Although knowledge is increasing from randomized trials, the majority of these recommendations are based on observational human and animal data but have not been rigorously tested. It is expected that in the future, some of these recommendations will undergo close scrutiny and possible change.
![]() FIGURE 17.2 A and B: Fetal and neonatal circulation. From David Atkinson, MD, David Geffen School of Medicine at UCLA, with permission. |
insufficient or excessive oxygenation can be harmful to the newborn infant (14,15). Percentiles of oxygen saturation as a function of time from birth in uncompromised babies born at term have been recently published (Fig. 17.4) (16). To appropriately compare oxygen saturations to similar published data, the probe should be attached to a preductal location (i.e., the right upper extremity, usually the medial surface of the palm). During respiratory support, a special emphasis should be given to prevent oxygen-induced lung injury by providing the lowest level of oxygen supplementation that maintains adequate delivery of oxygen to tissues (17,18,19). Studies suggest that resuscitation with 100% oxygen may generate oxygen free radicals, which may cause tissue damage, particularly to the brain (15,20,21). Therefore, continuous monitoring of oxygen saturation should start in the DR by using recently published nomograms (Fig. 17.4) to reduce overall oxygen exposures (16). The current resuscitation guidelines recommend to start resuscitation in room air in all term infants (4). Although, for preterm infants, no definitive recommendation has been made (4), there is increasing evidence that DR resuscitation of preterm neonates with an initial oxygen of less than 30% is feasible, decreases oxygen exposure without increasing need for additional resuscitation, and decreases oxidative stress (18,19,22). In the absence of DR studies comparing important clinical outcomes such as bronchopulmonary dysplasia and long-term neurodevelopment, neonatal resuscitation of preterm infants should be initiated with air or a blended oxygen and titrating the oxygen concentration to achieve an oxygen saturation value in the interquartile range of preductal saturations measured by pulse oximetry (Fig. 17.4) (4,16).
TABLE 17.1 Some Factors That Place the Newborn at High Risk for Asphyxia | |||||||||||||||||||||||||||||||||||||||
---|---|---|---|---|---|---|---|---|---|---|---|---|---|---|---|---|---|---|---|---|---|---|---|---|---|---|---|---|---|---|---|---|---|---|---|---|---|---|---|
|
TABLE 17.2 Fetal Heart Rate Patterns Associated with Fetal and Neonatal Distress | ||||||||||||
---|---|---|---|---|---|---|---|---|---|---|---|---|
|
of atelectrauma and improve respiratory function in different ways: (a) CPAP reduces upper airway obstruction by decreasing upper airway resistance and increasing the pharyngeal cross-sectional area; (b) both CPAP and PEEP increase FRC; (c) CPAP and PEEP reduce inspiratory resistance by dilating the airways and allow a larger tidal volume for a given pressure with a reduction in the work of breathing; (d) CPAP and PEEP increase the compliance and tidal volume of stiff lungs with a low FRC by stabilizing the chest wall; (e) CPAP and PEEP increase the mean airway pressure and improve ventilation-perfusion mismatch; (f) PEEP conserves surfactant on the alveolar surface; (g) as CPAP and PEEP increase lung volume, oxygenation is also improved (23).
TABLE 17.3 Team Assignment During Neonatal Resuscitation | |||||||||||||||||||||||
---|---|---|---|---|---|---|---|---|---|---|---|---|---|---|---|---|---|---|---|---|---|---|---|
|
negative pressure, produces a fall in inflation pressures but results in a transient increase in tidal volume. Of course, the neonate may demonstrate no response to the inflation attempt, that is, not generating any change in intraesophageal pressure during PPV, and passive inflation subsequently results. It is important to recognize that these physiologic responses to PPV in the DR may cause large variability in the tidal volume and intrapulmonary pressures, despite delivery of constant inflation pressure.
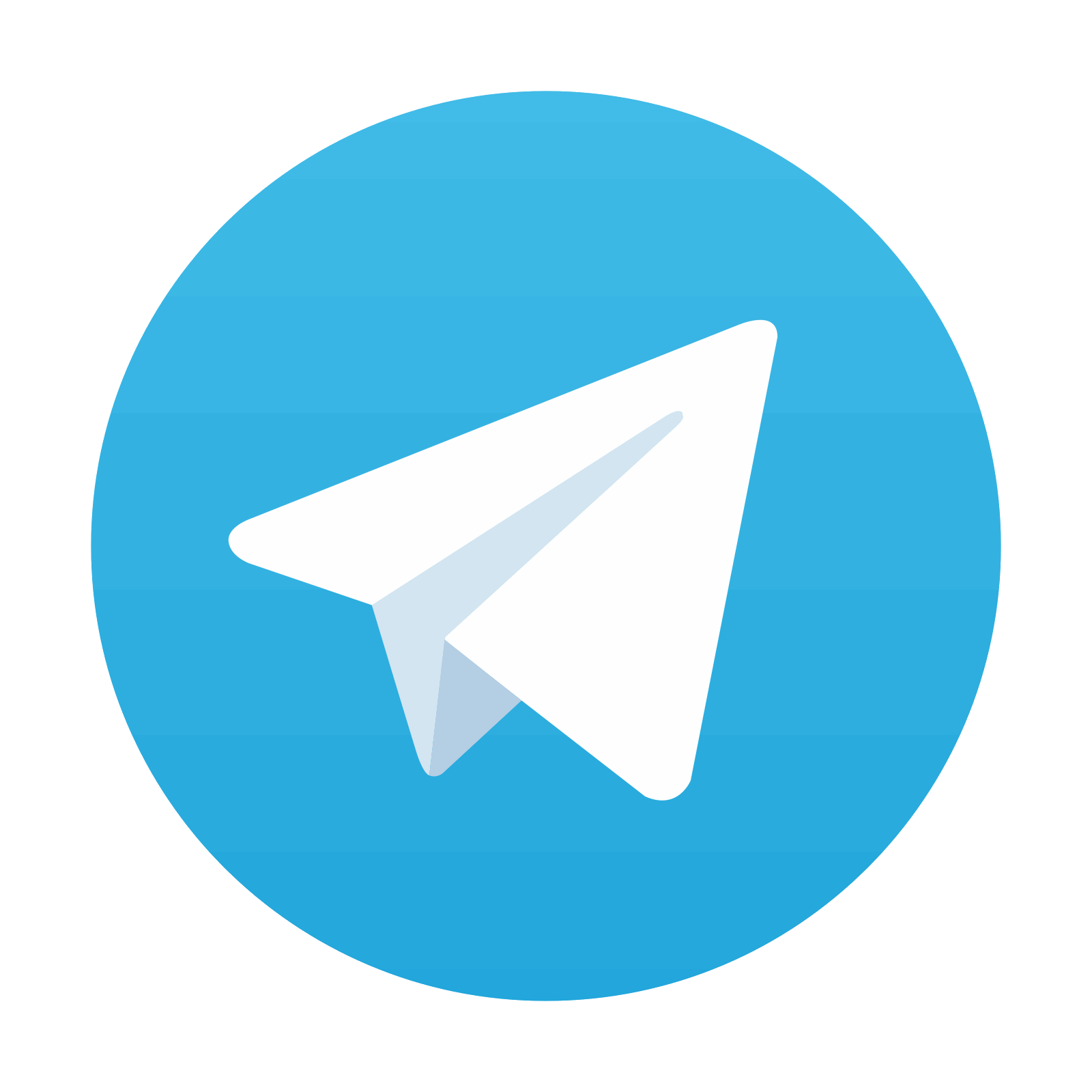
Stay updated, free articles. Join our Telegram channel

Full access? Get Clinical Tree
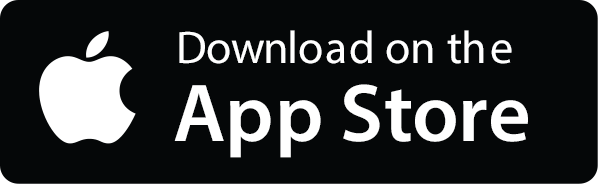
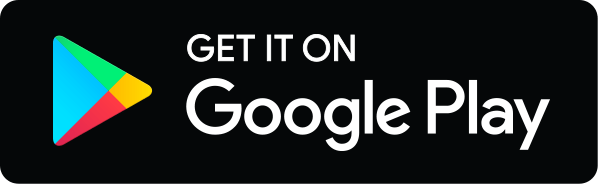
