Fetal Imaging: Ultrasound and Magnetic Resonance Imaging
Jennifer H. Johnston
The use of two-dimensional ultrasound (2D-US) in prenatal imaging has revolutionized antenatal surveillance and detection of structural anomalies in the fetus. Data obtained from fetal imaging are crucial for management throughout the duration of pregnancy, guiding clinical decision making and counseling as well as potential fetal interventions. Advanced imaging techniques such as three-dimensional ultrasound (3D-US) and ultrafast magnetic resonance imaging (MRI) are often valuable adjuncts to conventional US.
▪ ANTENATAL SONOGRAPHIC ASSESSMENT
Prenatal sonographic examinations may be classified as first trimester, standard second or third trimester, limited, or specialized. A first-trimester US may be performed to document fetal viability, establish gestational age, and ascertain the number of fetuses. Screening for aneuploidy can be performed, and some gross fetal structural abnormalities may be detectable. A standard second- or third-trimester examination includes fetal biometry and an anatomic survey. Limited examinations are performed to answer a specific question, for instance, to confirm fetal viability or determine fetal lie. Specialized examinations are done when fetal anomalies are suspected and include studies such as fetal Doppler US and fetal echocardiography.
During the first trimester, scanning can be performed transabdominally or transvaginally. Although the transabdominal approach allows for a better overview of the uterus and adnexa, the transvaginal approach affords superior resolution.
▪ ESTIMATION OF FETAL AGE
Accurate dating is critical for optimal pregnancy management, providing a benchmark by which to compare fetal growth throughout gestation and allowing for estimation of the date of delivery. Dating with US has proven to be a reliable method (1,2). Parameters used for age estimation should be easy to obtain, reproducible, and accurate. Many dating charts are available to correlate size of fetal structures with gestational age. Variability in age estimates increases as term approaches, and therefore gestational age should be established as early as possible.
![]() FIGURE 12.1 Fetal biparietal diameter (BPD). This measurement is obtained on an axial image of the fetal head at the level of the thalami (asterisk) and cavum septum pellucidum (arrow). |
A gestational sac may be detected by 5 weeks transabdominally but earlier with transvaginal scanning. An embryo is typically seen 1 week afterward. Although gestational age may be estimated from mean gestational sac diameter, the crown-rump length should be used once the embryo is visible as it is the most accurate method for estimating gestation age (3).
Beyond 14 weeks of gestation, other biometric parameters are used in combination to estimate gestational age. The biparietal diameter (BPD) correlates well with gestational age and is measured from the outer edge of the proximal parietal bone to the inner edge of the distal parietal bone on a standardized axial view of the skull (Fig. 12.1). Measurement of BPD in the early second trimester has a precision of ±1.4 weeks (4), but accuracy is diminished by abnormal skull shape, for instance due to cranial molding. In these cases, a cephalic index can be calculated by measuring the long and short axes of the skull. If this index falls outside the normal range of 70 to 86, fetal head circumference (HC) may be used as an alternative since it is independent of shape. HC is measured around the outer surface of the calvarium, at the same level as BPD.
The femur is the most commonly measured long bone due to its relatively large size and visibility. Femur length (FL) is measured from the proximal metaphysis to the distal metaphysis on an image aligned perpendicular to the femur (Fig. 12.2). Although less accurate than BPD and HC in the second trimester, FL is considered to be an accurate predictor of age during the third trimester and may be used alone to assign age if head parameters are unreliable due to pathology. FL is also an important parameter of limb growth for assessment of skeletal dysplasia.
Abdominal circumference (AC) is obtained in a transverse image containing the umbilical vein at the level of the portal sinus by tracing along the outer edge of the soft tissues. AC is considered less accurate than are BPD, HC, and FL for gestational age estimation and is the most difficult of the four to measure accurately. It is, however, the most useful parameter for calculation of fetal weight and assessment of fetal growth.
These parameters—BPD, HC, FL, and AC—become less accurate in predicting age as gestation progresses (4). The most accurate
estimation of age is usually achieved by using a combination of measurements as opposed to relying on a single measurement.
estimation of age is usually achieved by using a combination of measurements as opposed to relying on a single measurement.
▪ ASSESSMENT OF FETAL WEIGHT
Estimated fetal weight (EFW) is derived from formulas utilizing the same biometric parameters used for age. Numerous formulas have been studied for this purpose, some of which use a single variable such as BPD or AC, but accuracy is improved when more than one parameter is incorporated (5). The two most commonly used are the Shepard formula (which uses BPD and AC) and the Hadlock formula (which uses FL and AC) (6,7). Calculated EFW may then be compared to published nomograms for fetal weight percentiles. It is important to note that error in determining EFW is significant even when utilizing several biometric parameters, ranging between ±10% and 20%, and tending to increase with fetal size (5,8).
▪ ASSESSMENT OF FETAL GROWTH
It is believed that each fetus has an inherent growth potential, which will be achieved if the pregnancy progresses normally (8). Growth occurs most rapidly in the second trimester and then decelerates until term. Calculated EFW can be compared to the range of expected weight for a particular gestational age or be plotted onto standard curves based on population average. Additionally, customized growth curves are available that adjust for variables that affect fetal growth, such as fetal sex and maternal height, weight, and parity; this approach may provide some benefits, but further study is required (9). Serial US measurement of fetal weight is another method to assess fetal growth. The interval between US examinations for fetal growth should be no shorter than 2 to 4 weeks since too short a time period may yield apparent differences in weight that are due simply to measurement errors (3).
Intrauterine Growth Restriction
Intrauterine growth restriction (IUGR) refers to growth less than expected for gestational age and is most often defined as EFW under 10th percentile for age. The term IUGR implies underlying pathology, which can be divided into fetal (chromosomal abnormalities, infection), maternal (hypertension, smoking), and placental problems. This is in contrast to the fetus that is small for gestational age (SGA). The latter carries a good prognosis and describes a fetus that is small but has reached its expected genetic growth potential and, after birth, will be well proportioned without other abnormalities.
IUGR may be categorized as symmetric or asymmetric. Symmetric IUGR refers to a fetus that is uniformly small and is typically detected earlier in gestation. Causes of symmetric growth restriction include chromosomal abnormalities, multifactorial congenital malformations, and in utero infection. More common is asymmetric IUGR, which manifests as a small AC in relation to the remainder of the fetus and implies a placental or maternal abnormality. The fetus, receiving less than the required blood supply, preferentially shunts blood to vital organs such as the brain at the expense of the liver, muscle, and fat. If the insult continues, eventually the entire fetus becomes small as it loses the ability to compensate and assumes the appearance of “symmetric” IUGR. This can be differentiated from true symmetric IUGR by history and often by the presence of oligohydramnios, which is a result of chronic stress on the fetus. In contrast, symmetric IUGR is typically accompanied by normal amniotic fluid volume (AFV).
As a single parameter, AC is the most sensitive predictor of IUGR; however, numerous formulas including FL or HC have been used. Slow interval growth may also indicate the presence of IUGR, a helpful measure particularly when gestational age is uncertain.
Close monitoring is recommended in the setting of IUGR. Doppler assessment of the umbilical artery may be used for this purpose and can provide clues to the etiology of IUGR (see Doppler Assessment of Blood Velocities in Umbilical and Fetal Vessels). Other monitoring methods include serial sonographic assessment of growth, nonstress testing, and biophysical profile (BPP) testing. However, antenatal surveillance regimens vary between institutions due to the paucity of randomized controlled trials on this topic (10).
Macrosomia
An infant who is macrosomic, or large for dates, is typically defined as one with birth weight >4,000 g or over the 90th percentile for gestational age. Macrosomia is most commonly associated with material diabetes, but other causes include maternal obesity and some genetic syndromes such as Beckwith-Wiedemann syndrome. Fetuses with macrosomia are at risk for shoulder dystocia and brachial plexus injury. As is the case for IUGR, AC is the best single parameter for prediction of macrosomia (11). Weight estimates derived from formulas utilizing biometric parameters in suspected macrosomic fetuses have an error of ±10% (8). Also to be taken into account are factors that affect fetal and birth weight, such as fetal gender, race, and ethnicity. Other methods have been proposed to predict fetal macrosomia, such as measuring subcutaneous fat thickness. The greater availability of 3D-US has also spurred interest in using volumetric measurements for this purpose (12).
▪ ANEUPLOIDY SCREENING (SEE ALSO CHAPTER 10)
Screening for aneuploidy is typically performed during the first trimester and includes measurement of fetal nuchal translucency (NT) thickness. NT thickness is an effective screening tool for trisomy 21 and other genetic abnormalities and is associated with cardiac defects. The pathophysiology for increased NT thickness is not well understood but has been variously attributed to cardiac dysfunction, venous congestion in the head and neck, and impaired lymphatic drainage. The nuchal region is preferably evaluated at a gestational age between 11 weeks and 13 weeks 6 days (13). Nuchal translucency should be measured according to standardized guidelines on a midline sagittal image of the fetus and appears as a sonolucent strip along the posterior neck (Fig. 12.3).
Assessment for aneuploidy risk is enhanced by incorporation of maternal serum biochemical markers. In a population with a 5% screen-positive rate, using biochemical markers in addition to NT measurement has a detection rate for trisomy 21 of approximately 87%, compared to 70% for each alone (14). Greater maternal age is associated with an increased risk of aneuploidy and should be considered. First-trimester combined screening that includes fetal NT, maternal age, and maternal biochemical markers yields a 90% detection rate (15). Other sonographic markers of aneuploidy, such as an absent nasal bone, tricuspid regurgitation, and increased resistance to flow in the ductus venosus, may increase accuracy (16). Increased flow in the fetal hepatic artery is a more recently described marker for aneuploidy (17).
▪ ASSESSMENT OF NORMAL AND ABNORMAL FETAL ANATOMY (SEE ALSO CHAPTER 10)
Congenital malformations affect approximately 3% of all live births and are a significant cause of infant mortality, resulting in about 20% of infant deaths (18,19). Early prenatal detection of anomalies is important for counseling, planning intervention, and ensuring optimal delivery room management.
Ultrasound is the imaging modality of choice for initial fetal evaluation. A survey of fetal anatomy is best performed after 18 weeks of gestation, although certain structural abnormalities can be seen before this time (3,20). Ultrasound evaluation should include the brain, skull, face, neck, lungs, heart, stomach, kidneys, urinary bladder, abdominal umbilical cord insertion, spine, extremities, and genitalia. When abnormalities are detected, more detailed sonographic assessment may be undertaken. A follow-up examination at a later gestational age may be useful as some abnormal findings may improve (e.g., congenital pulmonary airway malformations [CPAMs]) and others may only become apparent as the fetus matures (e.g., hydrocephalus, cardiac defects, and cranial signs of spina bifida). When concerning US findings are seen, amniocentesis can be performed during the second trimester to detect chromosomal abnormalities.
While 2D-US remains the primary modality for fetal imaging, 3D-US can be a valuable complementary tool. Imaging with 3D-US allows for scanning and storing of volumetric data that can be manipulated at a later time and redisplayed in any plane desired. It also allows for the creation of 3D surface-rendered images and is particularly helpful in evaluating the face, extremities, and spine and neural tube defects (NTDs) (Fig. 12.4) (21). Like 2D-US, 3D-US has the advantages of being noninvasive and safe during pregnancy. The limitations of 3D-US are generally similar to those of 2D-US but include greater sensitivity to fetal motion.
Fetal Magnetic Resonance Imaging
With the advent of ultrafast scanning sequences, MRI has become a feasible method of prenatal imaging and a useful adjunct to US. Advantages of MRI include superior soft tissue contrast, larger field of view, and fewer limitations related to the ossifying fetal calvarium, maternal body habitus, fetal lie, and low AFV (22,23).
In determining the appropriate gestational age at which fetal MRI should be performed, consideration should be given to technical factors and the clinical information being sought. Prior to 18 to 20 weeks, detail on fetal MRI can be limited by fetal motion and low resolution due to the small size of the fetus. Although MRI in the third trimester benefits from excellent resolution, a balance should be struck between early diagnosis and waiting past the point of possible fetal intervention.
Although the safety of MRI during pregnancy has not been definitely proven, studies to date have not demonstrated deleterious effects on patients who underwent MRI in utero (24,25). While some animal studies have raised some concerns with regard to potential teratogenic effects, no retrospective human studies have demonstrated adverse findings (26). Another potential safety issue is acoustic damage to the fetus due to the loud knocking noises produced during MRI scanning; however, one small study found no evidence of hearing damage (27). Long-term effects on children who underwent prenatal MRI have also not been demonstrated (27,28,29), although these studies have generally been limited by small sample sizes and short length of follow-up.
After weighing the risks and benefits, fetal MRI can be considered in situations in which it can provide information that is not obtainable by US, that will affect the care of the patient or the fetus, or which will be valuable in counseling families during clinical decision making.
Fetal Central Nervous System Abnormalities
Prenatal screening for abnormalities of the central nervous system (CNS) is typically performed by US on a routine basis or due to elevated maternal alpha-fetoprotein (AFP). Although large CNS anomalies may be visible in the first and early second trimesters, the most favorable time for sonographic evaluation of the CNS is around 20 weeks of gestation (30). Sonographic evaluation of the brain consists of three standard axial sections, with additional coronal and sagittal images as needed. Features of the CNS that can be evaluated by US include brain parenchyma, sulcation pattern, corpus callosum, posterior fossa, and calvarium.
In the presence of abnormal US findings, fetal MRI may provide useful additional information. In the CNS, advantages of MRI include greater soft tissue contrast and the absence of reverberation artifact from the calvarium, making MRI a sensitive tool for evaluating the microstructure and composition of the brain (31). Sulcation pattern is easily seen by fetal MRI and serves as a marker for brain maturity. Ventricular size and configuration are also readily evaluated. Some anatomic structures not routinely depictable by US can be identified on MRI, such as the olfactory bulb, inner ear, optic chiasm, and pituitary gland (22). Signal changes in the brain also mirror the evolving composition of the parenchyma and are due to progressive diminishment in water content, increasing cell density, and myelination (31).
Ventriculomegaly
Ventriculomegaly refers to abnormal enlargement of the ventricular system and is a relatively common abnormality detected by obstetrical US. The term hydrocephalus is used specifically in cases due to increased pressure, most commonly due to obstruction to cerebrospinal fluid flow. Ventriculomegaly may also result from abnormal ventricular development or from parenchymal destruction due to processes such as vascular insults or infection. The major causes of ventriculomegaly are aqueductal stenosis, Chiari II malformation, agenesis of the corpus callosum, Dandy-Walker malformation, and fetal aneuploidy (32).
As ventriculomegaly is one of the most common false-positive findings during US fetal anatomic evaluation, accurate sonographic assessment of the ventricular system is key. Measurement of the lateral ventricles is performed at the level of the atrium of the ventricles on an axial view of the fetal head (Fig. 12.5A) (30). Malformations (both CNS and non-CNS) are more commonly found in severe ventriculomegaly (>15 mm) with a rate ranging between 58% and 65% (33). The reported rate of malformations in less severe ventriculomegaly varies widely in the literature but is likely
higher in moderate ventriculomegaly (12.1 to 14.9 mm) than in mild ventriculomegaly (10 to 12 mm) (34). Underlying aneuploidy is also a concern, particularly when additional structural malformations are present.
higher in moderate ventriculomegaly (12.1 to 14.9 mm) than in mild ventriculomegaly (10 to 12 mm) (34). Underlying aneuploidy is also a concern, particularly when additional structural malformations are present.
Fetal MRI is a valuable adjunctive tool in cases of ventriculomegaly to evaluate for etiology and any additional abnormalities (Figs. 12.5B and 12.6). The reported percentage of cases in which MRI detects an additional abnormality not seen on US ranges between 5% and 50% (33).
The most important prognostic indicator is the presence and type of additional structural or chromosomal abnormalities. The outcome of isolated ventriculomegaly, by contrast, varies with the degree of ventricular enlargement. In mild isolated ventriculomegaly, the rates of neonatal and perinatal death and of neurodevelopmental delay are lower when compared to moderate or severe ventriculomegaly (34). A substantial cohort of patients with isolated mild-to-moderate ventriculomegaly also experiences resolution of ventricular dilatation in utero (35).
Neural Tube Defects
NTDs result from the failure of full closure of the neutral tube early in gestation. These defects are often first suspected in the face of an abnormal screening maternal serum AFP result. Prenatal US can detect spinal abnormalities, evaluate for associated CNS and non-CNS abnormalities (e.g., clubfoot), and assess fetal growth. Findings of a myelomeningocele include splayed vertebral pedicles and a sac overlying the spinal defect (Fig. 12.7). For a detailed assessment of the spinal lesion, 3D-US is useful because it allows for manipulation of imaging planes after initial acquisition and can be displayed with various rendering algorithms to highlight bony structures or overlying skin. In addition, 3D-US is especially useful in depicting the specific level at which the dysraphic defect lies. Chiari II malformation is commonly seen with an open NTD and comprises downward herniation of the cerebellar tonsils into the upper cervical canal with crowding of the posterior fossa. Intracranial findings include a compressed cisterna magna and an abnormally rounded cerebellum (banana sign), concave contour of the frontal bones (lemon sign), small BPD and HC, and ventriculomegaly (36).
Although some features of Chiari II can be recognized by US, some intracranial findings such as the degree of tonsillar herniation and tectal beaking are better depicted by MRI. Other CNS abnormalities associated with NTD and Chiari II include gray matter heterotopia, callosal dysgenesis, holoprosencephaly, cerebellar dysplasia, and diastematomyelia (37).
Fetal Chest Anomalies
Appropriate growth of the lungs requires adequate thoracic space, as well as amniotic and lung fluid to provide positive transpulmonary pressure (38). A variety of fetal chest abnormalities can be detected by US by the second trimester.
![]() FIGURE 12.7 Myelomeningocele. Sagittal T2-weighted image depicts the neural elements traversing the spinal defect and the myelomeningocele sac at the lower spinal region. |
The lungs are normally homogeneous and, compared to liver parenchyma, more echogenic on US and hyperintense on MRI. The trachea and main bronchi are depicted as fluid-filled branching tubular structures. The diaphragm can be seen as a thin hypoechoic curved line between the lungs and the abdomen on US. Mediastinal shift and heterogeneity of the lungs can be the first clue to the presence of a space-occupying lesion.
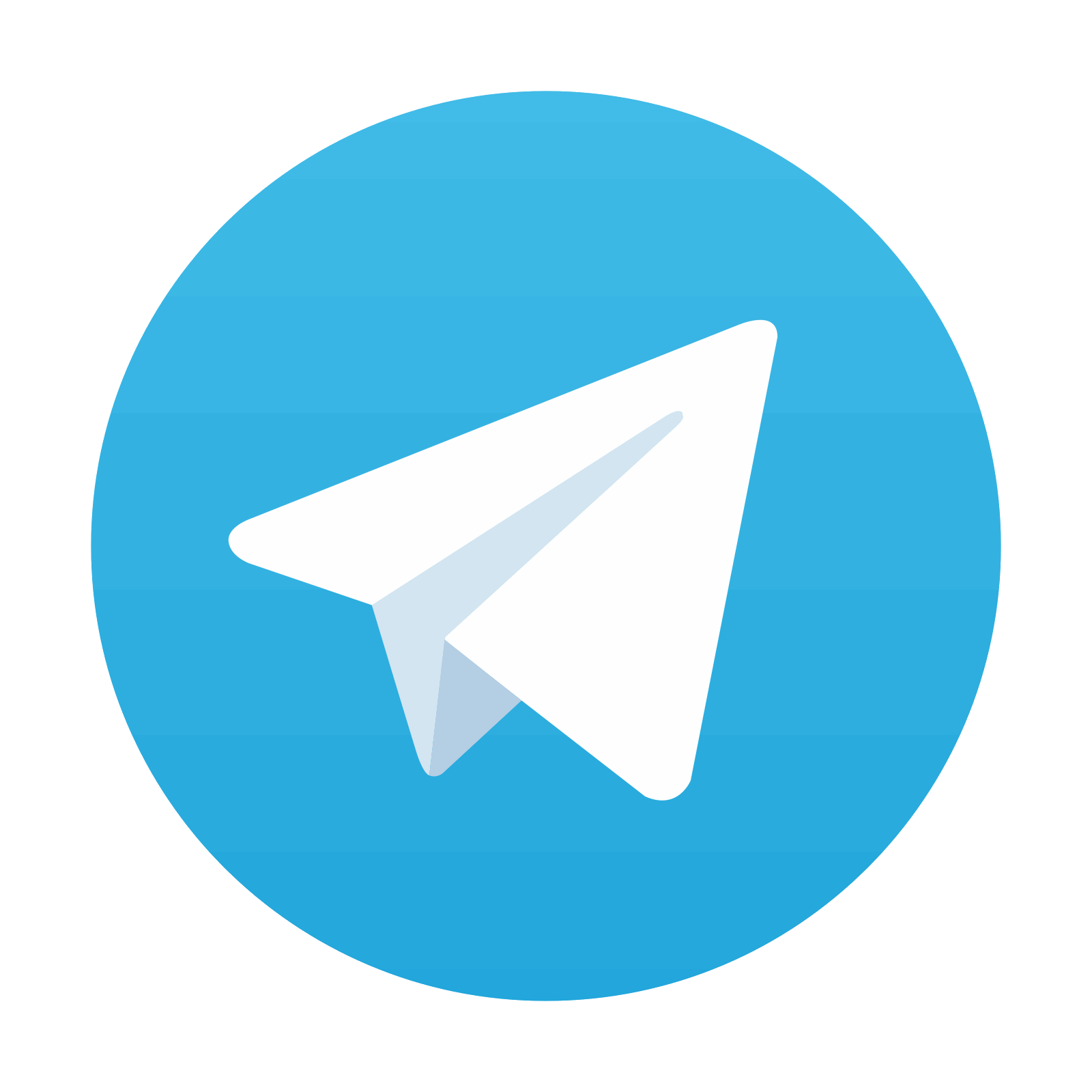
Stay updated, free articles. Join our Telegram channel

Full access? Get Clinical Tree
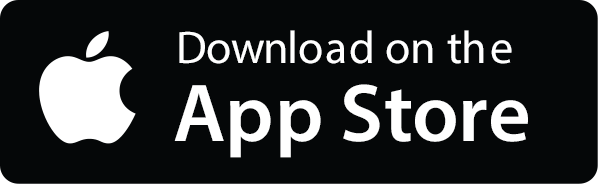
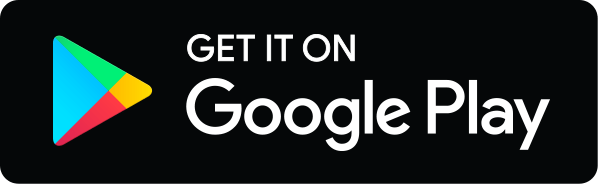