Neurosurgery of the Newborn
Kristian Aquilina
Treatment of structural and functional abnormalities of the neonatal central nervous system (CNS) provides unique challenges to both the neurosurgeon and neonatologist caring for the infant. Considerations of several interrelated pathophysiologic processes can provide an understanding of the nature of the abnormality and goal of treatment. This understanding forms the basis for a successful interdisciplinary team approach to the management of these infants.
Almost all neurosurgical interventions in the neonate can be discussed in terms of four categories of technical intervention: (a) drainage or diversion of fluids, (b) closure of openings (including neural tube defects), (c) removal of tissue (including neoplasms and anomalous masses), and (d) opening of fusions (such as craniosynostoses). In the management of many newborn patients, two or more of these inventions may be necessary. Some neonatal nervous system problems, such as vascular disorders, do not yet have a workable neurosurgical intervention.
When applying these interventions to neonatal care, their use must be guided by pathophysiologic mechanisms unique to the neonate, such as (a) the biomechanics of neonatal brain tissue and a distensible skull, (b) recognized and unrecognized congenital anomalies (microscopic and macroscopic), and (c) the plasticity of neonatal CNS tissue and its effects on response to injury. Recent advances in antenatal diagnosis allow unprecedented opportunity to manage these processes even before birth. Frequently, solutions to a neonatal neurosurgical problem must address combinations, or complex interactions, of these pathophysiologic mechanisms.
The biomechanical nature of neonatal brain tissue and a distensible skull accounts for one of the best-known cardinal signs of neurosurgical difficulty in the neonate, namely abnormal head growth. The prodigious ability of the skull to grow and sutures to widen can allow some of the most severe cases of hydrocephalus to result in relatively mild pressure effects, even when the need for cerebrospinal fluid (CSF) shunting is clear. These same considerations in the type and nature of response of the tissues to abnormal fluid buildup can result in dramatic craniocephalic disproportion once the pressure is relieved. The change in brain and skull biomechanics over time adds particular challenge to management of these problems.
Obvious congenital anomalies, especially those involving open neural tube defects and exposed CNS tissue, may require urgent neurosurgical intervention. A complicating aspect is the potential occurrence of concurrent microscopic abnormalities, such as widespread synaptic miswiring. In many cases, neurologic deficits may be present in a patient with macroscopic structural abnormalities, but major neurologic disability may come from less obvious problems with tissue development, currently not treatable by neurosurgical means. Diffusion tractography has identified multiple white matter abnormalities in association with open myelomeningocele and Chiari II (1).
The details of the plasticity and vulnerability of the neonatal CNS is discussed in Chapter 46. It is well known that the ability to recover function after CNS injury is age dependent and seems to be better in younger individuals. Interaction between the agedependent variables of CNS plasticity, recoverability, and vulnerability makes the task of estimating prognosis after an injury or neurosurgical intervention extremely difficult.
Antenatal diagnosis has changed many aspects of neurosurgical conditions. Obstetric management may be altered, for example, site and mode of delivery, in the setting of a known neural tube defect. Antenatal surgery for open myelomeningocele has been investigated in a randomized controlled trial (2). In addition, the psychological issues around the time of neurosurgical therapy for the newborn are altered, as the child’s family now has the opportunity to meet the neurosurgeon prenatally and fully discuss and decide upon any contemplated care plan before birth.
Disorders of Cerebrospinal Fluid Accumulation
The most common neurosurgical consultation for newborn patients is to evaluate and treat enlargement of the ventricular system. The clinical signs of progressive enlargement of the ventricular system include excessive increase in the head circumference (HC), fullness in the anterior fontanelle (especially when the patient is upright when the venous and fontanelle pressures should be low), episodic apnea and bradycardia, general lethargy, and abnormalities of ocular movement, especially restricted upgaze. Many of these findings are nonspecific and can be seen with hydrocephalus of any etiology. At a practical level, the most important distinction is between progressive and static abnormalities in ventricular volume. This is relatively easy to determine using serial cranial ultrasound examinations (Fig. 47.1). Resistive index (RI) measurements add a physiologic dimension to the anatomic images gathered by ultrasonography (3). In our experience, the presence of dramatic changes in the Dopplermeasured flow signals in the anterior cerebral artery with gentle, brief compression of the anterior fontanelle correlates extremely well with the intracranial pressure by measurement and the probability of eventual need for a ventriculoperitoneal (VP) shunt (3).
The vast majority of hydrocephalus cases seen in neonates are associated with, and traditionally thought to result from, abnormal resorption of CSF. After intraventricular hemorrhage (IVH) (Fig. 47.1), traditional thinking is that partial occlusion of the normal resorptive pathways of CSF through the arachnoid villi, as well as scarring in the basal cisterns, leads to a communicating hydrocephalus (4). The neonatal sagittal sinus does not contain arachnoid granulations, and minor pathways of CSF absorption are particularly important (5). Elegant studies in small and large animals, including nonhuman primates, have demonstrated bulk flow from the intraventricular CSF to the subfrontal subarachnoid space and on to the cribriform plate, lymphatics in the nasal mucosa, and subsequently large lymphatic channels in the neck (6). Other causes of hydrocephalus, including those associated with neural tube defects or CSF secreting tumors, are rare (Fig. 47.2). Placement of a shunt in borderline cases may result in atrophy of physiologic resorption systems and cause permanent dependence on the shunt for CSF absorption. The subarachnoid space does not develop to the same extent as in nonshunted neonates, and there is a tendency to microcephaly. Chronic shunt overdrainage in an infant may cause slit ventricle syndrome, where the ventricles are small and brain compliance is reduced. Recurrent obstruction of the ventricular catheter, due to coaptation of the ependymal surfaces on the catheter, is, in the presence of poor compliance, acutely symptomatic (7). These children have frequent shunt revisions, emphasizing the importance of selecting a valve that reduces overdrainage (8). This may involve a flow control, rather than a pressure control, valve, or consideration to including an antisiphon device in a pressure-controlled system.
It is difficult to determine whether a shunt continues to be required in a growing child. Absence of shunt flow in an asymptomatic child is difficult to establish, although recent evaluation of a device able to detect temperature difference of the skin along the distal shunt tubing has been promising (9). An ice cube is placed on the skin along the palpable tubing and a temperature drop distally along the tube is a good indicator of CSF flow (9). An alternative involves exteriorization of the distal catheter, with gradual elevation of the drainage chamber and occlusion over 2 to 3 days. If this is tolerated, it is possible that the child is shunt independent, although flow through an established subcutaneous tract still cannot be excluded. One of the problems with assessing the growth of ventricular size in the neonate is the possibility that the enlargement of the ventricles may at least partially reflect loss of tissue in the brain, rather than increase in pressure. This can become a difficult problem, as prolonged hydrocephalus itself certainly can cause loss of tissue bulk (10), and the value of shunt placement may be more difficult to determine when the tissue loss is not obviously the result of high pressure. The specific loss of myelin or white matter, called periventricular leukomalacia, is discussed in Chapter 46. The relationship between periventricular leukomalacia and hydrocephalus is of particular concern in neurosurgical decision making. When a preterm infant, especially one who has sustained an intracranial hemorrhage, shows lateral ventricular enlargement, it is difficult to determine to what extent this is a result of impaired CSF flow or absorption versus diffuse white matter damage or inadequate density of axons. In general, the absence of macrocephaly is an indicator of the atrophy. An MRI scan showing transependymal CSF flow on T2-weighted images, effacement of the cortical sulci, and inferior displacement of the third ventricular floor are indicators of hydrocephalus. Regular measurement of occipitofrontal circumference (OFC) with reference to established centile charts is crucial (11). In general, an OFC growth of less than 1 cm/wk is acceptable, whereas growth of more than 1.5 cm/wk is considered excessive. Head growth must be interpreted in the context of overall somatic growth measured by weight and length. Babies can have preferential brain catch-up growth, but it is suspicious if the rate of OFC growth is plotted at the high end of normal while the weight and length remain relatively unchanged. Because major intervention decisions are based on the intrinsically imprecise measurement of OFC, any effort to improve its precision is worthwhile. Minimizing the number of care providers obtaining the measurement and overtly standardizing the measurement site and technique improves accuracy. The effect of interpreter variability can be dampened by comparing the OFC to the previous day and also to 1 week earlier to calculate average daily growth by dividing by 7. Using a correct and accurate growth curve is also important (12). In some conditions, such as achondroplasia, OFC growth is different, and a centile chart specifically for this condition has been derived.
The increasing availability of detailed antenatal imaging with ultrasound and fetal MRI has allowed earlier diagnosis of congenital hydrocephalus, providing an opportunity to manage such pregnancies prospectively. Fetal ventriculomegaly is defined as an atrial width of over 10 mm; this same value applies throughout pregnancy (13). Ventriculomegaly is present in 2 per 1,000 live born babies (13). For isolated mild fetal ventriculomegaly (10 to 15 mm) diagnosed after 24 weeks’ gestation, the risk of moderate-to-severe neurodevelopmental delay is only 4%; 83% show normal development and 8% minor delay (14). Severe ventriculomegaly (>15 mm) is often associated with other neurologic abnormalities, which then primarily influence prognosis. In a recent large series, fetal hydrocephalus due to intracranial hemorrhage or associated with corpus callosum agenesis, atresia of the foramen of Monro, or arachnoid cyst had a good prognosis; those associated with holoprosencephaly, encephalocele, or virus infection demonstrated poorer outcomes (15).
Benign external hydrocephalus is characterized by a rapid increase in HC. This is most prominent around the age of 6 months. Imaging shows enlargement of the subarachnoid spaces with slightly enlarged or normal-sized ventricles. There is at least one close relative with macrocephaly in up to 90%. HC growth stabilizes by 18 months and then continues to follow a higher centile parallel to the 98th. This condition probably represents delayed maturation of CSF absorption capacity, and CSF diversion is not required (16). In macrocephalic children, it is important to exclude glutaric aciduria type I, which, if identified early, can be treated with dietary and metabolic measures, reducing the risk of significant neurologic morbidity (17).
Treatment of Neonatal Hydrocephalus
Due to the disadvantages and complications of VP shunting, the decision to place a shunt must be made only after all nonsurgical options have failed. The fact that the CSF resorption may change with time, and indeed may improve, provides a rationale for conservatism. As a patient grows and the dynamic relationship between CSF production and resorption changes, the need and indications for diversion of CSF may change over the child’s lifetime.
Children born with hydrocephalus due to nonhemorrhagic causes, such as aqueductal stenosis, posterior fossa cysts, holoprosencephaly, or hydranencephaly, can be shunted within 1 to 2 days of birth, pending evaluation of general medical status. Although affected infants usually are born at or near term, a presurgical weight of approximately 1,500 to 2,000 g is adequate size to support peritoneal drainage of ventricular CSF (18). There are ethical issues that should be raised before placing a VP shunt in a child suffering from hydrocephalus with significantly reduced brain function from either an in utero event or a developmental anomaly. Frank discussion with the child’s parents regarding prognosis is critical. Shunting for affected children without other life-threatening anomalies is certainly reasonable for the purposes of controlling head size in the growing infant. With maximal interventive therapy, most of these children are expected to live beyond a few weeks, and such day-to-day issues as whether the child’s head will fit in an infant car seat need to be considered. Shunting at birth is a relatively low-risk method of preventing massive head enlargement and allowing other factors aside from congenital hydrocephalus to determine the child’s outcome (19). Despite the progress in endoscopic techniques and the establishment of endoscopic third ventriculostomy (ETV) for CSF diversion in obstructive hydrocephalus, its efficacy in neonates remains controversial. A large Canadian review of 368 children who underwent ETV in nine centers showed that the 5-year success rate for ETV in children under 1 month of age was only 28% (20). This has, however, been disputed in smaller studies that have emphasized that the underlying etiology of hydrocephalus is more relevant to prognosis than to age (21,22). The International Infant Hydrocephalus Study, an ongoing randomized controlled study evaluating the effectiveness of ETV in children under 1 year as compared to shunt, is expected to report in 2015. Nearly all acquired hydrocephalus in the premature infant is posthemorrhagic (Fig. 47.1). These infants have usually not achieved adequate weight for placement of permanent shunts, and the final determination of lifelong hydrocephalus has not been made. Serial lumbar punctures are, initially, the best way to reduce ventricular size and intracranial pressure. As the cranial sutures are open, the risk of coning after lumbar puncture (LP) is extremely rare in this situation. Up to 10 mL/kg of CSF may be removed at one time. As these neonates often have a combination of communicating and obstructive hydrocephalus, LPs often soon become ineffective. Obstruction of CSF pathways at the spinal level may also occur (23). Direct ventricular puncture through the anterior fontanelle is not ideal, and parenchymal needle tracks appear on ultrasound examination after multiple aspirations. In the ventriculomegaly trial, the risk of ventriculitis and repeated LPs was 7% (24). In practice, once two LPs or one ventricular tap has been necessary to control ventriculomegaly, insertion of a ventricular subgaleal shunt or ventricular access device (VAD) would be recommended (25). Acetazolamide and furosemide were investigated in the International Post-hemorrhagic Ventricular Dilatation (PHVD) Drug Trial Group study in a randomized trial in 1998 (26). The trial was discontinued prematurely by the data monitoring committee as it became clear that the treatment group fared worse. This was likely related to PaCO2 elevation in ventilator-dependent neonates with immature renal function.
An additional approach that has been tried for posthemorrhagic hydrocephalus (PHH) is the introduction of fibrinolytic therapy directly into the ventricular system. Enzymes such as streptokinase, urokinase, and tissue plasminogen activator all have been proposed and tested with variable success. Some centers have suggested that the risk of VP shunts can be reduced using this technique (27). Others have been unable to show a decrease in the need for VP shunting with this maneuver (28,29). It is possible that the trials that found no helpful effect from fibrinolytic therapy had less favorable results because of patient selection, with a trend toward enrolling the most severe and potentially intractable cases.
Drainage, irrigation, and fibrinolysis therapy (DRIFT) developed out of an attempt to reduce intracranial pressure and wash out toxic cytokines from the ventricular system as early as possible (30). This involves insertion of right frontal and left occipital external ventricular catheters. Tissue plasminogen activator is injected and, 8 hours later, irrigation with artificial CSF is commenced under intracranial pressure monitoring. The drainage fluid clears from dark and thick to straw colored CSF within about 72 hours. A phase I study enrolled 24 infants with ventriculomegaly (97th centile +4 mm) (30). Mortality, disability, and shunt dependence (74%) were lower than that of historical controls. Seventy infants, between 24 and 34 weeks’ gestation, were subsequently randomized in a multicenter trial to DRIFT or standard treatment (31). Although shunt insertion rates were not different, a follow-up study at 2 years demonstrated that severe cognitive disability was significantly reduced in the DRIFT group (32). Seventy-one percent of infants who had received standard treatment were severely disabled or dead, compared to 54% in the DRIFT group. Thirty-one percent of infants surviving DRIFT had severe cognitive disability, compared to 59% in the standard treatment group. However, larger trials are needed to further delineate the risks and benefits of this high-risk, labor-intensive treatment. At this point, the underlying difficulty with fibrinolytic therapy is the lack of overlap of the safety and efficacy windows. This therapy cannot currently be considered standard therapy.
![]() FIGURE 47.3 Ventricular access device with 30-mm catheter. Implantation allows easy percutaneous access to ventricular fluid. |
Persisting increase in ventricular size in the preterm after IVH neonate, still too small to allow implantation of a VP shunt, is managed by insertion of a VAD or a ventricular subgaleal shunt, allowing intermittent or continuous CSF drainage. A VAD provides a safe route for repeated aspiration of ventricular CSF, with low reported infection rates (Fig. 47.3) (33,34). Insertion, through the frontal corner of the anterior fontanelle, can be safely performed in neonates under 800 g. In a large series of 325 preterm infants with grade III to IV IVH studied over a 10-year period, 65 infants underwent insertion of a VAD. There were four infections during the course of treatment, and three had to be revised due to malfunction (34). Revision and infection rates were similar in a Dutch study on 76 preterm infants over a 12-year period (33). Tapping frequency is determined by regular measurements of the ventricular index. Ventriculosubgaleal shunts are also used as temporizing devices prior to placement of a VP shunt. The device, as well as the surgical implantation technique, is very similar to the VAD. The ventricular catheter is connected to a reservoir with a side arm. Either this can be left open into the subgaleal space or 10 cm of shunt tubing may be attached to it and coiled within the subgaleal pocket, fashioned by blunt dissection between the pericranium and aponeurosis of the galea. The major advantage is that the subgaleal pocket may allow some resorption of CSF and certainly can absorb fluid pulsations. Infants with ventriculosubgaleal shunts typically require far less frequent taps and sometimes no taps at all (24). If necessary, the reservoir or subcutaneous fluid collection can be tapped, just like a VAD. The cosmetic issue of a visible subcutaneous fluid-filled space can be concerning to parents and care providers who are unfamiliar with this technique, but all can be reassured that this will resolve after placement of a VP shunt, which follows in the vast majority of cases. The indications for tapping ventriculosubgaleal shunt devices are the same as for VADs, and the follow-up assessment tests are identical, including HC, sonographic assessment of ventricular size, and RI. In the large St Louis series, there was no difference between VADs and ventriculo-subgaleal (VSG) shunts in terms of infection, need for revision, shunt insertion rate, shunt infection, and revision rate or mortality rate (34).
Another intervention that has been suggested is the use of ventriculoscopic removal of clot; however, based on little published experience, this has not been demonstrated to avert the need for VP shunts. A recent report describes endoscopic aspiration of IVH in four neonates, between 30 and 40 weeks’ gestational age (35). Hematomata were aspirated piecemeal through the endoscope. The contralateral ventricle was accessed through a septostomy, although in two out of four neonates contralateral endoscopy was necessary to aspirate the hematoma satisfactorily. Only one of the four neonates did not require a VP shunt (35). The risk of PHH correlates with the severity of intraventricular hemorrhage. Although other grading systems have been described, we use the Papile four-level system of intracranial hemorrhage (36): grade I, subependymal hemorrhage only (no increased risk of PHH); grade II, intraventricular hemorrhage without ventriculomegaly (low risk of progressive PHH); grade III, intraventricular hemorrhage with ventriculomegaly (moderate risk of progressive PHH); and grade IV, intraventricular hemorrhage with intraparenchymal extension (generally at high risk of need for VP shunting, depending on degree of intraventricular component).
Traditionally, decisions to drain CSF were taken on the basis of signs and symptoms of raised intracranial pressure. These included a tense anterior fontanelle, rapidly increasing OFC, splaying of cranial sutures, as well as episodes of bradycardia and apnea. The current trend is to intervene earlier on the basis of serial ventricular measurements on ultrasound. The broadly accepted action point is a ventricular index 4 mm above the 97th centile. Physiologically, earlier drainage maintains a lower intracranial pressure over a longer period of time and reduces white matter stretch. In a survey of 37 neonatal intensive care units in Europe, 72% started therapy once the ventricular index crossed 4 mm over the 97th centile; 25%, however, intervened earlier, when the 97th centile was crossed (37). In a Dutch study, early intervention resulted in a lower shunt requirement rate than did intervention at the 4 mm +97th centile, and the incidence of moderate or severe disability was higher in the late group (38). This was not a randomized study, however, and further research is required. An important difficulty with such studies is that infants treated “early” may have stabilized and not required any intervention at all if the later action point had been awaited. A prospective randomized trial, ELVIS (Early vs. Late Intervention Study) is currently recruiting patients. In three recent large single-centre studies, the rate of shunt implantation for neonates with ventricular dilatation following grades III to IV IVH have varied between 16% and 32% (34,39,40). The decision to insert a VP shunt should not be taken too early. Shunts in this population have a higher rate of failure and infection (8,41
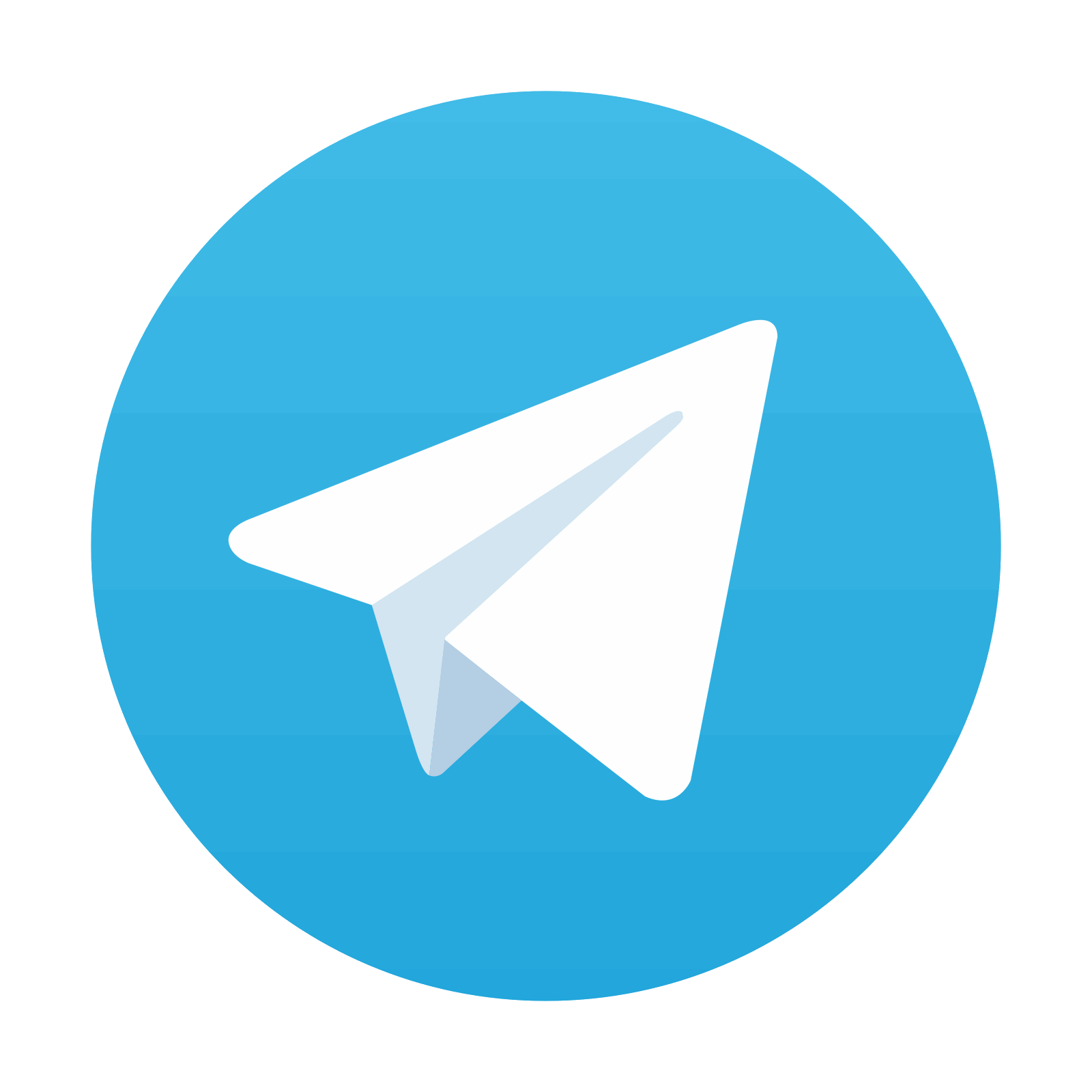
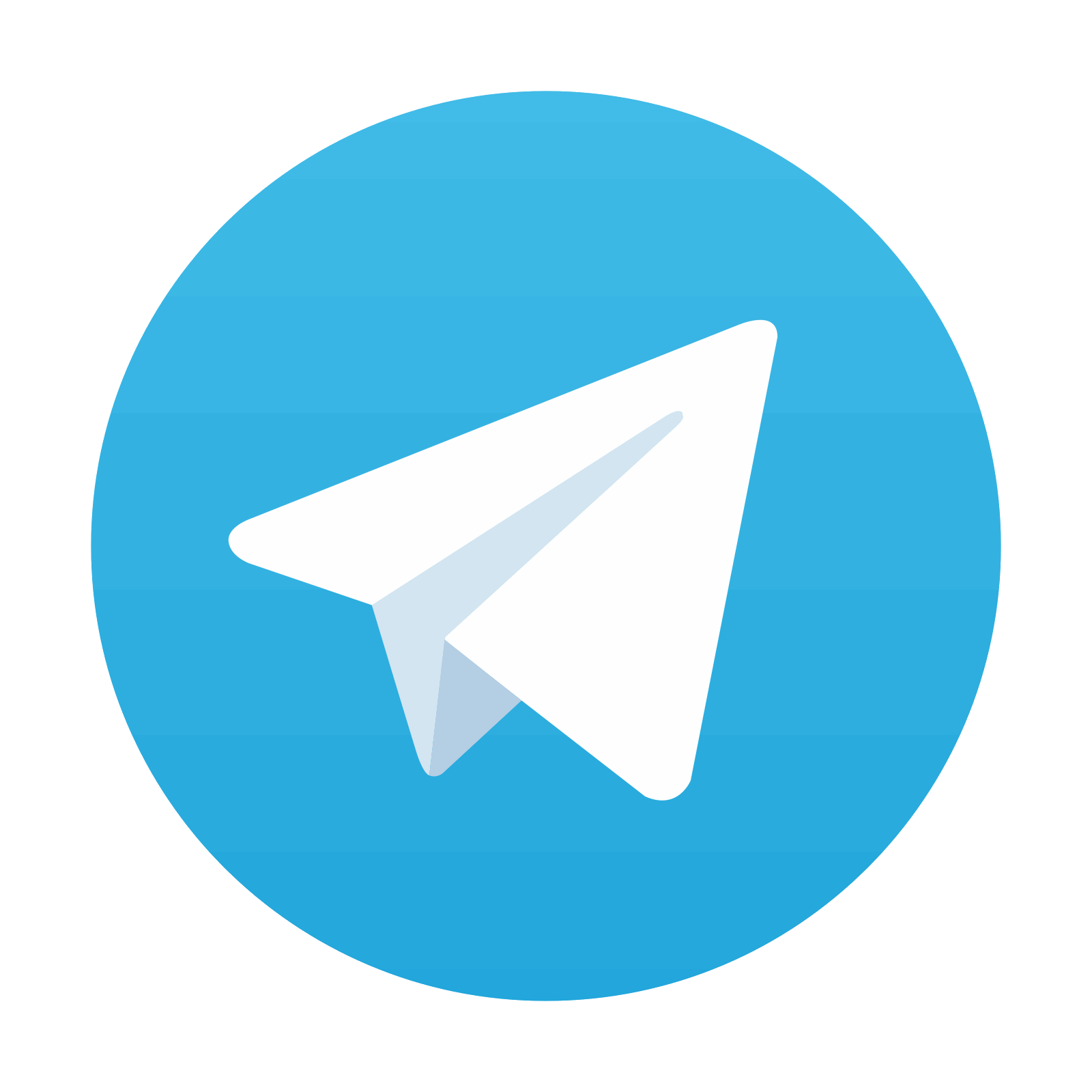
Stay updated, free articles. Join our Telegram channel

Full access? Get Clinical Tree
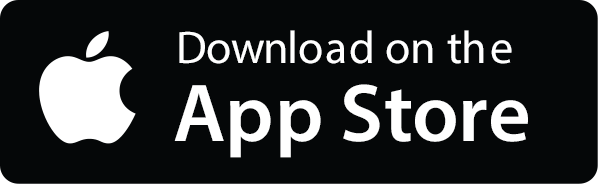
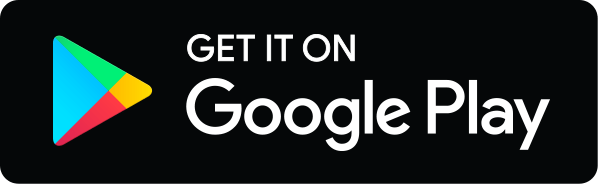