Drowning and Hypothermia
Melanie L. Brown
Madelyn Kahana
Department of Pediatrics, University of Chicago, Pritzker School of Medicine, Chicago, Illinois 60637.
University of Chicago, Children’s Hospital Pediatric Care Center, Chicago, Illinois 60637.
DROWNING AND NEAR-DROWNING
In the United States, drowning is the second leading cause of death in children. In 10 states, submersion injuries claim the lives of more children than any other injury or disease process (1). Preschool-age children and adolescents are at greatest risk—the former, victims of unprotected backyard swimming pools, the latter, victims of the effects of alcohol-related boating accidents. Male victims outnumber female victims in all age groups, particularly in adolescents, for whom the ratio approaches 10:1. For every child who drowns, four children are admitted to the hospital with significant near-drowning episodes, and are evaluated and discharged.
Pathophysiology
Drowning and near-drowning result in multisystem hypoxic injury. Initially, there is a period of struggling, during which small amounts of water enter the hypopharynx and produce laryngospasm. Copious amounts of water are then swallowed, followed by gasping and aspiration. In 10% to 15% of animals, laryngospasm persists until death, with no significant aspiration of water into the tracheobronchial tree (2). Autopsy examinations of the lungs of drowning victims support the notions that pulmonary aspiration is an active process and that trivial quantities of water flow into the trachea passively after consciousness is lost (3). During resuscitation efforts, gastric contents are almost invariably regurgitated, which complicates the pulmonary injury.
As a result of the period of asphyxia, the drowning victim suffers a global hypoxic injury. Although the pulmonary insult is often the focus of discussions of the pathophysiology of drowning and near-drowning, the cerebral injury is the most likely cause of morbidity and mortality in the modern era of pediatric critical care medicine. Other expressions of the hypoxic injury include acute tubular necrosis, loss of gastrointestinal mucosa, depression of myocardial contractility, hepatic necrosis, and disseminated intravascular coagulopathy (4). In the rare event that large quantities of water are absorbed into the systemic circulation, hemolysis adds to the hematologic pathology and aberrations in serum electrolytes occur (4).
Much is often made of the differences between the injuries that result from freshwater and saltwater submersions. Only 2% of drowning and near-drowning accidents occur in saltwater, so that even if the differences were not trivial, the magnitude of the problem of saltwater drowning is small. In addition, the injury that results from submersion is one of hypoxia. Only in experimental models, when more than 22 mL per kg of water is instilled into the trachea, do the differences between freshwater and saltwater drownings become important.
In theory, saltwater drowning results in rapid diffusion of fluid into the lung from the extravascular space because of the high osmolality of seawater. Protein-rich pulmonary edema results, and surfactant is deactivated. With freshwater aspiration, surfactant is altered, and the lung collapses from the loss of surface tension. In both instances, pulmonary compliance falls and the work of breathing becomes excessive. In both instances, respiratory failure is inevitable. In both circumstances, the extent and duration of the hypoxic period determine the severity of subsequent global injury (4).
If there is massive aspiration of fluid at the time of the accident, the victim of saltwater submersion has immediate and profound pulmonary edema. Intravascular volume is contracted, with the potential for severe hypernatremia from the osmotic movement of water from the intravascular space (2). In the case of the freshwater victim, pulmonary edema is less evident and the potential for intravascular volume overload is real. Osmotic forces drive water into the intravascular space and classic water
intoxication follows, with the possibility of life-threatening hyponatremia. Fewer than 10% of submersion victims aspirate sufficient fluid to make these differences clinically relevant.
intoxication follows, with the possibility of life-threatening hyponatremia. Fewer than 10% of submersion victims aspirate sufficient fluid to make these differences clinically relevant.
The diving reflex, a generally vestigial reflex in humans, active perhaps in 15% of the population, may be important in the recovery from prolonged submersion (5,6). The diving reflex is responsible for the ability of marine mammals to remain underwater for prolonged periods of time despite their need to respire on the surface. The major factor in their resistance to the consequences of prolonged asphyxia is a set of cardiovascular physiologic adjustments triggered by breath-holding diving, which conserves oxygen for those tissues most sensitive to hypoxia. Cardiac output is markedly reduced secondary to profound bradycardia. Peripheral vasoconstriction limits or arrests blood flow to skeletal muscle, the intestinal tract, and the kidneys. Cerebral blood flow is maintained at or slightly above predive levels. Coronary flow continues at a reduced level. Oxygen consumption drops dramatically to less than one-half the predive rate. The diving reflex is accentuated by hypothermia, enabling marine mammals to survive up to 70 minutes of submersion. The importance of this reflex in human drowning survivors is still hotly debated.
Treatment Issues
Despite descriptions in the medical literature and the lay press of dramatic recoveries from prolonged submersions, the success of resuscitation therapy for the drowning victim is limited. The extent of the injury inflicted and the potential for recovery are largely predetermined by the duration of the hypoxic period. Prompt prehospital resuscitation is crucial for the child with a potentially reversible process. Delaying initiation of basic or advanced life support in this patient population augments the hypoxic insult. Once the child has suffered a cardiopulmonary arrest, the outcome is usually dismal despite even the best resuscitative efforts—except perhaps in the special circumstance of the cold water drowning victim.
At the time of resuscitation, most submersion victims regurgitate. If gastric contents are aspirated, lung injury can be magnified. In the rare circumstance in which particulate matter is aspirated and the airway is obstructed, the removal of the offending material must precede further resuscitative efforts. Although routine use of the Heimlich maneuver or abdominal thrusts in the near-drowning victim has been widely discussed in the literature, the American Red Cross continues to recommend not delaying basic life support to perform this maneuver except in the case of the patient with an airway obstruction (3,7,8). The presence of water in the tracheobronchial tree does not warrant the routine performance of the Heimlich maneuver, which may, in fact, increase the quantity of regurgitated material and hamper efforts at maintaining a patent airway.
If the submersion injury occurs in a contaminated body of water, the pulmonary injury is augmented by the presence of toxic chemicals or bacteria in significant quantities.
The lung injuries that occur as a result of submersion in freshwater and saltwater are more alike than they are different (2,4). Compliance is reduced, surfactant is deactivated, and ventilation–perfusion mismatch produces hypoxemia. In severe injury, endotracheal intubation is mandated by blood gas aberrations, the increased work of breathing, and the altered mental status of the patient. The pulmonary injury often rapidly evolves into a pattern consistent with the acute hypoxic respiratory failure (AHRF).
The use of positive end-expiratory pressure (PEEP) to reduce ventilation–perfusion mismatch is the mainstay of successful mechanical ventilation. Efforts to reduce the inspired oxygen concentration to less than 0.6 limit oxygen-related pulmonary injury (2,4). Allowing hypercapnia is recommended in lieu of the normalization of blood gases in an effort to limit tidal volume to 5 to 7 mL per kg (9) and peak inflation pressure to less than 35 cm H2O. It is important to note that increased CO2 can cause changes in cerebral blood flow. These changes can lead to increased cerebral hypertension in the patient with anoxic encephalopathy.
Placing the patient with AHRF in prone position for 7 to 12 hours daily has received much attention as a strategy to increase oxygenation without significantly adding to morbidity (10,11). Interestingly, Kornecki et al. also observed that prone positioning caused increased diuresis with decreased positive fluid balance. It is likely that prone positioning may also improve mortality, but further study is needed. Inhaled nitric oxide and instillation of surfactant into the trachea by injection and by nebulization is being investigated with promising results. High-frequency oscillation has been used with success in the patient with severe lung injury, as has extracorporeal membrane oxygenation.
Conventional ventilation strategies begin with volume- or pressure-limited, time-cycled settings. The goals are twofold. First, the adequacy of oxygen delivery and carbon dioxide removal must be ensured. Second, the injury imposed by the effects of high concentrations of inspired oxygen and excessive tidal volume must be limited. Without attention to the latter, a potentially nonlethal pulmonary injury can evolve into one with mortal consequences. When the concentration of inspired oxygen reaches levels above 0.6, oxygen-related tissue injury is a real danger. To accomplish adequate oxygenation and limit the fraction of inspired oxygen, PEEP therapy should be instituted. Frequently, more than 15 cm H2O, delivered as PEEP, is necessary to reduce inspired oxygen to a safe level.
Adjunctive therapy with steroids and antibiotics has been recommended for the pulmonary injury from near-drowning. There is little evidence to support the use of corticosteriods in the early stages of near-drowning; however, more recent studies suggest that steroids may be of
some benefit in the late stages of adult respiratory distress syndrome (ARDS) (12). Initially, antibiotics should be reserved for the victim of a contaminated water source. During hospitalization, nosocomial pneumonia certainly occurs in this patient population, and antibiotics should be tailored for the specific infectious agent whenever possible.
some benefit in the late stages of adult respiratory distress syndrome (ARDS) (12). Initially, antibiotics should be reserved for the victim of a contaminated water source. During hospitalization, nosocomial pneumonia certainly occurs in this patient population, and antibiotics should be tailored for the specific infectious agent whenever possible.
The high intrathoracic pressure that results from the use of positive-pressure mechanical ventilation can diminish cardiac output sufficiently to require inotropic support. If this occurs, the placement of a pulmonary artery catheter can be helpful in guiding therapy. Cardiac output and oxygen delivery and extraction can then be measured. These data facilitate appropriate adjustments in drug selection and dose, as well as the manipulation of preload with minimal risk of imposed hydrostatic pulmonary edema. After ensuring the volume status is adequate, the selection of the appropriate vasopressor is essential in cases of hypotension. In general, these agents should be administered through a central line.
The intentional elevation of oxygen delivery to supranormal levels in an effort to improve survival has garnered some enthusiasm, but there is very limited evidence to support this contention. The adequacy of oxygen therapy and delivery in the child with a severe lung injury is best evaluated with the data acquired from a pulmonary artery catheter. Mixed venous oxygen saturation should be maintained at or above 65% whenever possible. This saturation level requires that the risks of therapy be considered when adjustments are made to achieve this goal. The child with low oxygen consumption may have a favorable mixed venous saturation, but is not likely to do well in the long term.
Oxygen delivery can be improved by numerous interventions. Transfusion of red cells, measures to increase cardiac output, and increases in arterial saturation augment oxygen delivery. The optimal hematocrit has not been determined, but in the severely injured child, transfusion to a hemoglobin of 15 g per dL is prudent. Cardiac output can be improved by manipulating preload, contractility, and afterload. The successful cardiopulmonary support of the pediatric near-drowning patient, although often complex, is almost always possible.
The success of cardiopulmonary support in the drowning victim is offset by the inability of any available therapy to modify the cerebral hypoxic insult. Although methods to reduce cerebral oxygen consumption have been used, no data support that a reduction improves survival. Both hypothermia and barbiturate coma significantly reduce cerebral oxygen consumption, but neither increases the number of neurologically intact survivors. Monitoring intracranial pressure has been recommended to guide therapy, but again, no data support an increase in intact survivors as a result. In fact, data suggest that monitoring intracranial pressure and aggressively treating intracranial hypertension increase the number of vegetative near-drowning survivors (13). Refractory intracranial hypertension predicts poor long-term outcome.
If intracranial pressure monitoring is used, optimal cerebral perfusion pressure (CPP) should be the goal. The CPP is the difference between the mean arterial pressure (MAP) and the intracranial pressure (ICP). Just as normal values for ICP vary with age, recommendations for minimum CPP also vary. CPP greater than 70 mm Hg is recommended in adults to maintain adequate tissue perfusion and oxygenation, while the recommendations are a CPP greater than 40 to 50 mm Hg for infants and toddlers, and CPP greater than 50 to 60 mm Hg for older children (14,15). Numerous emergency measures can be used to acutely decrease the ICP in the case of increased ICP and pending herniation, as evidenced by Cushing’s triad (hypertension, bradycardia, hypoventilation), decorticate/decerebrate posturing, or pupillary changes. These measures include mannitol (0.5 to 1 g per kg) in the nonhypotensive patient, short-term hyperventilation (goal PCO2 30 to 35), proper sedation, and the removal of unnecessary noxious stimuli. Long-term or overly aggressive hyperventilation has been associated with increased cerebral ischemia.
The treatment of the renal insufficiency that accompanies near-drowning is possible because the injury is transient. If dialysis is required, the duration of acute renal failure is generally between 3 and 6 weeks. Hemodialysis or continuous venovenous hemofiltration is preferred in the patient with a significant pulmonary insult because peritoneal filling compromises the ability to ensure adequate mechanical ventilation.
The gastrointestinal mucosal injury is also short lived, but demands the use of parenteral nutrition until the mucosa regenerates. Because early and aggressive nutritional support is crucial in the injured child, hyperalimentation is recommended until the gut can support adequate caloric intake. As soon as possible, the enteral route of nutritional support is preferred. Trophic feeds can generally be started within the first 24 to 48 hours of injury.
The hematologic insult in near-drowning injury is a multifactorial one. Disseminated intravascular coagulopathy accompanies hypothermia, hypoxemia, and shock. Hypoxemia injures the bone marrow and results in markedly reduced platelet production that can persist for several weeks. The use of broad-spectrum antibiotics can cause vitamin K deficiency and attendant coagulopathy if replacement therapy is not instituted. Hematologic support of the drowning victim should be tailored to the specific needs of the patient. To avoid hemorrhagic complications, coagulation variables should be normalized whenever possible.
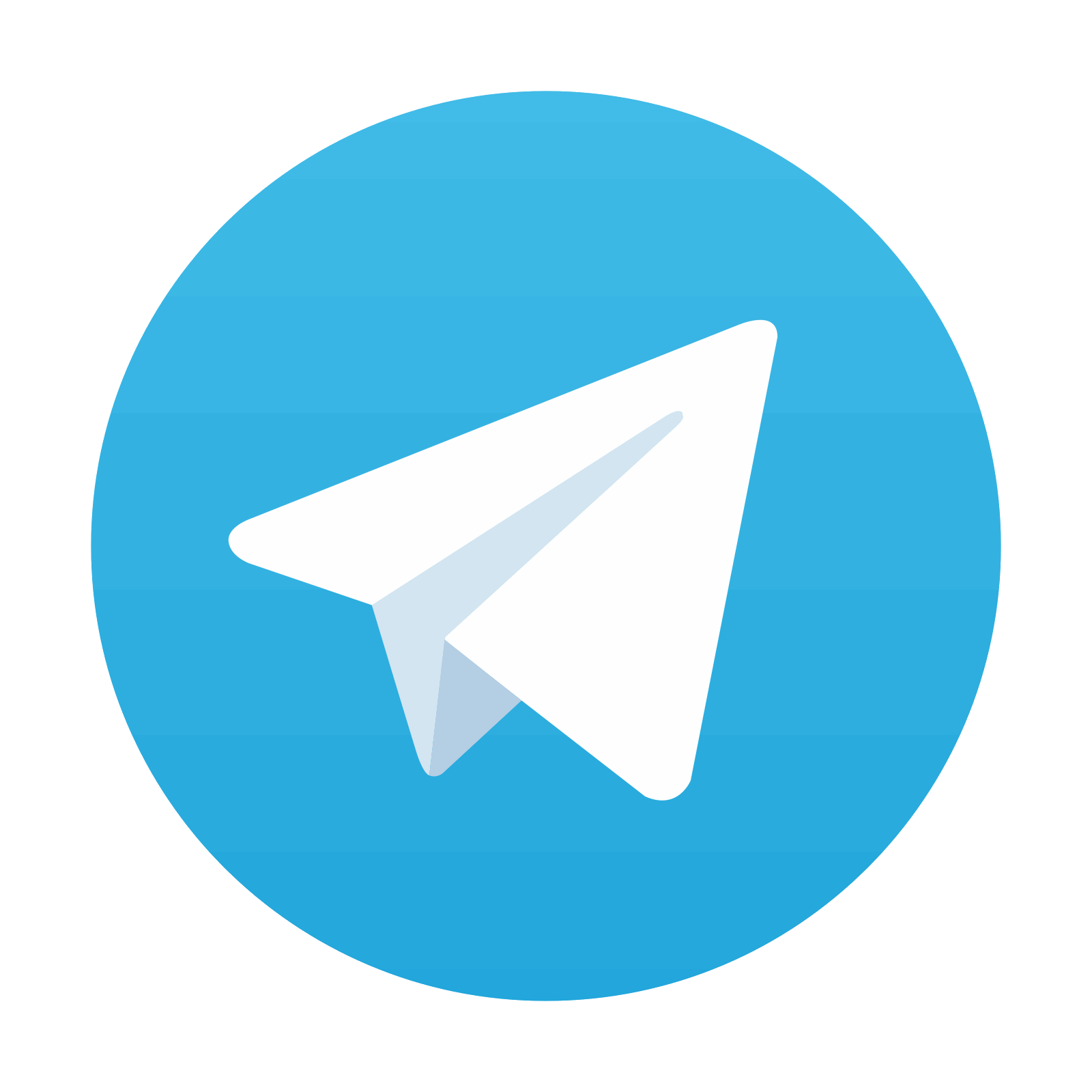
Stay updated, free articles. Join our Telegram channel

Full access? Get Clinical Tree
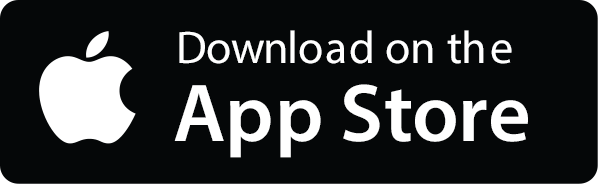
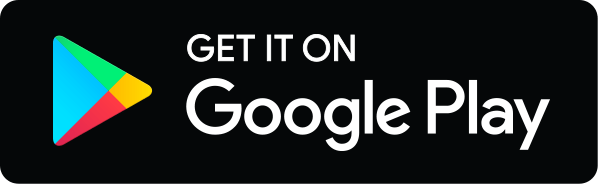