Gastrointestinal Disease
Jon A. Vanderhoof
Rosemary J. Pauley-Hunter
Although most pediatric gastroenterologists are uncomfortable with primary care of the sick premature infant, they often are valuable consultants to the neonatologist. In evaluating a complex gastrointestinal (GI) or hepatobiliary problem, a gastroenterologist often uses an organ system-specific developmental pathophysiologic approach. The opinion of the consultant may augment the analysis of the primary physician; however, it remains the responsibility of the neonatologist to put the consultant’s view into perspective as it relates to the other complex problems of the sick neonate.
The gastroenterologist also may offer his or her skills in invasive procedures to aid in the diagnosis of GI and liver disease. Upper and lower GI endoscopy, liver biopsy, rectal biopsy, esophageal, antroduodenal, colonic and anorectal motility studies, and even endoscopic retrograde cholangiopancreatography can be performed in term infants and, depending on the skill and training of the gastroenterologist, in premature infants as well. In some institutions, gastroenterologists with expertise in nutrition provide assistance for parenteral nutrition-dependent or malnourished infants. Their role becomes especially important in infants with GI or liver disease who may require long-term follow-up, such as the infants with progressive liver disease or short-bowel syndrome who require home parenteral nutrition.
▪ DEVELOPMENT OF THE GASTROINTESTINAL TRACT
Subsequent to the development of the individual organs of the GI tract, specialized features of the system begin to become apparent, mostly in the second and third trimesters (1). At approximately 14 weeks of gestation, differentiation of the pancreatic endocrine and exocrine tissues begins, and crypts and villi begin to form in the small intestine. A few weeks later, the colon, initially populated with villi similar to those in the small intestine, begins to develop its more characteristic surface, with gradual loss of villi. As these morphologic changes occur, numerous functional processes begin, some of which mature early in utero, some only at birth, and some during the 1st year of life.
Carbohydrate Absorption
The functional maturation of the digestive process is complex (2). There are marked differences in maturation of the digestive and absorptive processes of different nutrients (Table 37.1). In the neonate, most dietary carbohydrate is presented in the form of lactose, the predominant carbohydrate in virtually all mammalian milk. Lactose and other disaccharides are digested by enzymes located on the brush border membrane in mature enterocytes, those located on the distal and midportions of the small intestinal villi. Component monosaccharides are released after hydrolysis by disaccharidases. Lactase hydrolyzes lactose to glucose and galactose, and both subsequently are transported by active carriermediated transport (3). Other disaccharidases include maltase, which hydrolyzes maltose to two glucose units; glucoamylase, which hydrolyzes glucose oligosaccharides to glucose monomers; and sucrase, which hydrolyzes sucrose to fructose and glucose (4). Sucrase is actually a double enzyme, the other part of the molecule being isomaltase, which hydrolyzes &agr;-1-6 bonds of &agr;-limit dextrins. Disaccharidase activities are highest in the proximal and midjejunum and decrease distally.
Lactase activity develops later in gestation than do the other disaccharidases. Although other disaccharidase levels can be detected somewhat earlier in gestation and reach nearly adult levels between 26 and 34 weeks of gestational age, lactase levels are only 30% of full-term levels by that point in gestation. Functional lactase activity typically develops within the 1st month of the premature neonates’ life (5). Salivary glands produce an amylase that may be important in the digestion of complex carbohydrates in the newborn. This enzyme is detectable at 20 weeks of gestation and is present in significant quantities in premature infants. As with pancreatic amylase, however, the ability of the newborn to secrete sufficient salivary amylase is substantially reduced and matures throughout the 1st year of life. Salivary amylase is inactivated by gastric acid but probably retains some activity in the stomach of premature infants. Glucoamylase is a brush border enzyme capable of digesting glucose units from the nonreducing ends of starch and dextrin. Glucoamylase is present in neonates and infants at 50% to 100% of adult levels (6).
Finally, it is probable that some malabsorbed carbohydrate is digested in the colon through the colon salvage pathway. Colonic anaerobic bacteria are capable of metabolizing carbohydrates to produce short-chain fatty acids that then are absorbed through the colonic mucosa (7). Considering the relative pancreatic insufficiency and lactase deficiency in the newborn infant, the colon salvage pathway may be an important mechanism by which infants absorb carbohydrates. See Chapter 34 for further information on carbohydrate homeostasis.
Fat Absorption
Fat absorption is a complex process, primarily because fat is insoluble in the aqueous environment of the small intestinal lumen (8). Solubilization, therefore, is an important part of the fat assimilation process. The first phase of fat absorption is that of enzymatic digestion or lipolysis. Because most dietary fat is present in the form of triglycerides, otherwise known as triacylglycerols, these first must be hydrolyzed by pancreatic lipase.
Phospholipids are hydrolyzed concurrently by pancreatic phospholipase. Colipase, a cofactor secreted by the pancreas, also is required, facilitating the action of lipase by binding to bile saltlipid surfaces and improving the interaction of lipase with triglyceride. The efficiency of this process is augmented by the release of cholecystokinin (CCK) from the duodenal epithelium, which occurs in response to the presence of lipid and protein in the duodenum. CCK stimulates pancreatic secretion, gallbladder contraction, and simultaneous relaxation of the sphincter of Oddi, to mix large quantities of bile acids and digestive juices with lipids. Pancreatic lipase levels are reduced in preterm infants and intrauterine growth-retarded infants, significantly impairing lipolysis (9). Lingual lipase, secreted from the salivary glands, may facilitate lipolysis in the premature infant and partially compensate for the infant’s relative pancreatic insufficiency (10). Nonetheless, fat absorption is significantly impaired in newborn infants and, to a greater extent, in premature infants, due at least in part to pancreatic insufficiency.
Closely linked with the process of enzymatic digestion of fats is micellar solubilization by bile acids (11). Bile acid molecules are complex structures with both hydrophobic and hydrophilic ends. Bile acids interface with lipids to render them water soluble by positioning the hydrophobic portion in close proximity to the lipid globules although allowing the hydrophilic portion to remain free to interact with the aqueous environment. Lipids then become enclosed in disc-shaped water-soluble micelles that contain fatty acids, monoglycerides, phospholipids, cholesterol, and fat-soluble vitamins.
TABLE 37.1 Digestive and Absorptive Function in Infants Relative to Adults | ||||||||||||||||||||||||||||
---|---|---|---|---|---|---|---|---|---|---|---|---|---|---|---|---|---|---|---|---|---|---|---|---|---|---|---|---|
|
Solubilization is particularly important because of the presence of the intestine’s unstirred water layer. This stagnant layer of water overlies the microvillus membrane of the intestinal epithelial cells and is the primary barrier to lipid transport. The actual thickness of the unstirred water layer is complex and difficult to measure, but the layer is significantly reduced by the constant agitation of the fluid in the GI tract as a result of gut motility and villus contraction. Because of the convolutions in the small intestine caused by the presence of villi and microvilli, the total surface area available to interface between the intestinal surface and the unstirred layer is much greater than the interface between the unstirred water layer and the aqueous intraluminal environment. Penetration through the unstirred layer by the disc-shaped micelles is the rate-limiting step for lipid absorption. Disease processes that increase the unstirred water layer thickness will markedly inhibit fat absorption in much the same manner as disease states that render the supply of bile acids inadequate for micellar solubilization. Bile acids commonly are deficient in cholestatic liver diseases, such as neonatal hepatitis or biliary atresia, and in rare cases of congenital bile acid deficiency. Bile acids are deconjugated rapidly and reabsorbed in the presence of small intestinal bacterial overgrowth. Bile acid deficiency therefore may occur in patients with disorders that cause intestinal stasis and bacterial overgrowth, such as short-bowel syndrome. In disorders of mucosal injury, the unstirred layer thickness may be increased, making penetration of the fat-containing micelles difficult and further exacerbating fat malabsorption.
Bile acids are extremely important in the fat absorption process. In the absence of bile acids, only about one-third of dietary triglycerides, a very small percentage of fatty acids, and virtually no cholesterol or fat-soluble vitamins are absorbed. Medium-chain triglycerides may be better absorbed because of their enhanced water solubility, which allows penetration of the unstirred water layer without micellar solubilization. In both preterm and term infants, bile acid synthesis is limited and the bile salt pool size is low (12). Moreover, preterm infants may have an ineffective bile salt transport process in the distal ileum, resulting in impaired enterohepatic circulation of bile salts (13). Consequently, the bile acid concentration may be less than adequate for the formation of micelles and solubilization of fat. Thus, penetration of the unstirred layer is less efficient in the term infant and further impaired in the preterm infant compared to adults.
After lipids are enclosed in the bile acid micelle and reach the lipid bilayer membrane of the small intestinal mucosal cell, absorption into the cell occurs by passive diffusion. Because of the convolutions of the GI tract, a large surface area exists for lipid assimilation. In the absence of disease, this process progresses in the term and preterm infant relatively uninhibited. In disorders in which the absorptive surface area is reduced or damaged, however, such as in short-bowel syndrome or any form of diffuse enterocolitis, fat, carbohydrate and, to a limited degree, protein are malabsorbed.
Within the enterocyte, monoglycerides and esterified fatty acids are immediately resynthesized to triglycerides. These triglycerides, along with apoproteins, phospholipids, free cholesterol, some diglycerides, and esterified cholesterol, are stabilized within chylomicrons. The outer structure of the chylomicron then fuses with the basolateral membrane and is extruded into the lamina propria, and the triglycerides are carried by the lacteals and lymphatic channels into the blood stream.
Protein Absorption
The assimilation of protein begins in the stomach through the action of hydrochloric acid and pepsin. The maturational aspects of this process have been the subject of substantial study and some controversy. Conflicting data exist regarding the status of acid secretion in the newborn infant. Newborn infants appear to be capable of secreting acid, although the process is somewhat immature (14). Recently it was found that at 24 weeks of gestation, infants have gastric acid output similar to adults (15). Pepsinogen, the proenzyme for pepsin, which facilitates protein digestion in the stomach, is secreted in preterm infants in lower concentrations until approximately 31 weeks of gestation age (16,17).
The gastric aspects of protein digestion are relatively inconsequential, compared to the much more complete process in the small intestine. Enterokinase, produced in the duodenal mucosa, activates the pancreatic proteolytic enzyme trypsinogen, converting it to trypsin, which then activates essentially all of the other enzymes involved in protein digestion. Enterokinase levels have been demonstrated in human fetuses as early as 21 weeks of gestation (18). Enterokinase expression is diminished during fetal development, however, and is only 10% of adult levels in the term newborn. Additionally, pancreatic and duodenal proteolytic enzymes are present in preterm and term infants in lower concentrations than in older children and in adults. These enzymes initiate hydrolysis of proteins, and the hydrolysis process is completed by brush border and cytosolic peptidases. Protein is absorbed in the form of amino acids and dipeptides through active transport processes that appear to be well developed by 28 weeks of gestational age. Despite the relative immaturity of multiple phases of the protein assimilation process, both preterm and term infants are quite capable of absorbing adequate quantities of dietary protein (19). In small infants, the protein malabsorption resulting from mucosal injury is probably far less consequential than is the malabsorption of the other major macronutrients.
Micronutrient Absorption
Absorption of micronutrients matures at varying rates in infancy. Water is absorbed passively in response to sodium and other electrolytes, as it is in older children and adults (20). Experimental evidence suggests that the intestinal epithelium may be more secretory during early infancy, and the increased susceptibility of infants to diarrheal disorders probably is at least partially related to this process.
Mineral absorption depends on the form in which the mineral is presented to the infant. Iron, for example, is absorbed extremely well from breast milk. Even the preterm infant is capable of absorbing nearly 50% of the iron in breast milk, whereas only a small percentage of iron is absorbed from cow milk formulas. Iron is taken up in the small intestine by divalent metal transporter-1 and is either stored in mucosal cells by ferritin or transported to the systemic circulation by ferroportin. Hepcidin, a small peptide synthesized by the liver, can sense iron stores and regulates iron transport by inhibition of ferroportin; however, regulation of these iron transporters is immature in infants (21). Calcium and phosphorous also are well absorbed from breast milk (22,23). Magnesium, copper and, to a lesser extent, zinc are well absorbed by both term and preterm infants (24). In general, minerals are absorbed somewhat better from breast milk than from cow milk. Methods to increase absorption using glucose polymers,
hydrolyzed formulas, and additives such as oligosaccharides have shown some benefit (25,26,27). Most vitamins appear to be absorbed adequately in both term and preterm infants, although fat-soluble vitamin deficiency is common in disorders affecting fat absorption, especially disorders of bile acid deficiency. Calcium and magnesium homeostasis is discussed in Chapter 33.
hydrolyzed formulas, and additives such as oligosaccharides have shown some benefit (25,26,27). Most vitamins appear to be absorbed adequately in both term and preterm infants, although fat-soluble vitamin deficiency is common in disorders affecting fat absorption, especially disorders of bile acid deficiency. Calcium and magnesium homeostasis is discussed in Chapter 33.
Gut Motility
Although nutrient assimilation is heavily dependent on the development of digestive and absorptive function, actual feeding tolerance depends greatly on the maturation of gut motility (28,29,30). Neuroblasts migrate in a cranial-to-caudal direction between weeks 5 and 12 of gestation. There is gradual maturation of gut motility throughout the fetal period and the first several years of postnatal life. In the fetus, normal propulsive motility in the gut probably does not appear until approximately 30 weeks of age. Functional recordings in the fasting state of prematurely born babies aged 28 to 37 weeks show immature ileal motility (31,32). Interdigestive phenomena, known as migrating motor complexes (MMCs), can be demonstrated by approximately 33 weeks of gestation. Motor activity in the neonatal gut differs significantly from that in adults, in that the propagation rate of the MMC is substantially slower in neonates, and the complex is not abolished by feeding as in older children.
Sucking and swallowing reflexes begin early during fetal development, but the maturation of the process is not completed until after birth. The fetus is able to swallow amniotic fluid as early as 11 to 12 weeks of gestation. Actual sucking probably does not occur until approximately 18 to 24 weeks. This type of sucking is termed nonnutritive sucking, differentiating it from the more effective nutritive sucking mechanism that develops by 34 to 35 weeks of gestation. The onset of nutritive sucking closely parallels a rapid increase in growth of the fetal stomach (33) and the acquisition of mature (34) patterns of gastric antral and small intestinal motility.
By the time a term infant is born, sucking movements are followed in an orderly progression by swallowing, esophageal peristalsis, relaxation of the lower esophageal sphincter, and relaxation of the gastric fundus. The first stage of swallowing is an involuntary reflex in both the term and preterm infants. Early introduction of oral feeds may accelerate the time from gavage to full nipple feeding (35).
Some data suggest that nonnutritive sucking may play an important role in weight gain in preterm infants (34). The mechanism of this effect may be related to maturational changes in the infant GI tract, and sucking may facilitate gastric emptying and other GI functions, primarily through stimulation of secretion of GI regulatory peptides.
Maturation of GI motility may have important implications for a number of conditions. Gastroesophageal reflux is common in both term and preterm infants and probably relates to diminished lower esophageal sphincter function or inappropriate relaxation of the lower esophageal sphincter, often in association with delayed gastric emptying. The maturation of both lower esophageal sphincter function and gastric emptying has been studied extensively, with somewhat equivocal results. Depending on the technique used to measure sphincter function, the lower esophageal sphincter tone has been shown to be either low or normal in both preterm and term infants (36); however, by 31 weeks of gestation, most infants have well-developed esophageal clearance (37). In premature infants fed isocaloric formulas, long-chain triglycerides and glucose delay gastric emptying more than do medium-chain triglycerides and glucose polymers (38).
▪ GASTROINTESTINAL HORMONES
GI peptide hormones appear to play an important role in the structural and functional development of the gut and in the control of alimentary functions. The function of a vast endocrine system is integrated with that of the enteric nervous system, which itself uses other regulatory peptides as local messengers.
Endocrine cells producing gastrin, somatostatin, motilin, and glucose-dependent insulinotropic peptide (GIP) are detectable in the fetus at 8 weeks of gestation, with gastrin- and somatostatin-producing cells being most numerous (39). By 14 weeks, all of the endocrine cell types are present in the intestinal mucosa, although the anatomic distribution is more widespread than is that seen in the adult (39). By the end of the second trimester, the distribution of gut endocrine cells resembles that of the adult (39). Peptidergic nerves are first demonstrable in the myenteric plexus at about 12 weeks of gestation, correlating with the known developmental pattern of enteric nerve plexuses (39). These enteric nerves then migrate through to the submucous plexus. By the third trimester, all of the regulatory peptide systems are well developed (40). At birth, the molecular forms of the GI regulatory peptides and their distribution in the gut are similar to those of the adult (40). Surges of gut hormones appear to be responsible for the marked growth and functional change that occur in the alimentary tract in early neonatal life.
Gastrin is an important regulator of gastric secretion and is trophic to the gastric mucosa. At birth, cord blood levels of gastrin are already four to five times higher than are those in the adult, and prefeed basal levels remain elevated for several weeks (41,42). Furthermore, gastrin levels increase in response to the first milk feed (43). After 3 to 4 weeks of life, basal gastrin levels decline, a change accompanied by development of marked elevation of levels following feeding (41,44). Gastric acid is detectable in the stomach at birth and reaches a peak in the first day or two of life (42,45). Thereafter, acid output decreases for a period of about 1 month despite the hypergastrinemia and rapid growth of the stomach. It has been suggested that the lack of responsiveness to gastrin could be as a result of a lack of receptors in the oxyntic gland mucosa. Perhaps, a more likely explanation, however, is that secretion is suppressed by an inhibitor, such as peptide YY (PYY) or neurotensin, thus enabling gastrin to stimulate growth of the gastric mucosa without hyperstimulation of acid secretion (46,47). Animal experiments have shown that glycine-extended gastrin increases colonic mucosal thickness and that gastrin overall is a potent stimulant of GI mucosal growth and epithelial cell proliferation (48).
Gastrin-releasing peptide (GRP) is produced by pulmonary neuroendocrine cells (PNECs), with highest numbers of GRP-positive cells present in fetal lung. PNEC hyperplasia is frequently associated with chronic lung diseases (49). GRP has also been found to regulate odor preference in animal models, supporting the view that the GRP is involved in attachment and social behaviors (50). GRP stimulates the release of almost all GI hormones, intestinal and pancreatic secretion, as well as growth of the GI mucosa and pancreas (51).
Basal levels of the duodenal hormone, secretin, are higher at birth than they are in adults and, during the first 3 weeks of life, a more marked postprandial response develops than is seen in the adult (52). Secretin is considered to be a major factor in triggering the neutralization of acid chyme entering the duodenum and the postnatal surge of secretin, unlike that of the other alimentary hormones, occurs even in the absence of feeding, indicating the importance of this mucosal cytoprotective function (44). Secretin also seems to have a major role in blocking the trophic effects of gastrin (53).
Cholecystokinin, released from the upper small intestine, stimulates pancreatic enzyme secretion and contracts the gallbladder. Additionally, CCK has marked trophic effects on the pancreas and appears to be responsible for regeneration after resection or acute pancreatitis (54). The observed postnatal surge of plasma CCK concentrations, therefore, may be of importance in stimulating growth of this organ (55). CK and secretin have been shown to prevent jejunal and ileal atrophy in TPN-dependent animals (56), and CCK alone seems to induce early satiety (57).
Also released from the small intestine, motilin is a hormonal peptide with powerful motor functions. These motor functions include acceleration of gastric emptying and stimulation of the interdigestive myoelectric complexes (MMC) during the interprandial period. The motilin receptor-mediated induction of phase III of the MMC occurs by 32 weeks of gestational age. Motilin concentrations are low in cord blood, but preprandial basal concentrations show a massive postnatal surge that peaks at around 2 weeks of postnatal life (44). This peak is enhanced, but delayed, in preterm neonates. It is likely that this increase in circulating motilin concentrations is responsible for the known increase in motor activity of the gut that occurs during the neonatal period. Interdigestive motor complexes appear normal at birth in the term infant, but interdigestive cycles are incomplete in preterm neonates (58). Premature babies exhibit abnormal motor activity, with periods of motor quiescence and nonpropagating contractions. Thus, motor activity is more immature in preterm infants than in term infants (58). The relationship between maturation of the MMCs and the late postnatal surge of motilin in preterm neonates is not clear. Although the MMC is known to be stimulated by motilin, ghrelin, which is from the same peptide family as motilin, has been found to be key in the regulation of the MMC. It appears that the coordination of motilin and ghrelin is necessary to initiate phase 2 contraction of the MMC (59,60). Ghrelin is primarily expressed in the stomach and stimulates the release of growth hormone and plays a major role in regulating food intake, gastric emptying, and acid secretion (61).
The jejunal hormone, glucose-dependent insulinotropic polypeptide (GIP), is thought to be largely responsible for the postprandial increase in circulating insulin levels (62,63). Basal GIP concentrations are low at birth and increase gradually throughout the 1st month of life, together with the development of a marked postfeeding GIP response similar to that seen in the adult after ingestion of a mixed meal (44,64).
Neurotensin is an ileal peptide that has inhibitory effects on gastric secretion and motility but stimulates pancreatic and biliary secretion (65). Plasma neurotensin concentrations are higher in the neonate than in the adult, and an enhanced postprandial response develops in the 1st month of life (66). Both reduction of gastric secretion and slowing of the rate of gastric emptying will decrease the rate at which acid chyme enters the duodenum and, therefore, will result in a more steady absorption of nutrients from the gut. Thus, neurotensin may be important in the adaptation of the neonate to enteral nutrition. PYY is an important hormone from the distal intestine that inhibits gastric emptying and slows small bowel transit (67). PYY also inhibits gastric and small bowel secretion, leading to an increase in net absorption (46). Concentrations of PYY are elevated in cord blood and rise postnatally to a peak within the first 2 weeks postpartum (47). Preterm infants have higher levels of PYY and ghrelin than do full-term infants, and both these concentrations were negatively correlated with body weight, with the degree of correlation varying with age (68,69). There is evidence to suggest that gastric emptying and intestinal transit are rapid during the 1st week of life, both in term and preterm infants. The triggering mechanism for the changes that then take place is unknown, but it is likely that factors such as PYY play a role (67). Additionally, the very potent inhibitory effect of PYY on gastric secretion may account for the prevention of hypersecretion of acid during the early neonatal period, despite the marked hypergastrinemia (46). Both serum PYY and ghrelin concentrations have been found to reflect a negative energy balance, predict postnatal growth, and enable compensation (70).
Enteroglucagon is one of three biologically active peptides produced by posttranslational processing of the glucagon gene product in the small intestine and large intestine. Glucagon-like peptides I and II (GLP-I and GLP-II) are the two other peptides secreted in parallel with enteroglucagon, which can serve as a marker for production of all three. GLP-I has incretin effects and physiologically enhances insulin secretion in response to ingested nutrients in the same manner as GIP and is also a satiety hormone. GLP-II, on the other hand, is a trophic peptide that increases growth of the small intestinal mucosa (71,72). Plasma enteroglucagon concentrations show a very marked postnatal surge, which peaks within the 1st week and is associated with the development of a marked postprandial response (41,44). Because an increased rate of small intestinal growth occurs in the early neonatal period, it is likely that GLP-II is important in neonatal alimentary maturation (73). The resulting mucosal growth increases the absorptive area for the uptake of nutrients from the gut lumen. Somatostatin (SST) is a regulatory peptide that functions to repress the release of all GI hormones, decreases acid and pancreatic secretion, small bowel motility, and reduces splanchnic and portal blood flow (74). A synthetic analog, octreotide, has been used for patients with acromegaly to suppress pituitary hormone hypersecretion and has been found to be in higher levels in sick term and preterm infants as compared to healthy control infants (75).
Temporally, the postnatal surges of gut hormones parallel the changes in GI function that accompany the introduction of enteral feeding in the infant. Infants who have never received enteral feeding nor nonnutritive suckling do not experience these increased levels (44,76). Concentrations of all gut hormones, with the exception of secretin, remain low in infants receiving only parenteral nutrition (44,76).
Precise mechanisms control the secretion of each gut hormone, and the amount of a particular peptide liberated by a meal is adequate to stimulate the appropriate digestive response (77). The gut endocrine system, with its sparse distribution of overlapping cell types, is designed to produce an integrated digestive response to the discontinuous stimulation of ingested food (77). Because the type of food presented can influence the integrated hormonal response, it is apparent that differences in nutrition in early neonatal life may result in changes in the growth and functional development of the neonatal alimentary tract. It is likely that failure of secretion of trophic gut hormones is responsible for the hypoplastic gut and pancreas that accompany parenteral nutrition. Appropriate enteral stimuli or hormone replacement eventually may alleviate this problem. Indeed, early minimal enteral feeding, sufficient to stimulate hormonal surges, appears to have beneficial effects without any GI complications.
▪ ABNORMALITIES OF THE GASTROINTESTINAL TRACT
To avoid repetition, an attempt has been made to confine the abnormalities described in this section to those that might require consultation by a pediatric gastroenterologist. Some overlap with general surgery has been allowed, however, to avoid extensive cross-referencing (see Chapter 41).
Abdominal Wall Defects
The incidence of major defects of the abdominal wall, including omphalocele and gastroschisis, vary widely (78,79) with a predominance of gastroschisis cases. Omphalocele is a failure of the extraembryonic intestine to reenter the abdominal cavity through the umbilicus, a developmental anomaly that occurs between weeks 10 and 12 of gestational age. The defect includes the umbilicus, and the viscera typically are covered with a peritoneal sac. Occasionally, the sac may rupture, making the disorder difficult to distinguish clinically from gastroschisis. Omphalocele is often associated with other congenital anomalies or syndromes. Genetic evaluation of patients with omphalocele may provide information regarding causation or recurrence risk. Periconceptional multivitamin use may reduce the risk of nonsyndromic omphalocele (80,81).
Gastroschisis is an actual defect in the abdominal wall that occurs lateral to the umbilicus. The infant with gastroschisis has a normal umbilical cord not involved in the defect. The defect usually occurs to the right of the umbilical cord. Both omphalocele and gastroschisis may occur in the presence of other intestinal anomalies. Malrotation is present in association with omphalocele. Although intestinal atresias more commonly are found in gastroschisis, atresias may be associated with either anomaly. Abdominal wall defects frequently are diagnosed prenatally by fetal ultrasound (82). The preferred route of delivery remains controversial and may depend on the size of the defect (83). Gastroschisis or omphalocele requires immediate pediatric surgical consultation. Fluid losses and hypothermia are of primary immediate concern, especially in the case of gastroschisis because no membrane covers the bowel. Large fluid and heat losses are common, and intravenous fluid replacement should be initiated immediately. The defect should be wrapped, using warm, sterile, moist saline gauze or a sterile transparent plastic bag. Care must be taken to prevent twisting and infarction of the bowel. Positioning of the infant on the right side avoids kinking of the mesentery (84). A nasogastric tube should be inserted to minimize intestinal distention.
Immediate primary closure generally results in a shorter length of stay and less need for lengthy specialized nutrition (85). Staged reduction lasting 1 to 14 days is advocated as a method to avoid ischemic risk to the bowel. Patients with gastroschisis may demonstrate sluggish motility for up to 8 months, and a protracted course of parenteral nutrition commonly is required. Delayed onset of necrotizing enterocolitis (NEC) is not uncommon and should be suspected if bloody stools are observed.
Disorders of the Esophagus
Gastroesophageal Reflux
Gastroesophageal reflux is the most common esophageal disorder in the neonatal period (86). Gastric contents normally are retained within the stomach through the action of the lower esophageal sphincter, a zone of high pressure in the distal esophagus that remains tonically contracted except during deglutition (87). The anatomy of the stomach and esophagus, and their relationship to the diaphragm and related structures, may play a secondary role in retaining gastric contents within the stomach. Although considerable controversy exists, there is evidence to suggest that the lower esophageal sphincter may be fully functional in the normal fullterm infant. Some evidence suggests that sphincter pressure may be decreased, either continuously or intermittently, in infants with gastroesophageal reflux, facilitating reflux of gastric contents into the esophagus. There is considerable controversy over the incidence of reflux in the premature infant. Reflux appears relatively more common, but some data suggest that the lower esophageal sphincter may be competent. Delayed gastric emptying and other motility problems also may play a role in reflux in premature infants.
In adults and older children, chronic esophagitis as a result of reflux of acid into the distal esophagus is the major concern with gastroesophageal reflux. During the neonatal period, however, esophagitis rarely occurs. Reflux typically presents with continual regurgitation and spitting up or vomiting of small quantities of formula after eating. The association between reflux and apnea of prematurity remains controversial (88,89,90). Recurrent aspiration during reflux episodes may occasionally result in pneumonitis or exacerbation of preexisting neonatal pulmonary disease. If enough formula is regurgitated, the infant may fail to thrive. In neonates, reflux also may be associated with delayed gastric emptying (91). Delayed gastric antral distention occurs in some very premature infants in the early postnatal period (92). Such delays in antral distention could contribute to gastroesophageal reflux and feeding intolerance commonly seen in premature infants less than 32 weeks’ gestation. As in older children, reflux is encountered more frequently in infants with neurologic abnormalities.
Gastroesophageal reflux may exist as a primary disorder as a result of lower esophageal sphincter incompetence or intermittent relaxation, or it may be a manifestation of another disorder. First of all, it must be realized that gastroesophageal reflux may occur physiologically in all infants, although not with the frequency and severity of pathologic reflux. Any disorder that limits gastric emptying or causes a partial proximal small intestinal obstruction, such as annular pancreas or pyloric stenosis, will result in some gastroesophageal reflux. Small bowel disorders, including food protein-induced enterocolitis or infectious enteritis, will cause vomiting and regurgitation—in essence, gastroesophageal reflux. Finally, a variety of systemic disorders, including certain inborn errors of metabolism, chronic infection, chronic renal disease, and increased intracranial pressure, may result in chronic emesis similar to gastroesophageal reflux. Drugs, such as xanthines, which may be given because of apnea or lung disease, decrease lower esophageal sphincter pressure and may exacerbate or even cause reflux.
Several diagnostic studies are available to diagnose gastroesophageal reflux in infants; however, these studies as a rule do not separate primary from secondary causes. For example, an infant with milk protein enterocolitis or pyloric stenosis will have a positive test result for gastroesophageal reflux by any of the available studies. The most widely available test for reflux is an upper GI series, which is preferable to a barium swallow, because the latter only examines esophageal motility. The stomach must be filled with barium to assess the patient accurately for reflux. Unfortunately, assessing a child for gastroesophageal reflux radiographically lacks sensitivity because of the short time interval during which the child is observed, and it lacks specificity because of the likelihood of physiologic reflux occurring during performance of an upper GI series. Therefore, the primary role of an upper GI series is to exclude gastric outlet lesions, such as pyloric stenosis, or proximal small bowel partial obstructions, such as duodenal webs or annular pancreas.
Monitoring of pH was considered the gold standard through which episodes of acidity can be detected. However, the majority of GER occurs in the physiologic esophageal pH range, which is not detectable with the pH probe. Intraluminal impedance is a new method for pH-independent detection of esophageal bolus movement (91,93). The infant must be bolus fed during the study, to ensure adequate gastric distention to simulate the physiologic state. Considerable controversy exists over appropriate feeding for children during 24-hour pH monitoring. The inconsistency of acid secretion in small infants makes the procedure much less reliable during the neonatal period and, consequently, simultaneous measurement of intragastric pH often is helpful in determining the validity of the study.
A gastric emptying study is not useful to diagnose gastroesophageal reflux, but if surgery is being considered, it may be helpful in determining whether poor emptying is a contributory factor (94). Endoscopy with biopsy is a useful technique for detecting reflux in older infants; however, endoscopic biopsies are less useful during the neonatal period because pathologic reflux has not had sufficient time to cause esophageal mucosal injury. In older children, the presence of intraepithelial eosinophils suggests reflux or allergy, but this sign cannot be relied on in neonates, as biopsy specimens frequently are normal. The presence of eosinophils in the upper GI tract is strongly associated with allergic disease of the gut, especially if present in the esophagus in large numbers. Most such cases, however, appear later in childhood.
Treatment of gastroesophageal reflux is based on the severity of symptoms. If the infant is thriving well and the major complaint is frequent regurgitation and spitting, positioning on an incline at approximately 30 degrees with the head higher than the feet is beneficial. If the volume of reflux is severe and the infant is chronically irritable, has evidence of esophagitis, or is failing to thrive, then inhibition of gastric acid secretion may be necessary. Both histamine (2) receptor antagonists and proton pump inhibitors are available in liquid or powder preparations, and while they decrease
esophageal acidity, overall do not decrease the symptoms (95). Data suggest that aluminum antacids may elevate serum aluminum levels in small infants (96). Suppression of gastric acidity may actually be harmful as it may increases GI colonization of harmful microorganisms. Additionally, there is more and more evidence that use of aggressive acid suppression not only in infants but in older children and adults as well is associated with an increased incidence of GI and respiratory infections as well as Clostridium difficile infection (97,98,99,100,101,102,103).
esophageal acidity, overall do not decrease the symptoms (95). Data suggest that aluminum antacids may elevate serum aluminum levels in small infants (96). Suppression of gastric acidity may actually be harmful as it may increases GI colonization of harmful microorganisms. Additionally, there is more and more evidence that use of aggressive acid suppression not only in infants but in older children and adults as well is associated with an increased incidence of GI and respiratory infections as well as Clostridium difficile infection (97,98,99,100,101,102,103).
The effectiveness of metoclopramide is controversial, and it probably is most helpful when delayed gastric emptying coexists with reflux. Studies have shown the need for higher doses in preterm infants, which is also associated with increased side effects (104) such as irreversible tardive dyskinesia (105). Other motility agents such as cisapride and domperidone have demonstrated efficacy in adults, however, are not approved for use in the United States due to side effects such as prolongation of the QT interval (104,105). Lowto-intermediate dose erythromycin has demonstrated some benefits for improving feeding intolerance among preterm infants (106,107,108).
Transpyloric feedings have been proposed as an option for GER symptoms (109); however, in many instances, it is also associated with more adverse events (110). Some clinicians thicken infants’ formula with cereal or other thickening agents. Although this may reduce spitting, it usually does not reduce reflux or its complications and results in nutrient imbalance in the infant’s carefully formulated diet. Infant formulas containing rice starch as part of the carbohydrate component have been shown to have a modest beneficial effect in gastroesophageal reflux in infants. They have a distinct advantage over addition of rice cereal in that they maintain an appropriate macronutrient balance. It is important to remember that many of these formulas are 80% casein and 20% whey cow milk-based formulas, which increase the risk for formation of lactobezoars, especially in preterm infants, so in general they should be utilized with caution in this age group.
Gastroesophageal reflux may be treated successfully at any age with surgical fundoplication in approximately 95% of cases. The most common surgical procedures include the Nissen fundoplication, in which the stomach is wrapped and sutured 360 degrees around the distal esophagus, and the Thal fundoplication, which consists of a 270-degree wrap. Complications, including gaseous distention of the stomach and dumping syndrome, may be less common with the Thal procedure. Many surgeons now perform fundoplications laparoscopically. Indications for an operation for gastroesophageal reflux include recurrent aspiration pneumonia, failure to thrive secondary to severe vomiting unresponsive to in-hospital medical management, or apparent life-threatening apnea events associated with gastroesophageal reflux (111). Such procedures may be performed laparoscopically by competently trained pediatric surgeons.
Differential diagnosis of the typical neonate with chronic recurrent vomiting includes, in addition to gastroesophageal reflux, two major categories of disease. The first is upper GI anomalies, including pyloric stenosis. Virtually, all of these can be detected by upper GI contrast studies; pyloric stenosis can be excluded adequately by ultrasonography in the hands of an experienced pediatric ultrasonographer. The second major diagnostic category is breast milk or formula protein intolerance (112,113). These infants commonly vomit, especially those with significant small bowel mucosal disease. Such infants often are irritable and usually have loose, occult blood-positive stools. Flexible sigmoidoscopic examination of the rectum and sigmoid colon frequently demonstrates patchy colitis, often with lymphoid hyperplasia and eosinophilic infiltrates. This disorder is discussed in detail later in this chapter.
Esophageal Anomalies
The other major category of esophageal disease that presents in the neonatal period is tracheoesophageal fistula or esophageal atresia (114). These anomalies occur in approximately 1 in 4,000 live births. In addition to a prenatal history of polyhydramnios, increased salivation with coughing, choking, and cyanosis shortly after birth should raise the suspicion of tracheoesophageal fistula-esophageal atresia. The most common variety is that of atresia with the distal esophageal pouch connected to the trachea through a fistula. Such infants frequently have a stomach distended with air and respiratory symptoms as a result of tracheal aspiration of refluxed gastric acid. Esophageal stenosis is less common but both require immediate pediatric surgical consultation (115) (see Chapter 41). Vascular rings and pulmonary slings are congenital anomalies of the aortic arch and pulmonary arteries that commonly present with respiratory and/or feeding difficulties early during infancy or childhood (116).
After surgery, gastroesophageal reflux is a virtual certainty. Patients with tracheoesophageal fistula or esophageal atresia have incompetent lower esophageal sphincter function and aperistaltic contractions in the midesophagus. Although swallowing usually proceeds without much difficulty, gastroesophageal reflux with chronic esophagitis and occasionally stricture formation are frequent long-term complications. Subsequent esophageal dilatations and fundoplication may be necessary.
Disorders of the Stomach
Congenital Anomalies
Congenital anomalies of the upper GI tract frequently present with vomiting. The most common is pyloric stenosis, which occurs in approximately 1 in every 500 live births (117,118). The disease is most common in Caucasian males. A positive family history often is present. Pyloric stenosis usually presents with nonbilious projectile vomiting during the 3rd to 4th week of life. The disorder often is insidious in onset. After emesis, infants are hungry and will attempt to eat to compensate for malnutrition. It is now clear that pyloric stenosis is caused by the selective inadequate development of inhibitory neurons in the myenteric plexus of the pyloric region that utilize vasoactive intestinal polypeptide and nitric oxide as neurotransmitters to relax the sphincter. A new genome-wide significant locus for idiopathic hypertrophic pyloric stenosis at chromosome 11q23.3 has been identified and was correlated with single nucleotide polymorphism (SNP) previously found to be associated with lower levels of circulating cholesterol. Researchers have proposed that for these SNPs, the cholesterollowering allele was associated with an increased risk of IHPS and warrants further investigation (119). Eventually, nutrition deteriorates and infants become dehydrated and alkalotic secondary to chronic vomiting of the acidic gastric contents. Unconjugated hyperbilirubinemia is present in a small percentage. Patients with pyloric stenosis have normal or firm stools, in contrast to infants with formula protein intolerance, who usually have loose stools with evidence of malabsorption, inflammation, or both. Serum electrolytes reveal potassium and chloride deficiency and metabolic alkalosis. Physical examination demonstrates visible peristalsis in the epigastric region. Careful palpation of the abdomen while feeding may reveal a pyloric olive. The olive can be felt best when the stomach is empty, particularly just after vomiting. Diagnosis usually is confirmed by ultrasonography before proceeding with surgical intervention (120). Correction of electrolyte balance and the use of minimally invasive surgery result in corrective success regardless of the surgical technique with little morbidity or mortality (121). Pyloric stenosis has been noted to recur in rare cases. Nonoperative medical management of pyloric stenosis, consisting of anticholinergic drugs and small frequent feedings, occasionally may be helpful (122). This therapy, sometimes used in Europe, is rarely used in North America because of the excellent results of surgical intervention.
Other rare gastric anomalies also may present in the neonatal period. Various forms of gastric atresia or hypoplasia have been described, most of which present with vomiting at or shortly after
birth. Congenital microgastria may occur in association with a variety of other anomalies, including limb abnormalities, asplenia, megaesophagus, situs inversus, midgut malrotation, and cardiac anomalies. After major reconstructive surgery of the stomach, prognosis may be quite good. Rarely, gastric duplication cysts, gastric strictures, gastric volvulus due to diaphragmatic hernia, and gastric pneumatosis in association with necrotizing enterocolitis may occur requiring surgical intervention (123,124,125,126). Fetal ascites is an uncommon abnormality with significant fetal and neonatal mortality, particularly when the ascites develops before 24 weeks of gestation (127). Intrauterine management has led to a decrease in morbidity as ultimate management is dependent on the cause.
birth. Congenital microgastria may occur in association with a variety of other anomalies, including limb abnormalities, asplenia, megaesophagus, situs inversus, midgut malrotation, and cardiac anomalies. After major reconstructive surgery of the stomach, prognosis may be quite good. Rarely, gastric duplication cysts, gastric strictures, gastric volvulus due to diaphragmatic hernia, and gastric pneumatosis in association with necrotizing enterocolitis may occur requiring surgical intervention (123,124,125,126). Fetal ascites is an uncommon abnormality with significant fetal and neonatal mortality, particularly when the ascites develops before 24 weeks of gestation (127). Intrauterine management has led to a decrease in morbidity as ultimate management is dependent on the cause.
Acid Peptic Disease
Acid peptic disease may be seen in newborn infants (128,129,130). Ulcers in children occur most commonly in the neonatal period or during the second decade of life. In newborn infants, ulcers, whether gastric or duodenal, usually present with hematemesis. Occasionally, blood loss may be substantial, manifested by symptoms of hypovolemia and shock. Differential diagnosis of hematemesis in the newborn includes swallowed maternal blood or blood ingested from a cracked nipple through breastfeeding. Detection of swallowed maternal blood can be determined by various laboratory methods.
Diagnosis of peptic ulcer disease in the neonate requires endoscopy. Radiographic studies rarely are useful because the lesions are quite superficial and difficult to image radiographically. Endoscopy can be performed easily in a newborn infant by a skilled pediatric endoscopist using appropriate equipment. The smallest pediatric upper GI endoscopes can be used safely in term infants under conscious sedation. The minimum size of the infant in whom endoscopy can be performed safely varies with the skill of the endoscopist, but endoscopy often also can be performed safely in larger premature babies. A bronchoscope can be used in smaller infants, although the examination usually is unsatisfactory. Neonatal upper GI endoscopes are now available, which facilitate diagnosis significantly. Although ulcerations may be identified anywhere in the stomach and the duodenum, multiple superficial gastric lesions are most common in newborn infants. Ulcers may be primary, or they may be secondary, as in the case of drugs known to irritate the upper GI tract, such as steroids or theophylline. Treatment with antacids, or preferably H1-receptor antagonists such as cimetidine or ranitidine, for a period of 2 to 6 weeks results in complete healing of the lesion (131). Secondary ulcers may be treated in a similar fashion. Use of proton pump inhibitors (PPIs) may be less efficacious as neonates lack specific cytochrome P-450 enzymes required to handle PPIs until 6 months of age (132). In this instance, continuation of the offending agent requires careful assessment of the risk-to-benefit ratio, because lesions will heal more rapidly if the agents are discontinued. Symptomatic neonatal infection with Helicobacter pylori is a rare phenomenon (133); however, its appearance has been identified in developing countries, and postulations of maternal transmission and other factors have been identified to increase its appearance (134,135,136,137).
Spontaneous gastric perforation is a rare occurrence in the newborn. It occurs most commonly during the first 5 days of life, especially in infants subjected to severe stress or hypoxia (138,139). The constellation of symptoms typically includes a sudden deterioration in clinical status between the 2nd and 5th day of life, characterized by refusal to eat, vomiting, abdominal distention, and respiratory distress. Free intraperitoneal air (usually a large volume) and fluid are demonstrable on plain radiographs of the abdomen. Immediate surgical consultation should be sought.
Disorders of the Small Intestine
Congenital Anomalies
Of the various small intestinal disorders that present in the neonatal period, congenital anomalies that produce obstruction are likely to present earliest. Patients present with bilious vomiting, abdominal distention, and occasionally obstipation. Bilious vomiting indicates obstruction distal to the ampulla of Vater. Bilious vomiting associated with the passage of blood through the rectum suggests vascular compromise of the small intestine, necessitating immediate surgical intervention. Malrotation or nonrotation of the gut is an anatomic defect produced by incomplete rotation and fixation of the embryonic intestine after return from its extraabdominal location at about week 10 of gestation (140
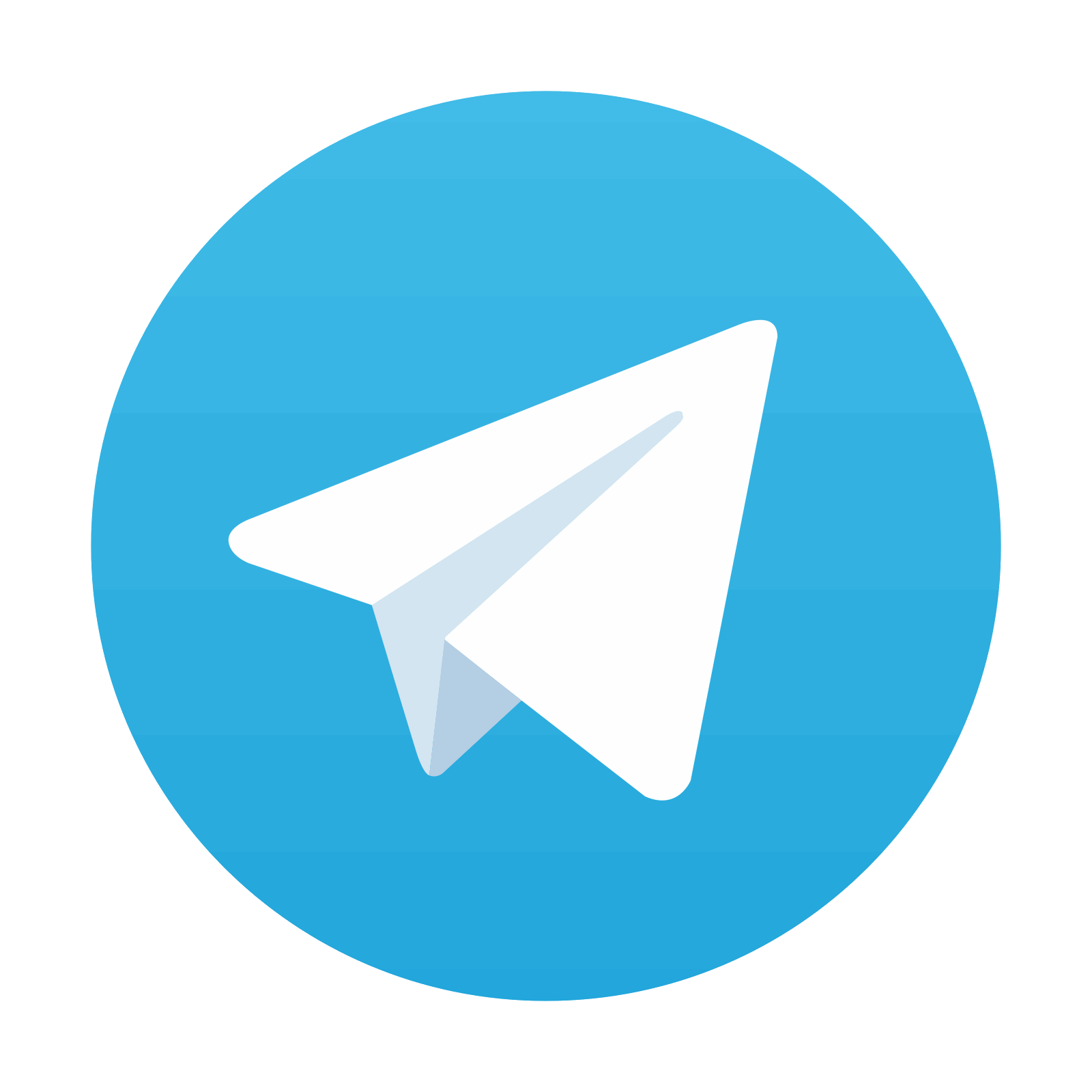
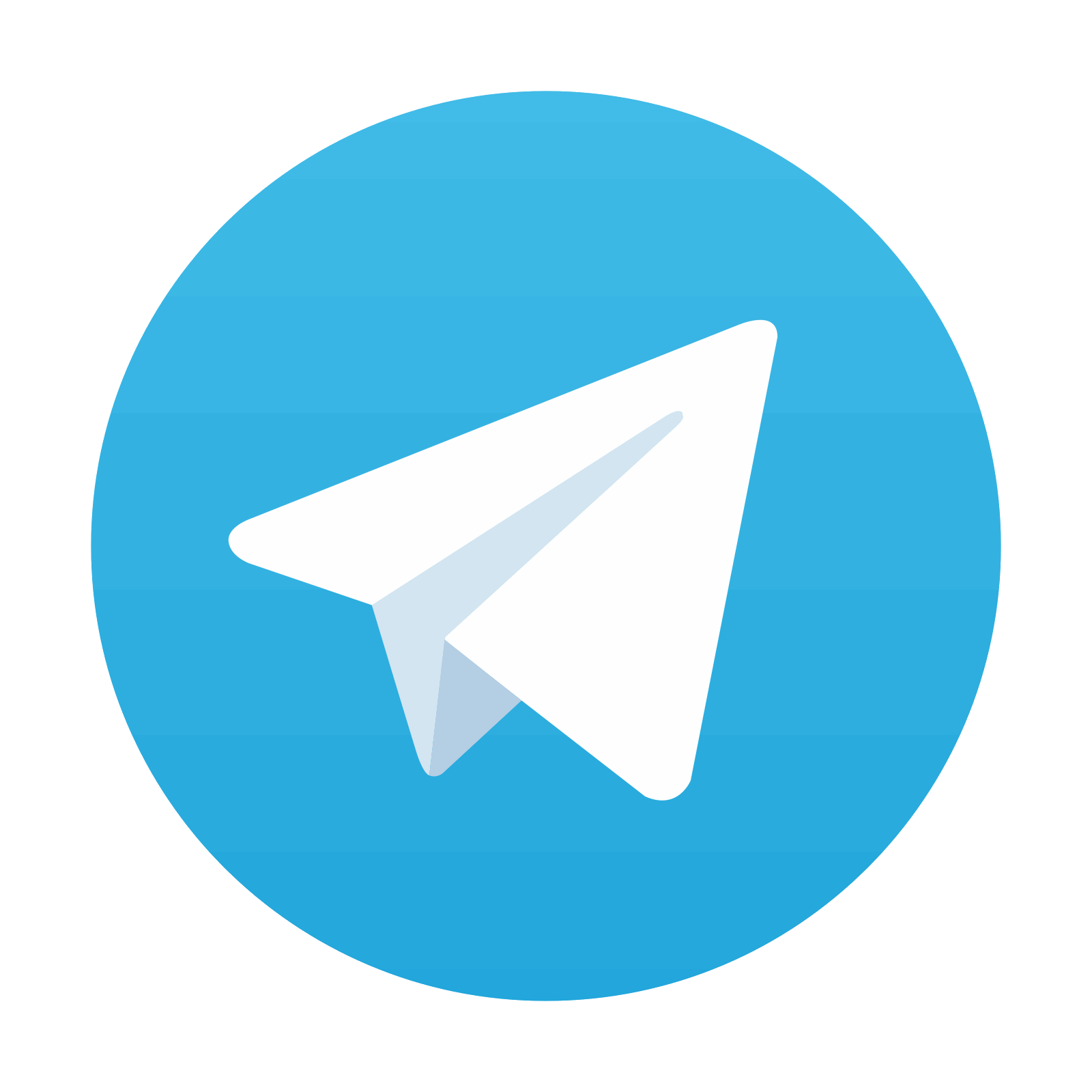
Stay updated, free articles. Join our Telegram channel

Full access? Get Clinical Tree
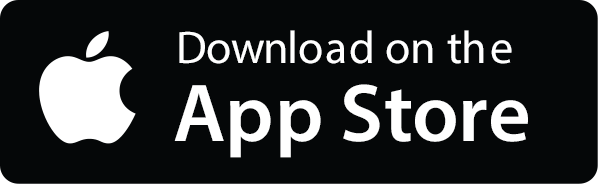
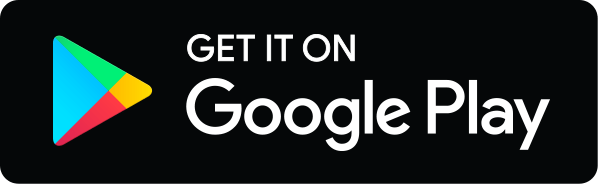
