Abstract
At the beginning of the menstrual cycle, there is an increase in bioactive follicle-stimulating hormone (FSH) levels, a stimulus for the growth and differentiation of follicular granulosa cells (GCs). GC steroidogenic enzymes are also inducible by FSH and are necessary for the production of estradiol (E2) and progesterone (P4), as well as expression of luteinizing hormone (LH) receptors on theca cells (TCs). LH then stimulates theca cells to produce androgens, which are metabolized to E2 by GCs under the influence of FSH. Elevated levels of E2 then inhibit FSH secretion, providing a negative feedback effect. Growth of the leading follicle continues owing to elevated levels of FSH receptors, whereas secondary follicles with fewer FSH receptors undergo atresia. Taken together, FSH and LH work in concert, as depicted by the classic two-cell (TC and GC), two-gonadotropin (FSH and LH) theory.
Two-Cell and Two-Gonadotropin Theory
At the beginning of the menstrual cycle, there is an increase in bioactive follicle-stimulating hormone (FSH) levels, a stimulus for the growth and differentiation of follicular granulosa cells (GCs). GC steroidogenic enzymes are also inducible by FSH and are necessary for the production of estradiol (E2) and progesterone (P4), as well as expression of luteinizing hormone (LH) receptors on theca cells (TCs). LH then stimulates theca cells to produce androgens, which are metabolized to E2 by GCs under the influence of FSH. Elevated levels of E2 then inhibit FSH secretion, providing a negative feedback effect. Growth of the leading follicle continues owing to elevated levels of FSH receptors, whereas secondary follicles with fewer FSH receptors undergo atresia. Taken together, FSH and LH work in concert, as depicted by the classic two-cell (TC and GC), two-gonadotropin (FSH and LH) theory. This theory of natural and artificial hormonal activity exists according to ligand–receptor interactions.
Ligand–Receptor Interaction
Cell surface receptors (membrane receptors, transmembrane receptors) take part in communication between the cell and the outside world. Extracellular signaling molecules attach to the receptor and activate cytoplasmic signals, which are then transferred into the nucleus. Steroid hormones bind to cytosolic receptors and are transferred into the nucleus. Once the receptor is occupied, it activates intracellular second messengers. These messengers convey signals to “switch on” or “switch off” gene expression, which then leads to a cellular response.
Ligands that elicit the same biological effects as natural ligands are called “receptor agonists.” Agonists bind to the receptor at the same binding site as the natural ligand, resulting in either full (conventional agonists) or partial (partial agonists) activation. Conversely, “receptor antagonists” mimic ligands that bind to a receptor and prevent receptor activation, thereby inhibiting the biological activity of the receptor. Receptor antagonists can be classified as reversible and irreversible (Figure 6.1). Reversible antagonists readily dissociate from their receptor, while irreversible antagonists form a stable chemical bond with their receptor. Receptor and/or ligand polymorphisms exist between different people and within the same body, which could affect signal and/or cellular responses. In addition, mutations of ligands or their receptors can affect cellular responses.
Figure 6.1 Receptor agonist and antagonist binding, and their action scheme.
Ovarian Stimulation
Ovarian stimulation technologies have been designed to resolve infertility problems. The treatments can be categorized into three major groups, and are used in accordance with the clinical conditions: 1. ovulation induction, 2. ovarian superovulation, 3. controlled ovarian hyperstimulation (COH). Ovulation induction and ovarian superovulation are technologies used to prepare the ovary for ovulation, after which fertilization occurs by natural intercourse or by intrauterine insemination (IUI). If pregnancy is not achieved by one of these two methods, COH or assisted reproductive technologies (ART) is the next choice of treatment. Its goal is to influence the recruitment of many antral follicles to produce multiple mature oocytes at the final stage, without releasing them. After that, the oocytes are retrieved by aspiration and then fertilized in the laboratory. Our ability to manipulate the selection of follicles is limited; all efforts have been directed toward recruitment in an attempt to bring multiple follicles to dominance instead of the naturally occurring dominance of one follicle only. The aspirated oocytes consist of both mature and immature oocytes.
Before starting the stimulation treatment, a reproductive gynecologist performs transvaginal ultrasounds and blood tests to obtain the baseline hormone levels, and the number of ovarian follicles. The basic hormonal evaluation of women before treatment includes measurement of LH, FSH, prolactin, thyroid-stimulating hormone, E2, P4, 17-OH-P4, testosterone, androstenedione, DHEA, and anti-Müllerian hormone (AMH). Additionally, antral follicle count (AFC), hysteroscopy for women, and semen analysis for men are performed. The results of these tests usually enable clinicians to determine the etiology of infertility and initiate appropriate therapy.
Both AFC and AMH have added value, together with female age and basal FSH, in predicting poor versus high ovarian response to in vitro fertilization (IVF). AMH, recognized as the most accurate biomarker of ovarian reserve, has the advantage of being cycle stage independent. A complete infertility evaluation should be performed before initiating ovarian stimulation to rule out all contributory factors.
Ovulation induction.
Traditional indications for ovulation induction include infertility associated with ovulation deficiencies, e.g., amenorrhea, oligomenorrhea, irregular ovulation, hypogonadotropic hypogonadism, and polycystic ovary syndrome (PCOS). All these cases require hormonal induction of ovulation.
Ovarian superovulation.
This type of hormonal treatment needs regimens for mild ovarian stimulation to obtain a few dominant follicles (two to three follicles), which are then usually treated by IUI. Indications for superovulation include mild to moderate male factor, unilateral tubal occlusion, and unexplained infertility in both the male and the female partner.
Controlled ovarian hyperstimulation.
The purpose of ovarian hyperstimulation is to allow retrieval of multiple mature oocytes during a single IVF cycle. After that, the oocyte is fertilized in vitro, the embryo is cultured, transferred, and implanted. Indications for this approach include mechanical factor and male factor and constitute the second step of treatment after failed ovulation induction and superovulation treatment.
Infertility Due to Defective Ovulation
Ovulation is the result of a delicate, well-synchronized balance between the central nervous system, hypothalamus, hypophysis (pituitary), and ovary. Any disruption of these intricate interactions may lead to defective ovulation and impaired reproduction. Approximately 20% of all female infertility cases can be attributed to ovulatory disorders.
Failure of the hypothalamus to secrete gonadotropin-releasing hormone (GnRH) in the proper pulsatile manner can cause a spectrum of clinical manifestations that range from a normal ovarian cycle to corpus luteum (CL) insufficiency, anovulatory cycle, and oligomenorrhea, to amenorrhea. These disorders are characterized by normal to low serum FSH and LH, and decreased E2 and P4 levels. While direct etiology is not always known, most hypothalamic ovulatory disorders are associated with or are due to physical stress, diet, body composition, emotional stress, environment, and other lifestyle-related aspects.
It is well accepted that emotional stress and psychological factors can influence the hypothalamic–pituitary–ovarian axis, resulting in ovarian dysfunction. Furthermore, psychological counseling or a change in lifestyle was shown to be effective in restoring ovulation in affected individuals. Individuals with amenorrhea and significant weight loss may have anorexia nervosa, or bulimia, or both. Other causes of functional hypothalamic defective ovulation may include malnutrition, malabsorption, and malignancies. All affect the hypothalamus via deregulation of the central nervous system – deregulation of hypothalamic neurotransmitters and neuromodulators. The apparent causes of most cases of hypothalamic ovulatory dysfunctions are related to abnormalities in the neuroendocrine factors that regulate pulsatile GnRH secretion. Hence, the majority of patients with hypothalamic ovulatory dysfunction present an unstable GnRH pulse frequency, which results in the absence of regular cyclical changes in serum FSH and LH concentrations, and regular or enhanced pituitary responsiveness to exogenous GnRH.
A classic developmental disorder is Kallmann syndrome or isolated gonadotropin deficiency. The syndrome results from the early degeneration of GnRH-producing neurons due to maldevelopment of the olfactory bulbs and the sulci of the rhinencephalon, leading to insufficient GnRH production and improper pituitary function.
Hypogonadotropism-associated defective ovulation can result from pituitary disorders that damage gonadotropin-secreting cells. Clinical appearance depends on the age of onset, the etiology, and the nutritional status of the patient and can be obvious or very subtle.
Clomiphene Citrate for Ovulation Induction
First synthesized in 1956, clomiphene citrate (CC) has been used clinically for ovulation induction since the 1960s. CC is a mixture of cis- and trans-isomers of a triphenylethylene derivative that acts as an E2 receptor blocker, with the cis-isomer seemingly constituting the active form [1]. CC is well absorbed orally and is metabolized by the liver to inactive metabolites. CC has an antiestrogenic role at the level of the hypothalamus, which leads to a significant increase in the pulse frequency of LH on cycle day 5 after treatment initiation [2]. CC regimens are initiated at a dose of 50 mg daily and increased until an appropriate E2 rise is registered, up to a maximum of 250 mg/day. CC is discontinued after an E2 response is noted, and human chorionic gonadotropin (hCG) is then administered when ultrasound demonstrates a lead follicle of 18 mm to 19 mm in diameter. Approximately 80% of patients will ovulate on CC treatment, while the associated pregnancy rate is only about 40% [3]. The negative effects of CC are ovarian cysts in about 13% of cycles and delayed endometrial development, which subsequently decreases receptivity. CC remains the drug of choice for normoestrogenic anovulation and can be used for mild stimulation.
Letrozole for Ovulation Induction
Letrozole inhibits the aromatase activity in the cytochrome P450 enzyme complex and induces an acute hypoestrogenic state that stimulates the release of FSH. Letrozole also inhibits the spontaneous LH surge. The efficiency of letrozole is dependent on the patient’s body mass index (BMI). Letrozole is found to be teratogenic, embryotoxic, and fetotoxic in animal models [4]. However, studies in humans have demonstrated its safety with regards to the health of offspring [4]. Letrozole is administered at a dose of 5 mg daily, for 5 days, from day 3 of the menstrual cycle.
In recent years, oral drugs, such as antiestrogens, aromatase inhibitors, insulin sensitizers, androgens, and others, have been increasingly used in the context of ovarian stimulation, either alone or in combination with exogenous gonadotropin preparations.
Superovulation for Intrauterine Insemination (IUI)
IUI was the first treatment made available for infertility. The major indications for IUI are mild to moderate male factor, mild to moderate endometriosis, unexplained infertility, and cervical factor. The rationale for performing IUI is to increase the number of highly motile spermatozoa (by its concentration in a small volume of medium and injection into the uterine space) proximal to the site of fertilization. In most cases, ovarian stimulation for IUI is achieved using a superovulation protocol with CC, an E2 antagonist that elevates secretion of endogenous gonadotropins, or with exogenous gonadotropins. Daily doses are adjusted (increased or reduced) every 2–4 days, in accordance with the ovarian response. When the dominant follicle(s) reaches 18–24 mm, ovulation is induced with hCG (an LH receptor agonist). If two dominant follicles are present, the risk of a multiple pregnancy is about 4%. However, if three or four dominant follicles are present, the risk increases to 18% [3]. If the ovulating dose of hCG is not administered, the follicles will usually become atretic. Since the life span of the fertilizable ovum is estimated to be only 12–24 hours, the timing of the insemination procedure may critically influence success rates. There is controversy as to the optimal number of inseminations per cycle. The majority of published series have documented the efficacy of one IUI performed on the day after the LH surge [5]. The procedures were performed on the day after receiving positive LH test results (one insemination) or on the day of the LH surge and the following day (two inseminations).
Immobilization of the women, by maintaining them in a supine position after IUI, has no positive effect on pregnancy rates. Comparable cumulative ongoing pregnancy rates per couple have been found, with no significant difference between the group of women who lay down for 15 minutes only (32.2%) after IUI versus those that did not (40%) [6]. No difference was found in miscarriage rate, time to pregnancy, or live birth rate between the groups.
Uterine contraction and weak or moderate endometrial movement on the day of IUI are preferable for the success of pregnancy. A cervico-fundal direction of movement, consistent with sperm transport, has also been implicated as beneficial for a successful outcome [7]. For this reason, coitus after IUI could be suggested.
The majority of pregnancies in IUI cycles with gonadotropins occur within three (in younger patients) to six cycles (in older patients) of therapy [8]. Pregnancy rates following one versus two IUIs close to ovulation time have been shown to be similar [9]. As in other ART procedures, catheter choice during IUI also does not seem to be a significant factor affecting pregnancy rates [10], which stands at ~18% and live births at ~8% [11–12].
In the case of unsuccessful IUI, the patient is then prepared for IVF, which, in the past, usually began with a pretreatment, which is less commonly performed today. Pretreatment is defined as administration of steroids (combined oral contraceptive pill, P4 alone or E2 alone) before commencing pituitary downregulation in the case of GnRH agonists or before FSH administration in the case of GnRH antagonist protocols. Pretreatment for 5–8 days suppresses natural hormone production and may help synchronize the development of the follicular cohort and aid in IVF cycle planning [13].
The stimulation phase includes the injection of medications for 8–14 days to induce the ovaries to produce follicles. IVF medications often involve self-administration of a combination of injections, patches, and pills. Once the patient is taking medications to stimulate the ovaries, regular blood tests are needed to measure the levels of hormones in the circulation. Because every woman responds to IVF medications differently, hormones must be adjusted on a day-to-day basis to ensure that sufficient follicles are stimulated, and to reduce the risk of ovarian hyperstimulation syndrome (OHSS), which can cause severe morbidity [14]. Other potential side effects include: swelling or rash at the injection site, mood swings and depression, enlarged ovaries, abdominal pain, and bloating.
All treatment regimens used to induce multiple follicular development in IVF programs result in a significant increase in circulating FSH concentrations, either by indirect stimulation of endogenous FSH secretion (e.g., regimens involving CC) or by direct exogenous administration of FSH (e.g., gonadotropin preparations). Although recruitment of a large number of preovulatory follicles (not all the growing follicles develop synchronously) after COH could lead to the retrieval of subpar oocytes [15], several studies have indicated that, for younger patients, the pregnancy rates per cycle after COH are higher than in natural cycles. The cumulative live birth rates are comparable between COH and natural cycles. However, in older patients, COH could lead to retrieval of suboptimal oocytes. Indeed, in older patients (35–42 years of age), COH is associated with lower implantation rates compared with natural cycles.
Human Menopausal Gonadotropins (hMG) for Controlled Ovarian Hyperstimulation
Many stimulation protocols have been introduced for COH of patients undergoing IVF. They include the different stimulation protocols using FSH in combination with GnRH analogs, oral supplementations, and ovarian triggering. Some protocols have shown a better outcome in terms of the live birth rate when extracted highly purified urine human menopausal gonadotropins (hMG), also called menotropins, were used for ovarian stimulation compared with use of recombinant FSH (rFSH) in the GnRH agonist protocol. One of the most efficient stimulation protocols uses a combined protocol of human-derived urinary FSH (uFSH) and rFSH. The combined protocol has brought a significant increase in the proportion of mature metaphase II oocytes and high-quality embryos when compared with either rFSH or uFSH alone. In poor and normal responders, a significantly higher delivery rate was achieved in rFSH + uFSH compared with the other protocols.
Initially, ovarian stimulation was predominantly performed using hMG, showing both FSH and LH bioactivity. However, this bioactivity can vary between different lots of hMG preparation. Differences in biopotency of batches of hMG are consistent with evidence that gonadotropins secreted by the pituitary are released as groups of proteins with some fractions being more bioactive than others. Menotropins are supplied in ampules containing 75 or 150 international units (IU) of FSH and an equal amount of LH per ampule. Purer FSH can be obtained by immunochromatographically isolating LH from urinary preparations of hMG. Variations in the bioactivity of urinary gonadotropins are also reflected by the unpredictable responses of women. hMG treatment is initiated following spontaneous menses or induced withdrawal bleeding, with an initial dose of 150 IU. This same dose is administered daily for 3 days, and then serum E2 is determined. If the E2 level has doubled, the same dose of hMG is continued, with serum E2 monitoring every 1–2 days. Sonograms provide information on follicle number and size. Usually, the first ultrasound is performed when the E2 level approaches 300 pg/ml. Sonograms are then performed every 1–3 days. Follicular growth is linear and usually proceeds at a rate of 2–3 mm each day. In spontaneous ovulation, the mean follicular diameter before ovulation ranges from 20 mm to 25 mm. In hMG-stimulated cycles, the mean follicular diameter ranges from 18 mm to 21 mm (~5 ml of follicular fluid) before ovulation induction with hCG administration [16].
Stimulation protocol with no desensitization of hypophysis: administration of FSH on days 4–6 of the cycle to induce folliculogenesis (not used in young and good responders). Then, FSH + LH from day 7 up to day 12, and then administration of hCG on day 13 with ovum pickup (OPU) by aspiration on day 15 (Figure 6.2).
Figure 6.2 Conventional superovulation strategy for IVF. ET, embryo transfer.
Recombinant gonadotropins.
rFSH was developed to reduce the inherent variability that resulted from the inconsistent starting materials of uFSH production, and to make FSH production independent of urine collection, thus expanding availability. Since glycosylation is essential to the function of FSH, the recombinant molecules must be produced using mammalian cell lines capable of this post-translational modification.
The glycosylation pattern of gonadotropins varies throughout the menstrual cycle, and the desired glycosylation profile of rFSH also had to be selected. After considering the feasibility of producing three FSH preparations with different glycan profiles, matching the glycosylation at the start, middle, and endpoints of the menstrual cycle, the decision was made to use the midcycle glycosylation pattern, as it was the most bioactive. rFSH is pure (99%) and has a more homogeneous glycosylation pattern than highly purified urinary and pituitary FSH or hMG preparations; the manufacturing process allows for high batch-to-batch consistency in both isoform profile and glycan-species distribution. After the successful production of rFSH, a similar process was used to produce recombinant human versions of LH and recombinant hCG.
Further development of gonadotropins has been a process focused on producing purer products that are safer and more efficacious. Biochemical differences from the original product (strength, purity, and composition of isoforms and/or glycosylation profiles) can cause differences in biological activity (including biopotency, receptor binding, postreceptor biochemistry in the cell, pharmacokinetics, and pharmacodynamics). The currently available products can be injected subcutaneously rather than intramuscularly, and pen injection devices are available, eliminating the need to reconstitute the product before injection and improving ease of use compared with syringe and vial. This has led to the current state of the art, where fertility treatment can be personalized using pure gonadotropin preparations at the start of and during ovarian stimulation, with the aim of maximizing safety and efficacy.
Exogenous gonadotropins may contribute to epigenetic changes in four imprinted genes, Peg1, Kcnq1ot1, Zac, and H19 [17], and may impair oocyte and embryo development [18]. The imprinting defects involved may lead to clinical implications in ART, such as failure of the embryo to implant, spontaneous abortion, and/or fetal growth retardation attributed to dysfunctional placentas.
When FSH injections are used, multi-follicular development is encouraged. As multi-follicular development is associated with increased serum E2 concentrations, there are high incidences of a premature LH surge before the follicles have fully matured. To prevent premature LH surge, GnRH analogs (GnRH antagonist) are implemented.
GnRH for Controlled Ovarian Hyperstimulation
The GnRH analogs are synthetic compounds based on amino acid(s) substitution to the originally isolated GnRH (GnRH-I and GnRH-II isoforms) decapeptide. Two types of analog have been synthesized, namely agonists and antagonists. While agonists occupy the GnRH receptors, initially release gonadotropins (flare-up), and downregulate the receptors over a period of time, antagonists block the action of GnRH at its receptors without initial pituitary gonadotropin release. Following an initial stimulation of GnRH receptors by GnRH agonists, the end result, much like with antagonists, is a reversible reduction of endogenous pituitary gonadotropin secretion, leading to a reduction in ovarian steroid production. Dose–response studies indicate that it suppresses both LH and FSH within 10 hours, with the duration of suppression being dose-related.
In the early 1980s, births after IVF were reported after CC-stimulated IVF cycles [19] followed by the use of urinary gonadotropins. In this type of treatment, the ongoing development of multiple follicles coincident with the supraphysiological production of E2, frequently associated with a premature late follicular phase rise in LH and P4, resulted in compromised IVF outcomes. For this reason, the downregulation of endogenous gonadotropin synthesis resulting from the co-administration of GnRH agonists was introduced in the late 1980s and rapidly became standard of care. At first, there were only GnRH agonists available. The duration of ovarian stimulation protocols increased significantly due to the initial stimulatory (so-called “flare-up”) effect of GnRH agonists on pituitary gonadotropin release, in which both serum LH and FSH are increased. The GnRH agonist-induced surge of gonadotropins can last for 24–36 hours [20]. Unfortunately, agonist suppression increased the incidence of OHSS, most likely due to the synchronization of the follicular cohort. Over the last several years, clinicians have slowly shifted away from GnRH agonist co-treatment towards treatment with GnRH antagonists and, today, there are two main COH protocols: the GnRH antagonist protocol and the long agonist protocol. This shift provided multiple advantages over use of agonists, and also allowed agonist trigger of ovulation with an associated dramatic reduction in OHSS.
GnRH antagonist protocol.
GnRH antagonist protocols have been demonstrated to significantly improve the clinical pregnancy rates for expected poor and high responders, and in women at high risk of developing OHSS. Treatment begins on day (D) 2–3 of the menstrual cycle, with daily administration of FSH. On D 5–6, GnRH antagonist is injected (Figure 6.3). The antagonist is designed to prevent premature ovulation that might hinder treatment success. The combined treatment is continued until mature follicles are obtained. Once mature follicles (generally over 17 mm diameter) appear, an hCG injection is administered to induce final maturation of the follicles and oocytes. Although some practitioners begin the antagonist treatment on a predetermined cycle day of stimulation (known as a fixed start), others rely on feedback from each cycle, including the size of the lead follicle, to individualize timing of antagonist treatment initiation (known as a flexible start) [21–22]. The highest clinical pregnancy rates are achieved when the antagonist is started with a lead follicle of 14–16 mm in size and E2 levels between 300 pg/ml and 600 pg/ml [23]. If the response of the ovary is low, the agonist short (flare-up) protocol is recommended.

Full access? Get Clinical Tree
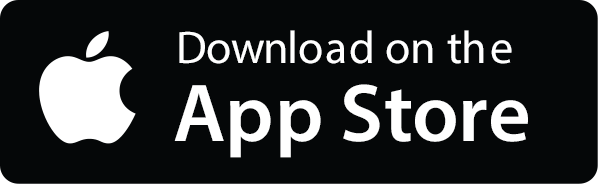
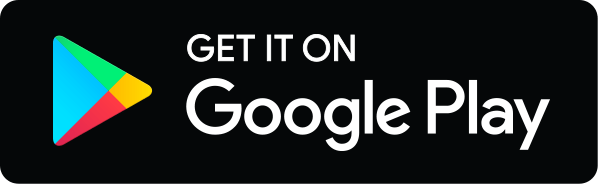