At specific times in the development of each long bone, two secondary centers of ossification appear—the growth plates on either end of the long bone. The growth plate, also known as epiphyseal plate, is an area where formation of new bone is possible. It is located at each end of long bones. Secondary ossification centers mostly occur after birth, with the exception of the growth plate of the distal femur and the proximal tibia, which develop during the perinatal period. From these centers of ossification, the end of each long bone is enlarged by centrifugal growth and allows the process of ossification to spread rapidly from the ossification centers. However, the rate of appositional growth from the secondary center is much slower than that of the primary growth plate. This development of new bone continues until closure of the growth plate at skeletal maturity.
As it is well known, a long bone is divided into its proximal epiphysis, proximal metaphysis, diaphysis (shaft), distal metaphysis, and an epiphysis at the distal end. The growth plate is found in the area of the metaphysis and holds the area of endochondral ossification. As we go from the epiphysis to the diaphysis, there first is an area of articular cartilage forming a cap onto the epiphysis (Fig. 37.2). Especially in long bones such as the femur, where the proximal epiphysis is part of the hip joint, this articular surface is essential for allowing movement in the joint. Therefore, the articular cartilage in this area will be required to transmit weight and will function as a so-called pressure epiphysis. Underneath the articular cartilage, the secondary ossification center of the epiphysis is located. It is supplied by the epiphyseal artery, which enables continuous immigration of osteogenic cells.

The growth plate can be anatomically divided into three types of tissue components: a cartilaginous component (epiphyseal plate), an ossific component (metaphysis), and a fibrous component surrounding the periphery of the plate. The cartilaginous part, which is composed of four different histologic zones, holds the essential stages during which new bone is created. This area is encircled by a fibrous component made up of the groove of Ranvier and the perichondral ring of LaCroix. Adjacent to the cartilage, we find a bony metaphysis.
During childhood, the growth plate consists of connecting cartilage, enabling the long bone to grow in length and girth. At adulthood, the components terminate growth and the growth plate completely ossifies into solid bone.
The Cartilaginous Component
The cartilaginous component of the growth plate is divided histologically into four zones, described as the resting zone, the proliferative zone, the hypertrophic zone, and the zone of provisional calcification (Fig. 37.3).
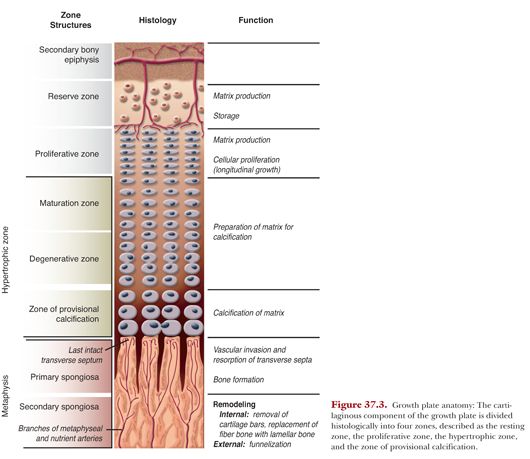
Located immediately below the secondary center of ossification, there is a zone of undifferentiated or resting cartilage cells, named the reserve zone. This zone contains irregularly scattered cartilage cells with a high lipid content, suggesting a nutrient storage potential. These cells act as precursor cells for the developing cartilage cells in the next zone. Injury to this zone is deleterious because it often results in a partial or complete arrest of growth. Adjacent to the reserve zone, there is the zone of proliferating cartilage. This zone comprises about half of the overall height of the physis. Although chondrocytes still appear rather flat in this area, they already start to proliferate. At the base of these columns, mitotic activity is found. Cells rapidly divide and become organized into columns, laying down a cartilage extracellular matrix that will later serve as a scaffold for future osteoblasts, which in the future allow the process of ossification. The entire growth in length of the long bone occurs at this location. In the zone of maturation or hypertrophic zone, which follows, cells are still organized in columns, but they enlarge to 5 to 10 times the size of the proliferating zone.
In this layer, there is no active growth and the chondrocytes begin to terminally differentiate, resulting in cell death, triggering blood vessel invasion and bone formation.
As the chondrocytes become hypertrophic, the extracellular matrix, consisting of collagens and proteoglycans, is diminished. This explains why the hypertrophic zone is the weakest: It lacks both collagen and calcified tissue. Therefore, most physeal separations and fractures occur through this layer because it is less resistant to stress than the other parts of the growth plate.
As endochondral ossification depends on the invasion of blood vessels and matrix remodeling, blood vessels enter the partially dismantled scaffold in the zone of provisional calcification. This process allows osteogenic progenitor cells to invade and deposit trabecular bone. The remodeling process causes variations in calcium and phosphate levels in the matrix at the junction of cartilage and bone. The variations in ion concentrations, matrix degradation and mineralization, and influx of new cell types all regulate the equilibrium of cartilage degradation to bone formation.
The Fibrous Structure
Surrounding the periphery of the growth plate lays a wedge-shaped groove of cells, called the groove of Ranvier,15 and a ring of fibrous tissue, the perichondral ring of LaCroix.16 The groove of Ranvier contains cells, which are active in division and contribute in the increase of the width of the growth plate. The structure of the perichondral ring of LaCroix is a fibrous collagenous network that is continuous with the fibrous portion of the groove of Ranvier and the periosteum of the metaphysis. Its function is to mechanically support and stabilize the bone–cartilage junction of the growth plate.17–20
The Metaphysis
The metaphysis holds the function of removal of the cartilaginous matrix and its replacement with bone. Its beginning is in the last intact transverse septum of each cartilaginous cell column. Lysosomal enzymes remove the last transverse septum and allow for endothelial and perivascular cells to invade. Between capillaries and osteoblasts that line the calcified bars, osteoprogenitor cells start the process of ossification. This area of only little or no bone formation is termed the primary spongiosa.21 Using the cartilaginous scaffold, osteoblasts progressively form bone, creating the secondary spongiosa. The initial fiber bone is soon replaced by lamellar bone by the process of synchronized osteoclastic bone resorption and osteoblastic bone formation.
Vascular Supply of the Growth Plate
The growth plate and the epiphyseal region are supplied by three vascular systems: the epiphyseal, metaphyseal, and perichondral blood supply.1 The epiphyseal artery mostly secures vascular supply of the epiphysis. It divides into small branches forming a network of vessels, which enter the epiphysis from different sides. Each small branch spreads out in a tree-like fashion and supplies 4 to 10 cell columns. Therefore, the proliferative zone is well perfused to allow for cell proliferation and division.22
In the past, an existence of transphyseal vessels was uncertain and subject to intense controversy. However, recent studies using modern imaging techniques have revealed blood vessels that cross the growth plate.23
The metaphysis is richly supplied with arterial blood from the nutrient artery as well as from the metaphyseal arteries arising from the ascending cervical arteries. About 80% of the metaphyseal vessels derive from the nutrient artery, which divides into several branches that supply the central region of the metaphysis.
The perichondral vessels supply the fibrous peripheral structures of the growth plate, such as the groove of Ranvier and the perichondral ring of LaCroix. Branches surround the growth plate and send anastomoses to both the epiphyseal and the metaphyseal arteries.24,25
THE INFLUENCE OF RECONSTRUCTIVE SURGERY AROUND THE KNEE ON THE DISTAL FEMORAL GROWTH PLATE
Participation of children and adolescents in sports is on the rise, with young athletes training year-round and specializing at earlier ages, predisposing them to sports-related injuries. Once thought to be rare, injuries of the anterior cruciate ligament (ACL) and medial patellofemoral ligament (MPFL) are seen with a greater frequency in the pediatric population. Numerous preventive training programs have been developed but are yet to be established among the society.26 Even though ACL injuries are among the most extensively researched orthopedic injuries and treatment options have evolved, there are still many controversies surrounding the appropriate treatment of these injuries in children and adolescents.
Anterior Cruciate Ligament Injury
Today, there seems to be a consensus among pediatric orthopedic surgeons that reconstructive surgery after a complete tear of the ACL or the MPFL yields a favorable outcome for pediatric and adolescent patients.27–34 Although conservative management is an appealing option given the increased potential of regeneration in children, clinical results after nonoperative treatment have not been satisfactory.35–38 In cases of partial tears of the ACL, nonreconstructive management may be successful in younger children and adolescents.30,34
However, reconstructive surgery allows for early mobilization and participation in sports and may prevent further meniscal injury and articular cartilage damage, which can result in early-onset osteoarthritis.27,39–44 Especially in cases where bone bruising is detected on magnetic resonance imaging (MRI) studies, the incidence of intra-articular injuries such as meniscal tears seem to be increased.45–47 Bisson et al.45 stated that 90% of all subjects were found to have bone bruising on MRI after injury of the ACL. In their cohort, moderate and severe bone bruising seen on MRI was associated with an increased arthroscopic finding of medial meniscal tears.45 Yoon et al.47 found that medial meniscal injury was detected in 66% of cases with medial and lateral bone contusion.
In a meta-analysis from 2013, Ramski et al.32 were able to show that benefits in multiple outcomes favor early surgical stabilization over nonoperative treatment. They found that children and adolescents suffering from an ACL injury who underwent nonoperative or delayed treatment were likely to experience more instability and pathologic laxity.
Furthermore, many were more frequently unable to return to their previous activity levels when compared to patients treated with surgical reconstruction of their ACL.32 Many authors published similar results and concluded that up to 50% of children treated nonoperatively for a complete ACL tear did not return to athletic activity.48
There has been ongoing discussion and research regarding the optimal technique to reconstruct the ACL in children and adolescents. Generally, modern techniques can be categorized in extraphyseal reconstruction, transphyseal reconstruction, and all-epiphyseal reconstruction. Each one is directed toward restoring the stability of the knee, with extraphyseal and all-epiphyseal techniques trying to minimize the risk of iatrogenic physeal injury (see Section 1 on Anterior Cruciate Ligament Injury).
Patellar Dislocation
Patellar dislocation is commonly diagnosed in children and adolescents and often results in severe patellofemoral instability. The MPFL has been recognized as a strong stabilizer of the patella, providing 50% to 60% of the innate medial passive resistance. Lateral dislocation of the patella results in disruption of this ligament. The initial event of dislocation is frequently traumatic in origin, with recurrent episodes of patellar dislocation after a low-energy trauma or even during forceful flexion of the knee. In a previous study, Kepler and colleagues49 were able to detect that in 44 children and adolescents with acute primary MPFL disruption, injury occurred at the patella in 61% of cases, at the femur in 12% of cases, and at both sites in 12%. Ligamentous laxity, skeletal dysplasia, or collagen disorders such as Marfan or Ehlers-Danlos syndrome predispose patients to patellar dislocation.
Even though conservative treatment with short immobilization followed by physical therapy (concentrating on the evaluation of gait patterns and restoring potential preexisting muscle imbalances and the mechanical axis) can be successful in cases of traumatic rupture of MPFL, surgical reconstruction is recommended if conservative management is ineffective and recurrent episodes of patellar dislocation occur. In cases of atraumatic patellar dislocation, initial conservative treatment is often ineffective and high rates of redislocation—up to 80%—have been reported.50,51
However, surgical treatment in skeletally immature patients is still a subject of discussion. More than 100 different surgical techniques exist to stabilize the patella and address various contributing anatomic factors that may promote recurrent dislocation of the patella, such as the patellofemoral osseous geometry, overall limb alignment, and physeal anatomy.
Many authors advocate surgical reconstruction of the MPFL, allowing skeletally immature patients to quickly return to their full level of activity.52–55 Recent studies have shown that short-term results seem to favor reconstruction over repair of the MPFL in children and adolescents.56,57 Therefore, several physeal-sparing operative techniques have been presented and discussed in the literature.58–64 In order to achieve satisfactory long-term results, it is essential to restore biomechanical kinematics of the knee through an anatomic reconstruction of the MPFL.
When planning an MPFL reconstruction in a child with open physis, the anatomic relationship of the MPFL insertion around the distal femoral physis needs to be considered. The MPFL insertion on the medial condyle of the femur has been described to be distal to the adductor tendon insertion and proximal to the femoral insertion of the medial collateral ligament, with close proximity to the medial aspect of the distal femoral growth plate.55,63,65 Kepler and colleagues49 as well as Balcarek66 and Nelitz and colleagues67 have further investigated the origin of the MPFL and found the zone of insertion to be just distal to the distal femoral physis. This knowledge is essential in order to avoid injury of the growth plate and to allow for an anatomically correct reconstruction (Fig. 37.4). Bone fixation proximal to the distal femoral physis may result in proximal migration of the femoral insertion of the MPFL, causing for loss of graft tension, graft isometry, and loss of motion in the knee (see Section 2 on Patellofemoral Instability).
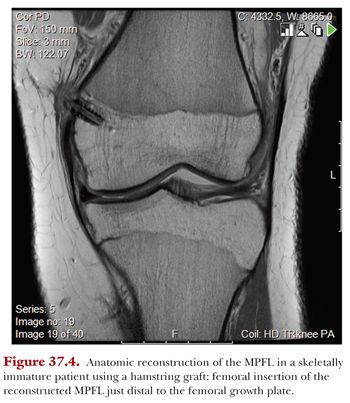
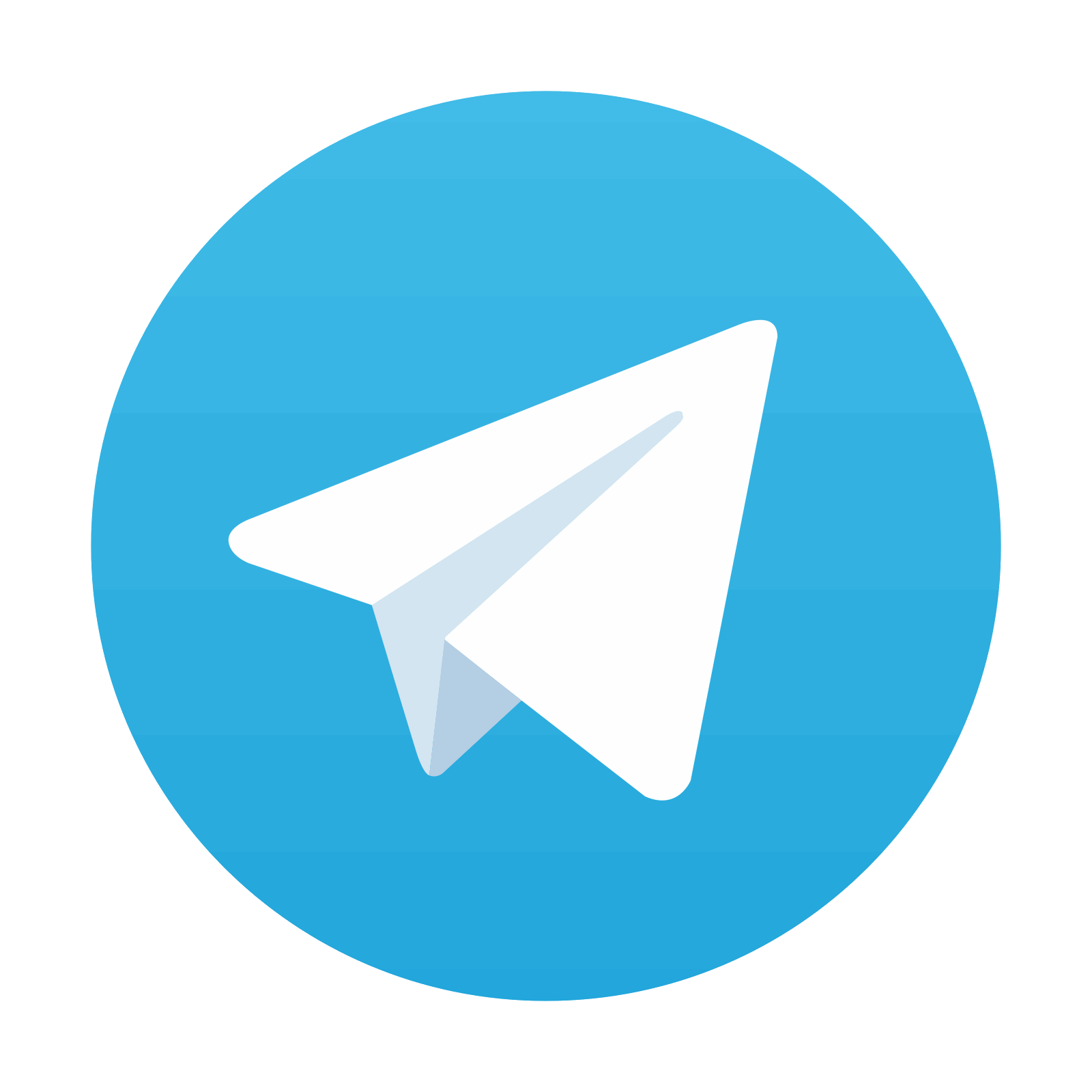