Enteral Nutrition
INTRODUCTION
The enteral route is the most physiologic and natural way of administering nutrients to humans, and attaining full enteral feedings can often be challenging to clinicians who care for preterm infants. Establishing enteral feedings should be one of the most important goals, especially in very low birth weight (VLBW) infants. The utilization of the gastrointestinal tract provides these patients with multiple benefits, which include enhanced growth and neurodevelopment, improved immunologic functions with decreased infections, and acceleration of intestinal adaptation and maturation with a subsequent increase in the absorption of nutrients. Despite the numerous advances made to date in the area of nutrition for preterm infants, such as the use of parenteral solutions with more proteins, the majority of these infants are discharged weighing less than the 10th percentile for age.1,2 The early initiation of parenteral nutritional support in preterm infants is a trend now widely supported by neonatologists, but often, enteral nutrition is underutilized, delayed, or totally withheld for prolonged times because of concerns of feeding intolerance or the fear for the development of necrotizing enterocolitis (NEC), which remains the most common devastating gastrointestinal complication of the preterm infant. The principles that should be considered to prevent “metabolic shock” and to optimize nutrition in the preterm infant should include the following:
1. Clinicians should be aware that metabolic and nutritional requirements do not stop with birth.
2. That hours, not days, are the longest periods this group of infants should be allowed to face without receiving either enteral or parenteral nutrition (PN).
3. That the metabolic and nutritional requirements of the newborn are equal to or greater than those of the fetus.
In this chapter, we review characteristics of the developing intestine as they relate to enteral nutritional strategies for the preterm infant.
The Developing Intestine
The intestine not only serves as a nutrient digestive absorptive organ but also is one of the largest immune organs of the body and serves a significant endocrine and exocrine role in humans. The intestine has neural tissue in amounts equivalent to that of the entire spinal cord. As the fetal intestine develops, the increase in its total surface area is significant, largely because of the villus and microvillus growth during this period of development.
In many neonatal intensive care units (NICUs), because of concerns of feeding intolerance and NEC, the gastrointestinal tract of the critically ill, low birth weight infant either is not exposed to nutrients at all for prolonged periods after birth or is provided only “minimal enteral nutrition,” and most nutrients are only provided parenterally. The PN solutions are lifesaving nutritional medical interventions in the preterm infant, but ideally, the approach should be to provide most of the nutrients via the enteral route as soon as safely possible to promote intestinal growth and normal physiology. As illustrated in Figure 11-1, the lack or absence of luminal nutrients will result in mucosal atrophy, a decrease in the stimulation of trophic hormones, alterations in the microbiota, increased polymorphonuclear attraction, and an increased likelihood of development of the systemic inflammatory response syndrome, translocation of intestinal microbes, and sepsis. Because of the higher nutritional requirements needed for intestinal growth during the neonatal period, the lack of luminal nutrients may likely be of even more significance in these infants than in adults.
FIGURE 11-1 Detrimental effects of parenteral nutrition (PN) use and lack of enteral nutrition. Vicious cycle of lack of feeding and perpetuation of negative effects at the intestinal, immunological, and liver function levels. IgA, immunoglobulin A; PMN, polymorphonuclear cells.
The newborn deprivation of enteral nutrients can be deleterious. These effects have been demonstrated by studies in which neonatal pigs were suckled and subsequently gained 42% of their weight in the first 24 hours after birth. In contrast, such growth did not occur in the unsuckled animals.3 Using piglets, other researchers have demonstrated not only major differences in intestinal mucosal growth with enteral feeding compared with PN, but also increases in hepatic and superior mesenteric artery blood flow with feedings.4 Studies have also suggested that enteral feedings increase superior mesenteric blood flow and could thus be a predictor for increased tolerance to enteral feedings in preterm infants.5 Another study showed that enteral feedings increased mesenteric flow in anemic neonates, but that these responses were blunted in infants receiving blood transfusions.6 This further suggests that enteral feedings may represent a protective mechanism in counteracting gut ischemia. Studies in animals using near-infrared spectroscopy measurement as an indicator of intestinal blood flow suggested correlations to NEC in premature piglets.7 Early enteral nutrition also provides trophic benefits and promotes hormonal secretion by nutrient stimulation to the gut and should preclude the need for using prolonged PN.8,9
Immature mucosal barrier function, immature immune response, and alterations of the intestinal microbiota are believed to make preterm neonates particularly susceptible to intestinal inflammation, injury, and bacterial translocation. Late-onset sepsis (LOS), a complication commonly seen in preterm infants, is associated with prolonged use of intravenous catheters for PN because of delays in achieving full enteral feeds or the need for central lines for administration of medications. The likelihood that LOS is at least partially caused by the lack of enteral feedings rather than a catheter-related infection is supported by studies that Wildhaber and colleagues conducted using rodents. These studies showed a combination of enteral feeding and a central line resulted in a much lower incidence of intestinal bacterial translocation than the presence of a PN line without receiving enteral nutrition.10 In fact, a reduction in incidence of bacteremia was observed in VLBW neonates who achieved full enteral nutrition before the second week of life.11
There is a strong correlation between overall size of the intestine and its surface area. In term infants, the length of the small intestine can reach approximately 275 cm. Consequently, the inherent characteristics of the villi and microvilli represent an extensive amount of exposed surface area. Half of the growth in length of the intestine occurs during the last trimester of gestation.12 During this time, the fetus is known to swallow approximately 400 mL of amniotic fluid per day. This is a physiologic process that may play an important role in the development of the gastrointestinal tract. This process is suddenly interrupted with preterm birth. It is important to be aware of some of the developmental processes that occur during this time so that enteral nutrition may be optimized.
PROTEIN DIGESTION AND ABSORPTION
General
The composition of proteins ingested by neonates largely reflects that of either the mother’s milk or the commercial formulas given. The protein content and composition of human breast milk change throughout the lactation period. For instance, at the beginning of lactation, the protein content is about 2 g/dL with a whey to casein ratio of about 80:20; this changes to a protein content of about 1 g/dL with an about 60:40 whey to casein ratio in the following weeks with the evolution of more mature milk. Most preterm infant formulas are formulated to reflect the latter whey to casein ratio. These different proteins have different amino acid profiles and different digestibility given the concept of “fast” and “slow” proteins. Whey proteins will produce a rapid, high but transient increase in circulating insulin and aminoacidemia, whereas casein proteins produce more gradual, and relatively lower, but more sustained increase in insulin and amino acids.13
Several studies have evaluated the metabolic and clinical benefits of enteral amino acid supplementation.14–17 The postprandial rise in amino acids and insulin stimulates protein synthesis in the neonate and therefore growth.18,19 Among the individual amino acids that have specific roles in regulating protein synthesis and immune function are leucine, glutamine, and arginine. Leucine is the key amino acid that triggers the stimulation of protein synthesis.20,21 Using neonatal pig models, studies have shown that enteral or parenteral leucine will stimulate protein synthesis in the muscle and visceral tissue of the neonate even if formulas with low protein milk were used.22,23 The stimulation of protein synthesis by leucine is through the activation of the mammalian target of rapamycin (mTOR), a nutrient sensor protein kinase, which integrates the input from upstream pathways, including insulin, growth factor, and amino acids.21–23
Arginine is an essential amino acid that in the neonate is exclusively synthesized in gut epithelial cells from amino acid precursors such as citrulline, glutamine, and glutamate. Arginine is also a precursor of nitric oxide (NO), a potent vasodilator, and will therefore increase intestinal blood flow.24,25 Clinical studies have shown that low levels or a deficiency of arginine in preterm infants is associated with an increased incidence of NEC.26,27 Randomized controlled trials have shown that when arginine supplementation with quantities as low as 1.5 mmol/kg/d are given to premature infants, a reduced incidence of NEC results.15
Another amino acid of importance is glutamine; although nonessential, it is not sufficiently synthesized in catabolic states. Several human and animal studies have suggested that glutamine supplementation in the critically ill patient has some benefits as an immunomodulator nutrient.28–31 Glutamine in the intestine acts as an energy substrate, a precursor to gluthathione, and a nitrogen donor for other amino acids and nucleotides. Despite the attributed benefits of glutamine, studies of enteral supplementation with glutamine in VLBW infants failed to show reduction in nosocomial sepsis. However, on secondary analysis, the neonates who received glutamine supplementation had less gastrointestinal dysfunction and lower intraventricular hemorrhages.14 A Cochrane review of 7 highly heterogeneous randomized controlled trials of glutamine supplementation in preterm infants failed to demonstrate any statistically significant effect on mortality or other neonatal morbidities.32
The digestion of proteins begins in the acidic environment of the stomach and continues in the small intestine under the influence of pancreatic proteases and peptidases. Most dietary proteins in human infants must first be digested into amino acids or di- and tripeptides. Proteolytic enzymes are secreted into the lumen of the upper digestive tube from 2 primary sources: (1) The chief cells of the stomach secrete pepsinogen, which is then converted to the active protease pepsin by the action of acid, and (2) the pancreas secretes a group of potent proteases, among them trypsin, chymotrypsin, and carboxypeptidases, which require activation by enterokinase. Through the action of these gastric and pancreatic proteases, dietary proteins will be hydrolyzed within the lumen of the small intestine predominantly into medium and small peptides (oligopeptides).
Subsequently, small peptides, primarily di- and tripeptides, are absorbed into the small intestinal epithelial cell by cotransport with H+ ions.33,34 Once inside the enterocyte, the vast bulk of the absorbed di- and tripeptides is hydrolyzed into single amino acids by cytoplasmic peptidases and exported from the cell into blood. Only a few of these small peptides enter blood intact.
Absorption of amino acids is dependent on the electrochemical gradient of sodium across the epithelium in the small intestine. Further, absorption of amino acids, like that of monosaccharides, contributes to generating the osmotic gradient that drives water absorption. The basolateral membrane of the enterocyte contains additional transporters that export amino acids from the cell into blood; these are not dependent on sodium gradients.
The contribution of the microflora to gastrointestinal amino acid metabolism may be nutritionally important. Studies have indicated that essential amino acids are synthesized and absorbed by the gastrointestinal flora.35
Developmental Aspects of Protein Digestion and Absorption
Digestion
Gastric Acidity
When comparing full-term and premature infants, hydrochloric acid secretion was found to be much lower in premature infants than in full-term infants.36 Of significance, however, is that both basal and pentagastrin-stimulated acid secretion were noted to double from the first to fourth week of postnatal life in preterm infants.37 The actual pH of the stomach contents in infants is influenced substantially by food intake. The entry of milk into the infant’s stomach causes a sharp increase in the pH of the gastric contents and a slower return to lower pH values than in older children and adults.38
Pancreatic Proteolytic Activity
The protease cascade in the small intestine is catalyzed by food-stimulated secretion of enterokinase from the upper small intestinal epithelium. However, even though enterokinase is detectable at 24 weeks’ gestation, its concentration is relatively low and reaches only 25% of adult activity at term.39 This theoretically can be limiting to protein digestion and may be responsible for an increased capability of larger antigens or microorganisms to pass into the intestine without breakdown by luminal enzymes.
Pancreatic secretion of proteolytic enzymes starts at the beginning of the fifth month of gestation. Levels of trypsin concentration encountered during the first 2 years of life are reached by the age of 3 months.40 From birth onward, the concentration of chymotrypsin (after pancreozymin-secretin stimulation) increases approximately 3-fold and reaches adult levels in 3-year-old children.40 Serial measurement of fecal chymotrypsin concentrations in preterm infants (23 to 32 weeks’ gestation) during the first 4 weeks of life demonstrated values generally similar to those found in term infants.40
Absorption
For a few days after birth, the “leakiness” of the neonatal gut has the ability to absorb intact proteins. This ability, which is rapidly lost in a process called closure, is of importance because it allows the newborn to acquire passive immunity by absorbing immunoglobulins in colostral milk. Thus, the ability of the gastrointestinal tract to exclude antigenically intact food proteins increases with gestational age, and that gut closure occurs normally before birth.41
Proteolytic and Peptidase Activity
In one study, brush border and intracellular proteolytic enzyme activities were measured in fetuses (8–22 weeks’ gestation), children (7 months to 14 years), and adults. The peptidase activities in all 3 groups were comparable, suggesting that the small intestine of the term and preterm newborn should be able to efficiently digest peptides.42
Clinical Correlations
The fetus accretes about 2.2 g/kg/d of protein at 26 weeks’ gestation, and it declines to about 0.9 g/kg/d in the term infant. The placenta supplies up to 3.5 g/kg/d of amino acids to the developing fetus, and preterm delivery will abruptly interrupt this amino acid supply and any further protein accretion. Herein lies the importance of starting nutrition immediately after birth, especially in the preterm infant. Early parenteral and enteral nutrition has been shown to be safe and to decrease morbidity.8,43 Amino acids supplied in excess of those needed for protein accretion are oxidized and contribute to energy production. Certain patients have a transient increase in the blood serum nitrogen (BUN) levels that usually does not exceed 60 mg/dL.44 In the absence of an inborn error of metabolism, this is of no clinical significance, and preterm infants normally tolerate it well without risk of encephalopathy.
The limited capacity of premature infants to secrete acid should be considered when using histamine 2 (H2) blockers or proton pump inhibitors, which are widely prescribed in many NICUs, especially for the treatment of symptomatic gastric reflux. Some studies suggest that critically ill premature infants treated with H2 blockers have a higher incidence of nosocomial sepsis and NEC.45,46 Therefore, the use of H2 blockers should be undertaken with caution and perhaps only in infants that have failed other interventions. Although speculative, it is possible that with the already-limited hydrogen ion production in the stomach of the premature infant, additional blockage further diminishes the acid barrier to microorganisms and allows for a higher load of bacteria in the more distal regions of the intestine.
Despite the digestive limitations and decreased enzymatic capabilities of premature infants, no significant data have been offered to demonstrate benefits or advantages of using a formula of hydrolyzed protein fractions over whole-protein formulas,45,47 and studies have yet to demonstrate any benefit for choosing a hydrolyzed protein formula over human milk.
CARBOHYDRATE DIGESTION AND ABSORPTION
General
The predominant carbohydrate in human milk and of most infant formulas is lactose. Lactase intestinal activity is limited in the preterm infant when compared to term infants. Interestingly, lactose intolerance is rarely seen in the preterm infant.
Starches and complex carbohydrates must first be hydrolyzed to oligosaccharides by digestive processes in the mouth, stomach, and intestinal lumen. This is accomplished primarily via salivary and pancreatic amylases. Oligosaccharides must then be hydrolyzed in the intestinal epithelial brush border into monosaccharides prior to their absorption. The catalysts required for this process to proceed are the brush border hydrolases, namely, maltase, lactase, and sucrase. Dietary lactose, sucrose, and maltose come in contact with the absorptive surface of the epithelial cells covering the intestinal villi, where they engage with brush border hydrolases: maltase, sucrase, and lactase.
Developmental Aspects of Carbohydrate Digestion and Absorption
Carbohydrate Digestion
Pancreatic amylase activity has been demonstrated in amniotic fluid and pancreatic tissue from 14- to 16-week-old fetuses.48,49 Although salivary amylase activity rapidly increases shortly after term birth, pancreatic amylase remains low until 3 months of age and does not reach adult levels until nearly 2 years.50
Carbohydrate Absorption
Although lactase activity in the small intestine of preterms is thought to be limited, suggesting a limited capacity for lactose hydrolysis, colonic fermentation activity is adequate for colonic salvage of lactose even during the second week of life. Using a stable isotope method for serial assessment of lactose carbon assimilation, Kien and associates51 demonstrated efficient absorption of lactose in premature infants (30 to 32 weeks’ gestation and 11 to 36 days of age). The predominant carbohydrate found in human milk is lactose, and human milk is usually tolerated better than formulas in low birth weight infants.
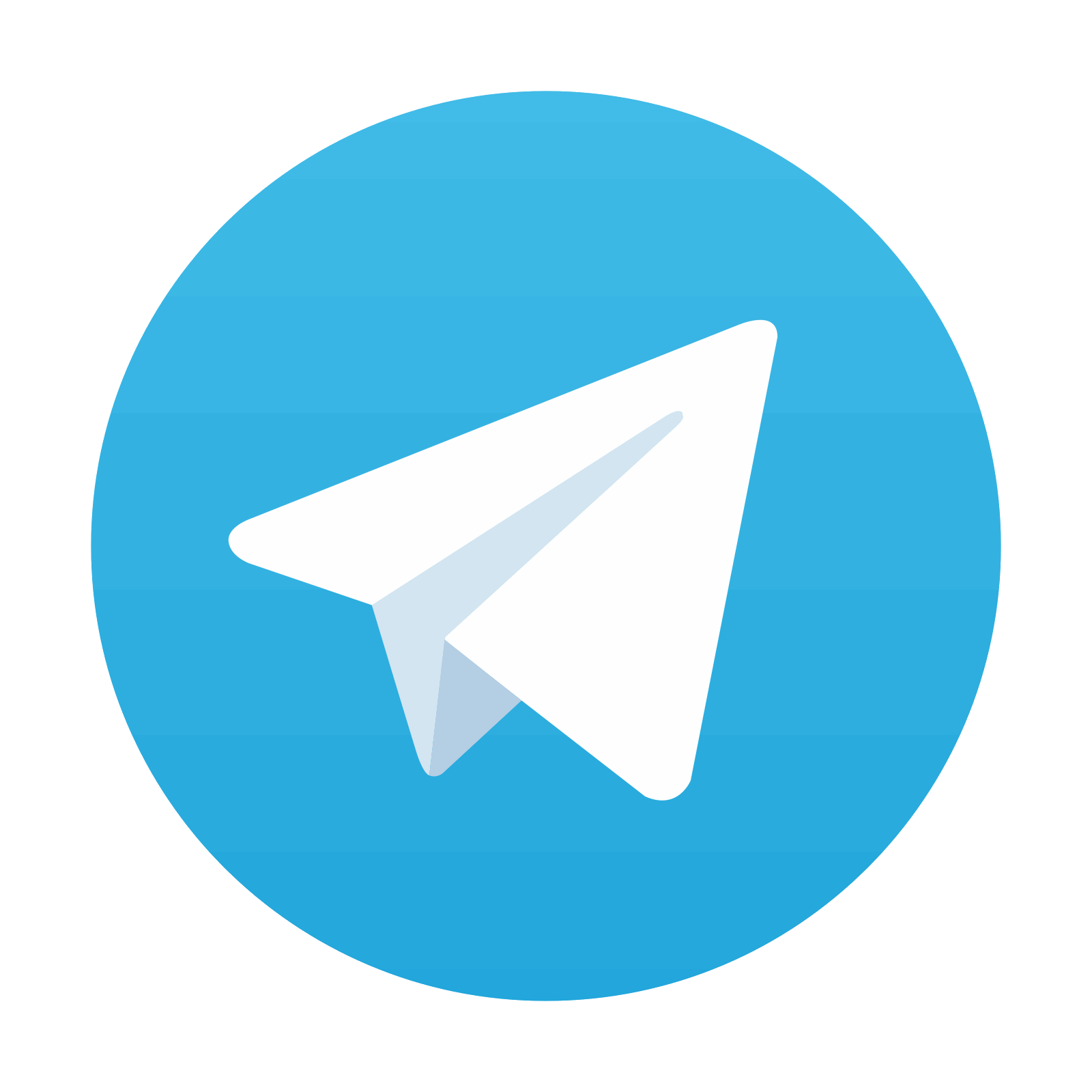
Stay updated, free articles. Join our Telegram channel

Full access? Get Clinical Tree
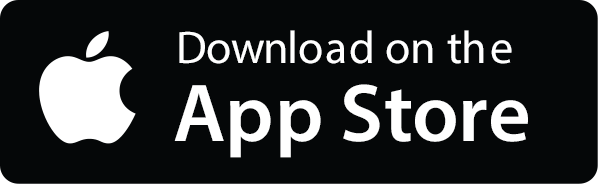
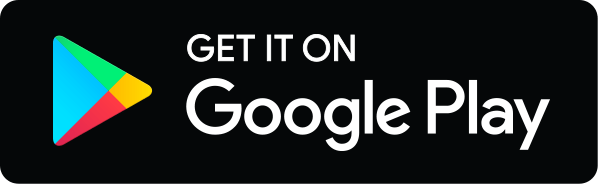