion of the blastocyst, preventing the persistence of a hole that would allow herniation or interfere with the normal hatching that occurs later within the uterus.96
Sperm-egg fusion is mediated by specific proteins. Two membrane proteins from the sperm head have been sequenced; one (PH-20, also called SPAM1) is involved in binding to the zona pellucida, and the other (PH-30, also called fertilin) is involved in fusion with the oocyte.97,98 PH-20, with hyaluronidase activity, is also active in dispelling the cumulus.99 The cell membrane of the unfertilized oocyte contains integrin adhesion/fusion molecules that recognize peptides such as fibronectin, laminin, and collagen.100 Fibronectin appears on the spermatozoa, but it is disputed whether it appears with caudal maturation or after capacitation. Vitronectin is a sperm protein that is activated after capacitation and the acrosome reaction and may be the key peptide interacting with oocyte cell membrane integrins.101 These steps in the fusion process will occur only with sperm that have undergone the acrosome reaction. Multiple sperm-associated surface proteins are involved in binding to the oocyte membrane, but no single peptide has been identified as absolutely essential for fertilization, implying redundancy.102,103
Fusion of the sperm and oocyte membrane to form a zygote is followed by the cortical reaction and metabolic activation of the oocyte. An increase in intracellular free calcium in a periodic, oscillatory pattern always precedes the cortical reaction and oocyte activation at fertilization, and this is believed to be the mechanism by which the spermatozoon triggers these developmental events.62,104,105 It is believed that calcium signaling in fertilization is initiated by the introduction of a sperm factor into the egg, a phospholipase protein that activates inositol 1,4,5-trisphosphate leading to calcium release.105 An analysis of failed fertilizations in one couple after intracytoplasmic sperm injections (ICSI) indicated a high prevalence of failed oocyte activation; repeated ICSI after initiating oocyte activation with a calcium ionophore resulted in a successful pregnancy.106
The initiation of the block to penetration of the zona by other sperm is mediated by the cortical reaction, another example of exocytosis with the release of materials from the cortical granules, lysosome-like organelles that are found just below the egg surface.107 As with other lysosome-like organelles, these materials include various hydrolytic enzymes. Changes brought about by these enzymes lead to the zona reaction, the hardening of the extracellular layer by cross-linking of structural proteins, and inactivation of ligands for sperm receptors.108 Thus, the zona block to polyspermy is accomplished. The initial change in this zona block is a rapid depolarization of the oocyte membrane associated with a release of calcium ions from calmodulin.109,110 The increase in intracellular calcium acts as a signal or trigger to activate protein synthesis in the oocyte. The depolarization of the membrane initiates only a transient block to sperm entry. The permanent block is a consequence of the cortical reaction and the release of enzymes, also apparently triggered by the increase in calcium.
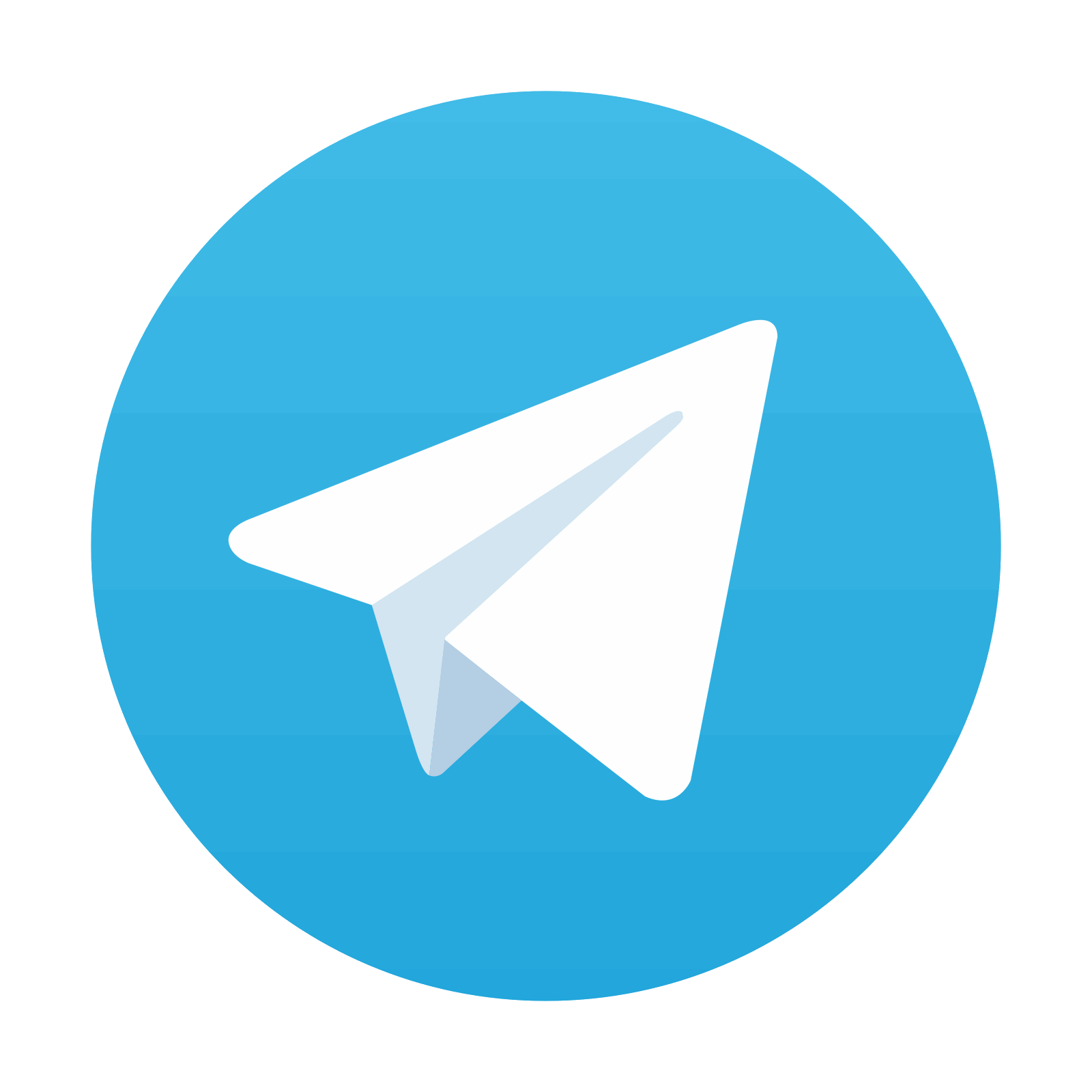
Stay updated, free articles. Join our Telegram channel

Full access? Get Clinical Tree
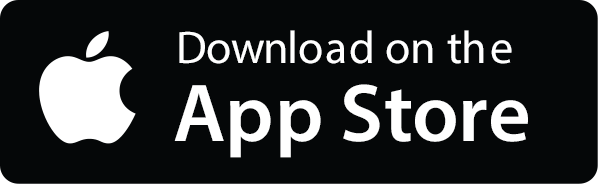
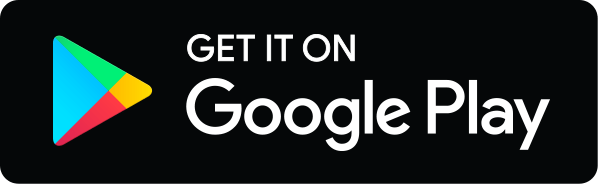