Parenteral Nutrition
TOTAL PARENTERAL NUTRITION
Total parenteral nutrition (TPN) as used today in the neonatal intensive care unit (NICU) became a part of modern medicine in the late 1960s. Dudrick et al, in groundbreaking work, first demonstrated in beagle puppies the technique for parenterally administering nutrients.1 Shortly thereafter, Wilmore and Dudrick2 reported the use of this technique in an infant who had virtually no remaining small intestine and was totally dependent on these parenterally delivered nutrients of glucose, fibrin hydrolysate, minerals, and vitamins. There were no intravenous fat emulsions available, so plasma was given in small aliquots to provide some essential fatty acids (EFAs) and trace elements.2 Although this historic patient did not survive, normal growth and development was maintained for several months solely on this primitive solution. By the early 1970s, the technique was being used extensively in infants and children with congenital or acquired surgically correctable lesions of the gastrointestinal tract3,4 and in infants with intractable diarrhea.4,5 Use in the low birth weight infant soon followed.4,6,7
Today, TPN is a firmly established strategy in modern neonatal intensive care. The extremely low birth weight (ELBW) infant (<1000 g birth weight) with limited endogenous stores of nutrients and an increased rate of resting energy expenditure is at risk for rapid development of malnutrition or even actual starvation.8 The pioneering work of Dudrick and Wilmore has led to the current practice of early amino acids as the first infusate within hours of life in a 500-g infant. This chapter focuses on the use of TPN in very low birth weight (VLBW) infants (<1500 g birth weight).
The ELBW infants have unique nutritional requirements characterized by high metabolic rate, high protein turnover, and high glucose utilization. The ELBW infant has endogenous energy reserves of about 200 kcal, only enough energy to last days without exogenous sources. The 23-week infant has reserves that will be exhausted within hours. These infants are extremely vulnerable to inadequate nutrition.
Nutrient intakes provided to ELBW infants parenterally are much lower than what the fetus receives in utero. This intake deficit may persist throughout the infants’ stay in the NICU and even after discharge.9 These suboptimal nutrient intakes are critical in explaining the poor growth these infants often experience. The early growth deficit has long-lasting effects, including short stature and poor neurodevelopmental outcomes.10,11 Therefore, the observation of postnatal growth failure is a “surrogate” for inadequate nutrition and the likelihood of adverse neurodevelopmental outcome. Early aggressive TPN theoretically allows the transition from fetal to extrauterine life to occur with minimal interruption of growth and development and has been a successful strategy to improve growth and prevent inadequate nutrition.12,13
EARLY AGGRESSIVE AMINO ACID ADMINISTRATION
Immaturity of the gastrointestinal tract and critical illness in ELBW infants precludes substantive nutritional support from enteral nutrition the first days or even weeks of life; thus, nearly all of these infants are supported parenterally. Large amounts of amino acids are available to the fetus in utero, which are utilized not only for protein synthesis but also as a fuel source. It seems logical to supply a neonate with adequate amounts of amino acids for energy, as well as a growth substrate, to promote protein accretion for ongoing development.
Understanding fetal nutrition has been helpful in designing postnatal strategies for ELBW infants. At 70% of gestation, there is little fat uptake. Fetal energy metabolism is not dependent on fat until early in the third trimester, and then it increases only gradually toward term. Glucose is delivered to the fetus from the mother at low fetal insulin concentrations, generally at a rate that matches fetal energy expenditure. The human placenta actively transports amino acids into the fetus, and animal studies indicate that fetal amino acid uptake greatly exceeds protein accretion requirements. Approximately 50% of the amino acids taken up by the fetus are oxidized and serve as a significant energy source. Urea production is a by-product of amino acid oxidation. Relatively high rates of fetal urea production are seen in human and animal fetuses compared with the term neonate and adult, suggesting high protein turnover and oxidation rates in the fetus. Therefore, a rise in BUN (serum urea nitrogen), which is often observed after the start of TPN, is not in and of itself an adverse effect or sign of toxicity. Rather, an increase in urea nitrogen is a normal accompaniment of metabolizing amino acids.
Providing early parenteral amino acids prevents metabolic shock. Concentrations of key amino acids begin to decline in the VLBW infant at the time the cord is cut. This metabolic shock may trigger the starvation response, of which endogenous glucose production is a prominent feature. Irrepressible, glucose production may be a cause of so-called glucose intolerance that often limits the amount of energy that can be administered to the ELBW infant. It makes sense to ease the metabolic transition from fetal to extrauterine life. Withholding TPN for days, or even hours, sends the infant unnecessarily into a metabolic emergency. Thus, the need for TPN may never be more acute than right after birth.
It is noteworthy that 3 separate studies measuring glucose tolerance, including one that measured insulin activity, confirmed that glucose tolerance was improved in the infants receiving early, higher-dose amino acids.13–15 Therefore, early amino acids may stimulate insulin secretion. This is consistent with the notion that forestalling the starvation response improves glucose tolerance. Clinically, this improved glucose tolerance safely allows the provision of more nonprotein energy while avoiding hyperglycemia.
This relationship between early amino acids and insulin secretion is shown on Figure 10.1. This metabolic benefit prevents 2 morbidities—hyperglycemia and nonoliguric hyperkalemia—that make the provision of nutrition difficult. The mechanism may be that, without initiation of early parenteral amino acids, plasma concentrations of certain amino acids (eg, arginine and leucine) decrease. Secretion of insulin depends on the plasma concentrations of these amino acids as well as that of glucose. A shortage of amino acids limits the secretion and activity of insulin. Finally, glucose transport and energy metabolism are adversely affected by a reduction in insulin and insulin-like growth factors. This scenario leads to a downregulation of glucose transporters at the cellular level, resulting in intracellular energy failure via a decrease in Na+, K+-ATPase (sodium-potassium adenosine triphosphatase) activity. This directly contributes to leakage of intracellular potassium and is associated with nonoliguric hyperkalemia. Early TPN with amino acids minimizes the abrupt postnatal deprivation of amino acid supply and provides the following benefits:
• Prevention of protein catabolism
• Maintenance of growth-regulating factors such as insulin
• Prevention of hyperglycemia and nonoliguric hyperkalemia
FIGURE 10-1 Early administration of amino acids. TPN, total parenteral nutrition. (From Adamkin DH. Nutritional Strategies for the Very Low Birth Weight Infant. Cambridge, UK: Cambridge University Press; 2009.)
Figure 10.2 shows protein loss that occurs in infants that are mechanically ventilated at 26 week of gestation and approximately 900 g birth weight at 2 days of age who were receiving glucose alone.16 Also shown are clinically stable 32-week gestation premature infants and normal term infants for comparison. It is clear that there is a significant effect of gestation on protein metabolism as the rate of protein loss in ELBW infants is 2-fold higher than in normal term infants.16
FIGURE 10-2 Protein losses measured in three groups of infants receiving glucose alone at 2–3 days of age. Protein losses are calculated from measured rates of phenylalanine catabolism. (From Denne SC. Protein and energy requirements in preterm infants. Semin Neonatol. 2001;6(5):377–382.)
The impact of this rate of protein loss is shown in Figure 10.3. At 26 weeks of gestation, a 1000-g birth weight infant begins with body stores of approximately 88 g. Without exogenous provision, the infant loses over 1.5% of body protein per day.16 Contrast this with the normal fetus, who would be accumulating body protein at over 2% per day. Obviously, significant body protein deficits can accumulate rapidly in ELBW infants not receiving early amino acid administration.
FIGURE 10-3 Change in body protein stores in a theoretical 26-weeks gestation, 1000-g premature infant receiving glucose alone as compared with a fetus in utero. (From Denne SC. Protein and energy requirements in preterm infants. Semin Neonatol. 2001;6(5):377–382.)
The first studies of early TPN used doses between 1.0 and 1.5 g/kg/d, an amount that will replace ongoing losses. More recent studies support dosages of 3.0 g/kg/d initiated within hours of birth. Ultimately, amino acid intake should be 3.0 g/kg/d; however, one can consider intakes of 3.5 to 4.0 g/kg/d for infants weighing less 1200 g if enteral feeds are withheld for prolonged periods. Growth benefits associated with parenteral amino acid infusions should be associated with less-extreme postnatal weight loss and an earlier return to birth weight. An earlier return to birth weight means the VLBW infant will be less likely to develop extrauterine growth restriction.
We recently published an 8-year experience developing an early amino acid strategy paying particular attention to clinical and metabolic responses.13 Table 10.1 shows the benefits of early amino acids, which include a decrease in postnatal weight loss and age at return to birth weight, especially with earlier initiation of amino acids within hours of birth and amino acid dosage over the first 5 days of life at 3.0 g/kg/d.13 Prevention of extrauterine growth retardation (EUGR) by weight was seen with higher dose and earlier initiation.13 We also confirmed that there is no demonstrable relationship between the preceding day’s amino acid intake and BUN.13 This is because BUN is related not only to amino acid intake but also renal function, hydration, acuity of illness, as well as degree of prematurity.13 Therefore, in most situations a modest rise in BUN (a major earlier concern) is consistent with the utilization of amino acids as a source of energy.17
Table 10-1 Outcome data (mean ± SD)
Another nutritional benefit in our study showed early intravenous amino acids not only improved energy intakes but also at the same time allowed for more conservative fluid management, which may benefit ELBW infants in prevention of chronic lung disease.13
In assessing potential toxicity with amino acid intakes of 3.0–4.0 g/kg/d shortly after birth, a study comparing 1 g/kg/d vs 4 g/kg/d showed no significant differences between groups in the amount of sodium bicarbonate administered, the degree of metabolic acidosis, or the BUN concentration.15 When compared with plasma amino acid concentrations of normally growing second- and third-trimester human fetuses (sampled by cordocentesis), fetal amino acid concentrations for both essential and nonessential amino acids matched well to those in the group receiving 3.0 g/kg/d (except for threonine and lysine, which were significantly lower than seen in fetuses). Concentrations in the group at 1 g/kg/d were about 50% lower compared to fetal levels. Efficacy was significantly lower in the 1-g/kg/d group by nitrogen balance determined using leucine stable isotope methods.15
Whether plasma concentrations of amino acids of the fetus should be duplicated in the VLBW infants is unknown. Crystalline amino acid solutions designed for infants promote nitrogen balance and yield “safer” aminograms than the adult mixtures used before the pediatric solutions became available.18 However, these solutions do not provide sufficient threonine and lysine, both essential amino acids that may at least theoretically increase nitrogen and protein balance.19 Also, cysteine and tyrosine, considered indispensable amino acids for the infant, are not included in the mixtures20 because both are insoluble. VLBW infants have been known to have lower plasma concentrations of cysteine and tyrosine. Greater intakes of the precursors of those 2 amino acids, methionine and phenylalanine, respectively, do not necessarily result in greater plasma levels of the desired amino acids, although plasma levels of the 2 precursors do increase.21 Cysteine hydrochloride can be provided as a supplement to TPN; however, trials of cysteine addition have not shown consistent clinical benefits.22 Pediatric amino acid solutions may contain soluble N-acetyl-L-tyrosine, but its efficacy for enhancing tyrosine metabolism is questionable as much of the dipeptide appears to be excreted into the urine.20 Therefore, both tyrosine and cysteine remain problematic.
Like tyrosine, taurine, and cysteine, glutamine may also be a conditionally essential amino acid in preterm infants.23
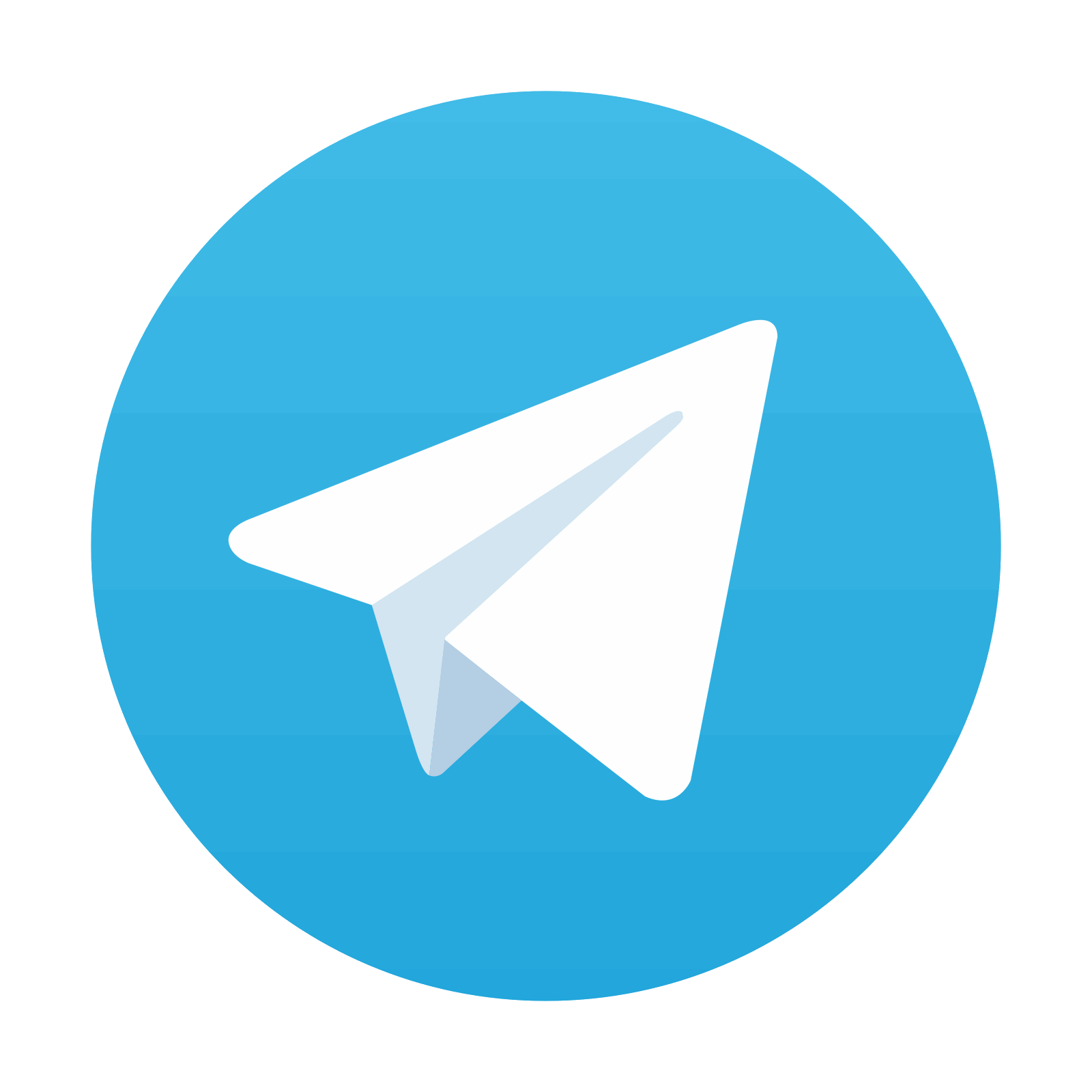
Stay updated, free articles. Join our Telegram channel

Full access? Get Clinical Tree
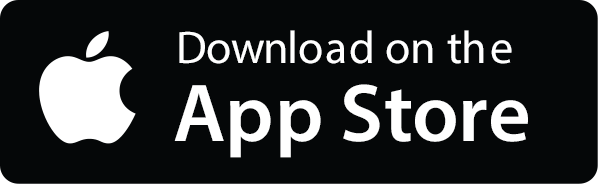
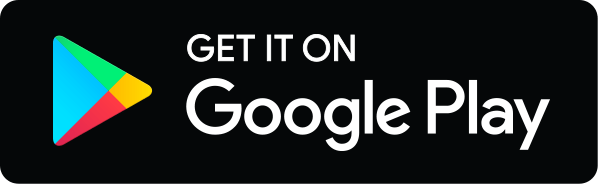