Oncogenesis
Joel Shilyansky
Department of Pediatric Surgery, Medical College of Wisconsin, Children’s Hospital of Wisconsin, Milwaukee, Wisconsin 53226.
Oncogenesis or development of cancer is a progressive stepwise cellular process that leads to pathogenic conditions. The two fundamental processes required for oncogenesis include transformation and immortalization. Cellular proliferation is normally regulated by growth factors and inhibited by growth arrest signals. However, transformed cells can proliferate in the absence of growth factors and in the presence of signals for growth arrest. With progression, cancer cells are able to exploit normal cellular processes, acquire characteristics that enhance their survival, proliferation, and spread. Moreover, cancer cells can often grow despite stressful conditions such as deprivation of nutrients or oxygen, crowding, altered interaction with stromal cells and extracellular matrix (ECM), and in the absence of growth hormones. Cancer cells are also able to invade adjacent tissues or escape into the lymphatics and the circulation. Critically, cancer cells can exit the circulation and establish new deposits or metastases. They avoid host defenses by exploiting mechanisms established to induce tolerance to self. Finally, cancer cells can induce vascular ingrowth and disturb host metabolism.
The behavior of cancer cells is dictated by the pattern of gene expression and by the interaction with the surrounding cells and ECM. The changes in the pattern of gene expression and the function of gene products may be caused by alteration in the genetic message resulting from mutations, gene amplification, chromosomal translocations or deletions, and from alterations in the regulation of gene expression. These genetic changes lead to enhanced expression of oncogenes to promote the malignant phenotype, and the reduced expression of tumor suppressor genes that normally suppress the malignant phenotype. An important concept is that, although each genetic change occurs randomly, oncogenesis is an organized multistep process. Thus, the genetic events accumulating in cancer cells are not random, but involve specific cellular processes or pathways. Altering the regulation of these pathways leads to oncogenesis.
GENE EXPRESSION IN CANCER
Multiple cellular mechanisms for altered gene expression and cellular responses have been described:
Inappropriate expression of a gene product is usually the result of alterations in the regulation of transcription of genetic messages. Such alterations may result from chromosomal translocations in which a portion of a chromosome is separated from its native location and transferred to a new site. In some cases, the regulatory sequences controlling gene transcription may be moved during the translocation process to enhance transcription of oncogenic sequences. A gene can also alter its expression in response to the insertion of DNA from a virus that disturbs the native regulatory mechanisms or introduces viral promoters and enhancers of transcription.
Altered gene copy number results from gene amplification or chromosomal duplications and leads to quantitative changes in gene transcription. In concert, accessibility of the genes to the transcriptional machinery is frequently affected. The number of gene copies and the accessibility of the genes determines the amount of gene product or protein produced in a cell.
Altered protein production is a function of the amount of message transcribed, stability of the mRNA, and the efficiency of translation and stability of the protein. The stability of a genetic messages and efficiency of translation are determined by the gene sequence and by specialized sequences in the untranslated regions of the mRNA. Protein stability is affected by the amino acid sequence and by posttranslational modifications. In addition, translational machinery has been increasingly recognized as playing a central role in regulating protein production.
Alteration in gene function. Point mutations and deletions within the coding sequence of the gene can change its catalytic or regulatory activities. Chromosomal translocations can change gene function by removing functional domains or inserting new domains from other loci to create hybrid proteins with novel activities.
Cancer-specific genes may be classified into three general groups based on their role in oncogenesis:
Oncogenes are genes whose products promote carcinogenenesis. These genes usually code for proteins that promote cell growth, proliferation, survival, motility, spread, angiogenesis, alteration in stromal functions and ECM composition. During carcinogenesis, genes with oncogenic potential escape normal regulatory mechanism.
Tumor suppressor genes (TSGs) are genes whose products normally regulate cell proliferation, division, migration, and utilization of metabolites. Death genes, which code for proteins that mediate cell death or apoptosis, function as TSGs. Loss of both copies of TSGs, either through physical loss or by mutations leading to loss of genetic function, is usually required for tumor progression.
Cellular clocks like telomerase are gene products that play a role in determining the extent to which cells may continue to divide. More recently, greater understanding of the mechanisms involved in maintaining the integrity of genome has led to the recognition that mutations in genes coding for DNA repair and replication machinery can lead to genomic instability. If the machinery that recognizes loss of DNA integrity and induces apoptosis is also defective, cells can accumulate mutations and acquire a malignant phenotype.
MULTISTEP CARCINOGENESIS
Oncogenesis is a multistep process characterized by the accumulation of multiple genetic alterations. The prototype of multistep carcinogenesis is the evolution of colorectal cancer; investigators have dissected the histopathologic stages of colonic mucosal epithelial transformation and molecularly characterized each stage in the process. This refined analysis suggests that transformation can commence with an increased proliferation within the crypts of the colon of cells carrying a mutated ras oncogene. A histopathologic progression schema can be drawn from hyperproliferation through early, intermediate, and late adenoma to frank carcinoma and metastatic cancer. Mutations of genes on chromosome 5q (APC), 18q (DCC), and 17p (p53), as well as additional chromosomal aberrations and changes in chromatin methylation patterns, which alter the availability of genes to transcriptional machinery, are shown to accumulate corresponding to the progression to frank malignancy.
The notion of multistep carcinogenesis has not been as clearly applied to pediatric malignancies. However, the basic principles are still applicable, especially in the context of more recent reports emphasizing the importance of the cellular context on tumorigenesis. The causes of pediatric malignancies are complex and multifactorial, but certain features of these cancers are distinctive. Several pediatric malignancies occur when the tissue of origin is in a pronounced growth phase, possibly promoting rapid growth or inhibiting the elimination of transformed cells. The presence of “activated” ECM and stroma may promote the growth or remove the restraints on some premalignant cells. One example of a tumor arising during a growth phase is osteosarcoma, which is commonly found in long bones of preteens or teenagers. These notions suggest that it may be possible that pediatric malignancies would become quiescent if these activated ECM signals could be pharmacologically controlled.
GENETIC INSTABILIITY AND DNA REPAIR
The minimal requirement for oncogenesis is cellular immortality and transformation or alteration in cellular response to the local environment favoring proliferation. In addition malignant cells can acquire the ability to invade and spread autonomously. The process of oncogenesis is driven by alterations in the genetic makeup of the cells that permit deviation from the programmed cellular responses. Chromosomal translocations, duplications, deletions, and inversions, as well as gene amplification and point mutations, are universally found in cancer cells.
Mutations
Fundamental to genetic inheritance is genetic stability over many generations of cells. However, cells have to contend with intracellular and extracellular insults to the chemical stability of the DNA. Sources of mutations include infidelity of DNA replication, inappropriate incorporation of uracil, oxidation and deamination of nucleotides, spontaneous hydrolysis resulting in depurination or depyrimidination, and chemical alteration in bases by reactive oxygen radicals (1). Conservative estimates suggest that spontaneous damage to DNA accounts for greater than 10,000 mutations per cell each day (2). In addition, exogenous genotoxins such as ionizing and ultraviolet irradiation, cigarette smoke, and chemotherapeutic agents can compromise the integrity of DNA. DNA damage can be characterized as predominantly cytotoxic, leading to
apoptosis or senescence, or predominantly mutagenic, leading to alteration in the sequence of the DNA molecule and potentially oncogenesis.
apoptosis or senescence, or predominantly mutagenic, leading to alteration in the sequence of the DNA molecule and potentially oncogenesis.
The presence of DNA damage triggers the activation of several cellular signaling cascades. Cell cycle progression is usually arrested in response to DNA damage, providing additional time for DNA repair and preventing the propagation of altered genes. If the DNA damage is beyond the capacity of a cell to repair, then there is normally activation of proapoptotic pathways leading to cell death. However, small areas of DNA damage may be tolerated to permit replication and cell survival. Such alterations are mutagenic and, if not repaired, may lead to oncogenesis. DNA damage activates DNA repair machinery, which falls into three classes, nucleotide excision repair (NER), base excision repair, and mismatch repair (MMR) (2). Failure to repair DNA can lead to the development of cancer. For example, defects in NER and MMR have been shown to lead to colon, uterine, ovarian, and gastric cancers (1,3,4).
Chromosomal Abnormalities
Most cancer cells manifest a large number of chromosomal abnormalities, such as translocations, deletions, duplications, and inversions. It is clear now that chromosomal rearrangements lead to oncogenesis by altering gene expression and function (5). For example, it is known that the gene product of the bcr-abl genetic translocation codes for a tyrosine kinase is required for the development of chronic myelogenous leukemia (CML) and many cases of acute lymphocytic leukemia (6,7). In 1998, a kinase inhibitor STI-571 was used to target bcr-abl and successfully treat CML patients, proving the causality of this t(9;22) translocation in oncogenesis (8,9,10,11,12).
The cloning of translocation points permits the identification of genes involved in oncogenesis. Molecular approaches then permit the identification and classification of cancers with increased accuracy, leading to better prognostic information and permitting effective therapeutic intervention. Even more exciting is the possibility of targeting the gene products resulting from chromosomal translocation, using novel tumor-specific therapies such as the case for bcr-abl (9,10).
Molecular Mechanisms Leading to Genetic Instability
Although the role of chromosomal rearrangements in oncogenesis has been recognized, the causes of genetic instability are not fully understood. Chromosomal rearrangement requires DNA double-strand breaks (DSBs), which may be induced by extrinsic insults such carcinogens and ionizing irradiation or by intrinsic cellular processes (13,14). DSBs are normally repaired by homologous recombination, where the homologous intact DNA strand is used as a template for accurate repair, or by nonhomologous end joining, a mechanism that joins double-stranded ends irregardless of sequence (13,14). When DSB repair mechanisms fail, hypersensitivity to DNA damage, chromosomal instability, and gene amplification result. In most cells, the presence of DSBs triggers the activation of checkpoint control pathways leading to cell cycle arrest and apoptosis (15,16). If checkpoint control pathways are inactivated, for example, due to mutations in the p53 gene, DSBs can lead to tumor formation or progression (15,17,18,19,20).
Genetic amplification has been best described in neuroblastoma, where MYCN may be amplified 700-fold, and can appear cytogenetically as homogeneously staining regions, or small lengths of extrachromosomal genetic material called double minutes. Gene amplification in neuroblastomas is generally linked to tumor progression, spread, and poor clinical outcome (21). The underlying molecular mechanisms leading to gene amplification are poorly understood, but seem to be associated with defective repair of DSBs and possibly telomere erosion (17,22,23,24).
CELLULAR PATHWAYS LEADING TO ONCOGENESIS
Cell Cycle and Proliferation
One of the basic characteristics of tumor cells is increased rate of proliferation compared with surrounding cells. There are four basic stages to cell division: mitosis (M phase), where two identical copies of the DNA are distributed equally into two separate daughter cells; G1, a gap phase where cells prepare for DNA replication; S, phase where DNA replication occurs; and G2, a gap phase where cells prepare for mitosis (25,26). Cells in G1 or G2 may enter into a quiescent phase G0, where cell division does not occur. To ensure proper progression through the cell cycle, cells have developed a series of checkpoints that ensure the previous phase has been successfully completed. Cells also require the appropriate set of exogenous and endogenous signals to enter a new cycle (25,27). Such signals ensure cells are able to perform their functional role and control the number and location of cells in a multicellular organism. If there are inherited or acquired abnormalities in this cell cyle regulatory machinery, cancer cell proliferation becomes autonomous of external signals and may proceed despite endogenous signals for growth arrest.
Understanding how tumor cells subvert cell cycle regulation may lead to improved therapies against cancer. Such strategies are already under intense study. Surprisingly, despite targeting pathways that seemingly affect all cells, such approaches appear to demonstrate significant selectivity for particular tumors.
Tyrosine Kinases
Tyrosine kinases (TKs) are an important group of signaling molecules that regulate cellular processes. These molecules can be divided into two groups. Receptor tyrosine kinases have an extracellular domain and are activated in response to external signals, whereas cytoplasmic tyrosine kinases respond only to endogenous intracellular signals. Activated TKs phosphorylate cellular proteins, often other kinases, initiating a signaling cascade. The interaction of multiple pathways, possibly triggered by circulating hormones, growth factors, engagement of adhesion molecules, or metabolites in the cellular environment, determines whether a signal is amplified or extinguished. More than 100 kinases have been identified and more than 30 are altered in cancer cells. Constitutive activation of oncogenic TKs (OTKs), such as src, frees cancer cells from dependence on external stimuli, such as adherence to extracellular matrix, in order to proliferate and survive (28,29). The oncogenic functions of many OTKs have been described. More recent evidence suggests that OTKs also protect tumor cells from cytotoxic therapies that target the DNA (16,29,30,31). This dependence on OTKs for tumor cell resistance to cytotoxic agents may be exploited (32). For example, treating patients with OTK inhibitors such as Imatinib mesylate, which targets bcr-abl, may open a window of opportunity during which tumor cells may be susceptible to chemotherapeutic agents, radiation therapy, or immunotherapy (9,10,12,29,31,33,34). Undoubtedly, other more specific OTK inhibitors will be developed for the management of other pediatric tumors.
Telomere Maintenance
Telomeres are the structures located at the ends of eukariotic chromosomes and normally shorten with each cell division. Telomeric DNA, containing tandem arrays of a GT-rich nucleotide sequence- 5′TTAGG3′ and telomere-specific binding proteins, is essential to stabilizing chromosome ends by forming a cap structure that protects them from terminal fusion (35). Telomere length may be considered a cellular clock and once the telomeres are degraded, cells enter senescence. Thus, prevention of telomere shortening is critical to oncogenesis (36,37). Cancer cells apparently have unlimited capacity to proliferate by maintaining telomere length using an RNA-dependent, DNA polymerase, termed telomerase (38). Inhibition of telomere maintenance leads to apoptosis of tumor cells in vitro, offering a potential opportunity for therapeutic intervention (39,40).
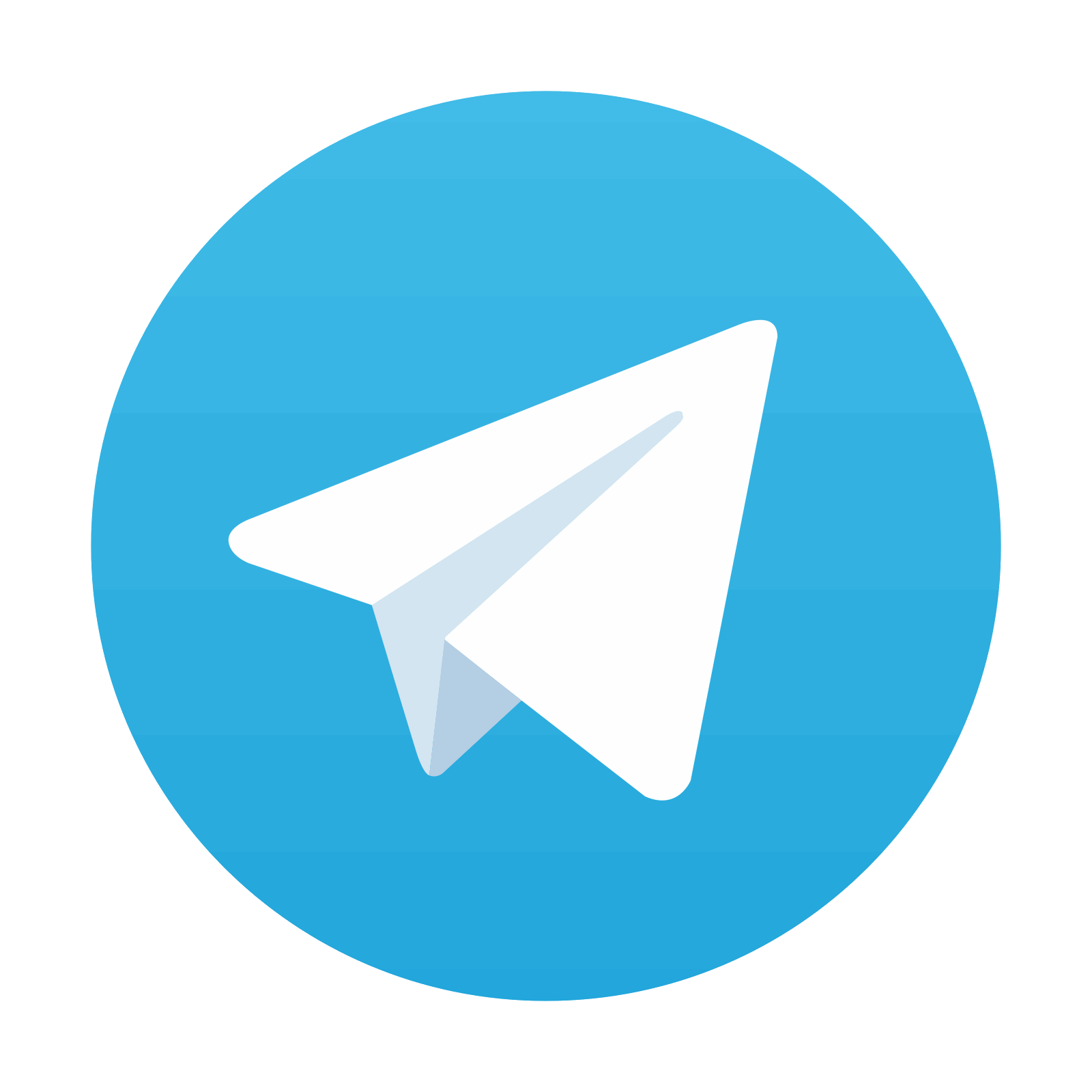
Stay updated, free articles. Join our Telegram channel

Full access? Get Clinical Tree
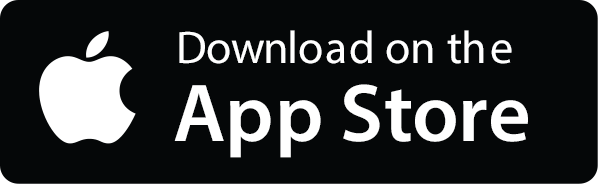
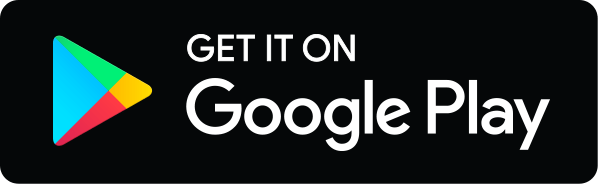