Extracorporeal Membrane Oxygenation (ECMO)
INDICATION
Extracorporeal membrane oxygenation (ECMO) is the use of prolonged extracorporeal circulation and gas exchange via a modified heart-lung machine to provide temporary life support in patients with cardiac or respiratory failure who are refractory to maximum ventilatory and medical management. ECMO allows the lungs to rest and recover while avoiding the damaging effects of aggressive mechanical ventilation, including barotrauma and oxygen toxicity. The first successful use of extracorporeal support in a newborn was reported in 1976 by Dr. Robert Bartlett.1 Subsequent data suggested that ECMO provided improved survival when compared with historical controls2,3; however, only 2 small trials with adaptive designs were performed prior to widespread use of ECMO.4,5 The UK Collaborative ECMO Trial, published in 1996, confirmed that ECMO significantly reduced mortality when compared with standard medical care (32% vs 59%, relative risk 0.55; 95% confidence interval 0.36–0.80) with improved survival in all diagnostic categories.6
The Extracorporeal Life Support Organization (ELSO), established in 1989, maintains a patient registry to collect data from more than 200 ECMO centers around the world. ELSO Registry data as of July 2013 included 27,000 newborns placed on ECMO for respiratory support.7 Approximately 800 neonatal respiratory cases are performed annually, with a cumulative survival of 75%. Review of the neonatal ELSO Registry data demonstrated ongoing demographic changes. ECMO use for neonatal respiratory failure has declined over the last 2 decades, likely related to the increasing use of high-frequency ventilation (HRV), surfactant replacement, and inhaled nitric oxide (iNO).8–10 There have also been significant changes in the diagnostic categories of those receiving ECMO. Dramatic decreases in the use of ECMO for respiratory distress syndrome and sepsis/pneumonia have occurred. There has also been a downward trend in the use of ECMO for meconium aspiration syndrome; it is no longer the most common indication for ECMO. In recent years, congenital diaphragmatic hernia (CDH) has become the most common indication for ECMO; however, the survival rate continues to decline for this and the other diagnostic categories. Most ECMO centers are treating fewer patients annually; however, the length of bypass is longer, and survival is lower. These changes challenge ECMO centers to maintain their expertise with a complex technology despite lower patient volumes.
Hypoxemic Respiratory Failure
Hypoxemic respiratory failure (HRF) frequently occurs in association with persistent pulmonary hypertension of the newborn (PPHN). PPHN is characterized by markedly elevated pulmonary vascular resistance and pulmonary arterial pressure, in conjunction with striking pulmonary vasoreactivity. This produces right-to-left shunting at the patent ductus arteriosus (PDA) and patent foramen ovale (PFO). It results in hypoxemia from extrapulmonary shunting, which can be poorly responsive to medical management. PPHN is felt to represent failure of the pulmonary circulation to adapt to postnatal conditions and as such is sometimes called “persistent fetal circulation.” The pulmonary vascular bed is abnormal, with extension of the medial smooth muscle layer into the smaller and more peripheral intra-acinar vessels. PPHN can occur in several clinical settings, described next.
Idiopathic
Idiopathic PPHN occurs when there is abnormally constricted pulmonary vasculature without parenchymal lung disease. It has been called “black lung” PPHN because of the lack of pulmonary vascular markings on a chest radiograph.
Parenchymal Lung Disease
Frequently, PPHN accompanies meconium aspiration syndrome, respiratory distress syndrome, sepsis, or pneumonia. In these settings, evidence of PPHN is seen in addition to intrapulmonary shunting from parenchymal lung disease.
Lung Hypoplasia
Lung hypoplasia can be seen with CDH and in infants with premature prolonged rupture of the membranes and long-standing oligohydramnios. In these conditions, lung growth and development are affected, and there is a variable degree of lung hypoplasia. This results in a decrease in the cross-sectional area of the pulmonary vascular bed with subsequent pulmonary hypertension and pulmonary vasoconstriction.
Rare, Fatal Conditions
Respiratory failure and PPHN can be the presenting features in surfactant protein B (SPB) deficiency, alveolar capillary dysplasia (ACD), and lymphangectasia.
Initial Treatment for HRF
1. Identify infants at risk for PPHN. Infants with meconium suctioned from below the cords should be monitored with pulse oximetry following birth.
2. Closely monitor these and other infants at risk for PPHN for signs of respiratory distress and desaturation. Umbilical artery catheter (UAC) placement should be considered earlier in the management of infants at risk of PPHN who have respiratory distress
3. Promptly treat hypoxemia and acidosis. Hypoxemia and acidosis increase pulmonary vascular resistance and should be promptly addressed.
4. Ensure adequate cardiac output. Maintain a mean arterial pressure greater than 45 mm Hg in a term or near-term infant to lessen the right-to-left shunt. This may be achieved with a combination of either volume boluses or pressors. Central arterial and venous pressure monitoring are desirable to guide fluid and pressor therapy.
5. Maintain adequate oxygen-carrying capacity by keeping the hematocrit greater than 40. Hematocrits in excess of 50 may result in increased pulmonary vascular resistance.
6. If there is evidence of pulmonary hypertension, either clinically or by echocardiography, then the suggested target arterial blood gas (ABG) values are pH greater than 7.40 and PaO2 greater than 60. PaCO2 values under 35 can decrease cerebral blood flow and increase the risk for brain injury. Consider use of cerebral near-infrared spectroscopy (NIRS) in this patient population to monitor for cerebral vasoconstriction. HFV may be considered to achieve the blood gas targets mentioned.
7. Minimize unnecessary handling and noise.
8. Ensure appropriate sedation and analgesia. Neuromuscular blockade may be considered if oxygenation remains suboptimal after other therapeutic approaches have been used, although this may exacerbate fluid retention and edema formation.
9. Calculate and follow the oxygenation index (OI) for all infants with oxygen requirement of 60% or greater:
OI = (Mean airway pressure) × (FiO2/PaO2) × 100
Maximal Medical Therapy Alternatives for HRF
High-Frequency Ventilation
High-frequency ventilation is used with the goal of decreasing ventilation-perfusion mismatch and optimizing lung inflation.11 HFV is generally considered when the OI is greater than 10.
Surfactant Therapy
Surfactant should be considered when the OI is 15 or greater. Infants with lung injury caused by parenchymal disease may have a secondary surfactant deficiency. A masked randomized trial reported a significant reduction in the need for ECMO with surfactant therapy.12 Subgroup analysis showed the greatest benefit in the group with an OI of 15–22. Consider using a test dose in unstable infants.
Inhaled Nitric Oxide Therapy
Inhaled nitric oxide therapy is a selective pulmonary vasodilator that has been shown in two prospective randomized trials13,14
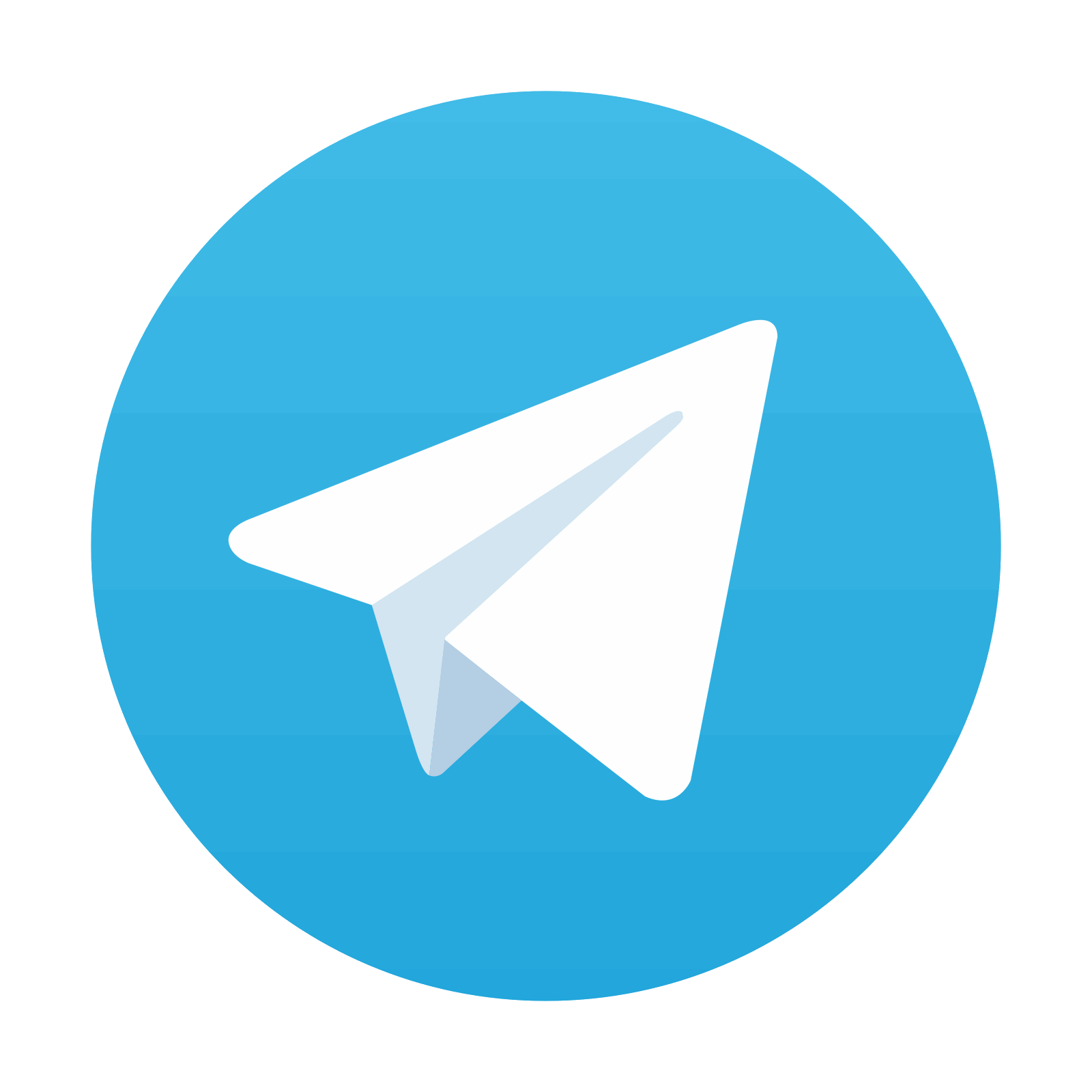
Stay updated, free articles. Join our Telegram channel

Full access? Get Clinical Tree
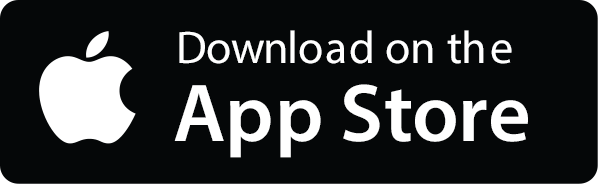
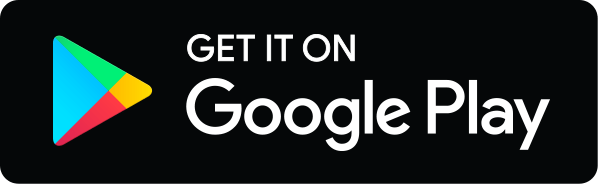