Abstract
Cervical mucus is a regulator of the sperm transfer from the vagina to the uterine cavity. Estradiol (E2) stimulates the production of large amounts of thin, watery, alkaline acellular cervical mucus with ferning, spinnbarkeit (crystallization), and sperm receptivity. Progesterone (P4) inhibits the secretory activity of cellular mucus and produces low spinnbarkeit and absence of ferning, which is impenetrable by spermatozoa. In midcycle, the cervix softens progressively, the os of the cervical canal dilates, and clear, profuse mucus exudes from the external os. In a few days after ovulation, the cervix becomes firm, and the os closes. The cervix is the first barrier for the sperm to overcome.
Conception is the process of becoming pregnant, which involves fertilization of the oocyte by a sperm cell and implantation of the formed embryo in the uterus.
Fertilization
Cervical mucus is a regulator of the sperm transfer from the vagina to the uterine cavity. Estradiol (E2) stimulates the production of large amounts of thin, watery, alkaline acellular cervical mucus with ferning, spinnbarkeit (crystallization), and sperm receptivity. Progesterone (P4) inhibits the secretory activity of cellular mucus and produces low spinnbarkeit and absence of ferning, which is impenetrable by spermatozoa. In midcycle, the cervix softens progressively, the os of the cervical canal dilates, and clear, profuse mucus exudes from the external os. In a few days after ovulation, the cervix becomes firm, and the os closes. The cervix is the first barrier for the sperm to overcome.
After ejaculation, within minutes of vaginal deposition, human sperm cells begin to leave the seminal pool and swim into the cervical canal (22 mm in length) where they could be found in 1.5–3 minutes post-ejaculation [1]. The sperm cells make their way through the uterine cavity (could be less than 10 minutes) into the Fallopian tubes, which have increased secretory activity. The Fallopian tube secretes mucus that results in the sticking of spermatozoa to the epithelium of the tube. Sperm binding occurs at the microvilli of the secretory cells [2]. There is chemotaxis of sperm cells to the cumulus–oocyte complex within the Fallopian tube, due to chemoattractants in the follicular fluid at ovulation time [3], which continue to be secreted by the cumulus-oocyte complex [4]. Peristaltic contractions of the female reproductive tract and cilia movement along the lining of the Fallopian tubes also assist the movement of sperm. Spermatozoa “swim” up the tube (extends about 10 cm) toward the ovum. Despite all this, however, only a small number of the sperm deposited in the vagina ever reach the ovum. Only 25–50 sperm cells out of 250–500 million spermatozoa reach their target.
Fertilization most often occurs in the outer one-third part of the oviduct, named “ampulla.” Sperm cells penetrate the cumulus layer (of approximately 5000 cumulus cells) by cleavage of the extracellular matrix (mostly composed of hyaluronic acid) under acrosomal hyaluronidase and reach the zona pellucida (ZP). Spermatozoa that have completed their acrosome reaction before meeting the cumulus cannot penetrate [5–6]. Receptor molecules on the layer of ZP that surround the ovum bind sperm attracted to the area. Once bound to a ZP2 (sperm-binding ligand), the sperm cell releases enzymes from the acrosome (acrosome exocytosis), becomes incorporated into the ZP, and penetrates ZP rapidly by acrosin, a trypsin-like proteinase of the sperm. Once the spermatozoon reaches the surface of the ovum, the two plasma membranes fuse, and the nucleus of the sperm moves inside the oocyte.
The fusion between sperm and oocyte membranes leads the sperm cell to deliver a putative oocyte activation factor – phospholipase C zeta (PLCζ) – to the oocyte [7]. In addition to that, the sperm cell donates to the oocyte its DNA and centrosome, which contains a pair of centrioles (the oocyte has none). The sperm’s centrioles are essential to organize a centrosome, which will form a spindle enabling the mitotic division of the zygote.
At the moment of sperm and oocyte membrane fusion, PLCζ hydrolyzes phosphatidylinositol biphosphate from the oocyte membrane into inositol triphosphate (IP3) and diacylglycerol (DAG) (Figure 5.1). IP3 interacts with its receptor on the membrane of the endoplasmic reticulum (ER), which allows Ca2+ release from the ER. Mutations in sperm PLCζ may prevent calcium signaling. The rise in Ca2+ increases the action of calcium-dependent protein kinase (PKC), activated by DAG, thus leading to the cortical reaction of the oocyte (activation of peripheral cortical granules; estimated ~8000 granules in the oocyte). The content of these granules is released into the perivitelline space immediately after fertilization (exocytosis) for the establishment of the polyspermy block. Zink sparks from peripheral cortical granules last ~10–15 seconds after cortical granule exocytosis. Cortical granules also release ovastacin, a Zn2+-related metallopeptidase that cleaves ZP proteins (closing of ZP) and membrane to provide a definitive block to the polyspermy. Cortical granules developed from Golgi complexes during oocyte development; however, their total number could be more related to the cytoplasmic maturation rather than nuclear maturation.
Reinitiated meiosis continues events, which lead to extrusion of the second polar body and zygote formation (male and female pronuclear).
A human oocyte contains an estimated ~100 000 – 200 000 copies of mitochondrial DNA (mtDNA), whereas a sperm cell delivers its ~25–75 copies of mtDNA. Paternal mitochondria are rapidly eliminated after fertilization as they are separated from the axoneme and degraded in lysosomes. A sharp reduction in paternal mtDNA content, together with the action of a specific ubiquitination mechanism of mitochondria destruction in the early embryo, explains the absence of paternal transmission of mtDNA to the developing embryo [8].
In as much as the oocyte lives only a short time (probably a day only) after leaving the ruptured follicle, the “fertilization window” occurs around the time of ovulation. Spermatozoa may live up to a few days after entering the female tract, therefore sexual intercourse any time from about 3 days before ovulation to a day or so after ovulation may result in fertilization.
Implantation
Implantation is the process by which the embryo attaches to the endometrium, first invading the epithelium and then forming the placenta. This process is commonly seen as one of the strongest connections in nature (Figure 5.2). Conceptus (embryo/fetus and associated extraembryonic membranes) growth and development require P4 and placental hormone actions on the uterus that regulate endometrial differentiation, uterine receptivity for blastocyst implantation, and conceptus–uterine interactions for implantation. Successful implantation depends on embryo quality and endometrial receptivity. There are three major stages in implantation of the embryo: apposition, attachment (adhesion), and invasion, which are only implemented if three major conditions are fulfilled: a receptive endometrium, a functional embryo, and a functional embryo–endometrial dialog (secretions). The basic characteristics of the processes of attachment and invasion of the embryo to the uterine epithelial surface are common to many species. For the achievement of a successful pregnancy, perfect synchronism between the embryo development stage, changes in the endometrial environment, and conceptus–uterine interaction are necessary [9].
Figure 5.2 The early development of the embryo and its journey throughout a reproductive tract.
The implantation period is associated with changes in endometrial epithelial morphology and receptivity. The epithelial cells of the uterus are of two varieties. The luminal epithelial cells face the lumen of the uterus, while others radiate into the endometrial stroma toward the base of the myometrium to form the uterine glands. These glands secrete a fluid rich in mucins, proteins, enzymes, growth factors, cytokines, and integrins, which all together play the role of an orchestra in implantation. The endometrial epithelium and uterine glands cyclically undergo dynamic remodeling, which is dictated by hormone-driven modification of the endometrium. The human endometrium is receptive to blastocyst implantation only during a very narrow time window in the luteal phase, which begins approximately 7 days after ovulation and lasts less than 2 days. This period, known as the “implantation window,” is the time of maximum endometrial receptivity for implantation [10], which is manifested by the expression of peptides and proteins that can serve as biomarkers of uterine receptivity [11]. Impaired uterine receptivity and embryo invasion are currently considered the major limiting factor for the establishment of pregnancy. It is estimated that suboptimal embryo quality accounts for one-third of implantation failures, while suboptimal endometrial receptivity and altered embryo–endometrial dialog are responsible for the remaining two-thirds [12–13].
The implantation window is characterized by the appearance of membrane apical protrusions, called pinopodes or uterodomes [14], on the cells of the luminal epithelium [15] and expression of full-length luteinizing hormone/human chorionic gonadotropin (LH/hCG) receptor [16]. Pinopodes are P4-dependent organelles that become visible between days 20 and 21 of the natural menstrual cycle [15; 17], persist for 24–48 hours [11; 18], and are observed between days 8 and 10 after the LH surge [19], with variation in the cycle days, on which these organelles form, of up to 5 days between different individuals [17; 20]. The apical surface of endometrial pinopodes participates in the adhesion process of the blastocyst, through mechanisms that are still unclear (Figure 5.3) [20].
Figure 5.3 Receptivity of endometrium. SEM (scanning electron microscopy) of the surface of the endometrial cavity at sequential days of a 28-day menstrual cycle. (A) Day 17: the apical membranes of the endometrial epithelial cells are covered by dense microvilli. (B) Day 19: smooth membranes develop and protrude towards the lumen (developing pinopodes). (C) Day 21: smooth membranes replace microvilli and protrude maximally (fully developed pinopodes). (D) Day 23: short microvilli reappear on the apices and the visible cell size increases (regressing pinopodes).
The embryo must recognize a site susceptible to anchoring and does so by rolling itself over the uterine lumen. The apical cell surfaces of the epithelia contain numerous microvilli, which are covered by a thick layer of glycocalyx (Figure 5.4); the embryo contains several complementary receptors and/or ligands, and these receptor–ligand interactions aid in initial adhesion [21–22]. The attachment–detachment events between the endometrial epithelium and the embryo have very localized effects. The embryo initially attaches to the wall of the uterus via its surface L-selectin, which binds carbohydrates on the uterine wall until it gradually slows to a complete stop. This embryo–uterine interaction was described as “a tennis ball rolling across a surface covered in syrup” [23]. Trophectoderm cells secrete syncytin-1, which may help the embryo stick to the endometrium and which plays a role in implantation and early placental development [24]. At the same time, the endometrium expresses binding proteins, i.e., integrin β3 and cadherin. The embryo is not always successful in attachment but continues making attempts until it locates a site that ultimately allows docking. Once the embryo adheres, it anchors and breaches the luminal epithelium to gain entry. Once the embryo breaches the luminal epithelium, the uterus transforms itself completely into a decidua by a process called decidualization, which involves remodeling of the endometrium that includes secretory transformation of the uterine stroma, an influx of specialized uterine natural killer (NK) cells, the exclusion of maternal T and B cells, and finally vascular remodeling [25]. The decidua is a highly vascularized environment created to house and nourish the embryo. In the decidua, the NK cells, which are generally cytotoxic to major histocompatibility complex (MHC)-null target cells, switch to a molecular phenotype that is less cytotoxic [26], and the numbers of T and B cells are kept to a minimum to avoid inflammation and fetal rejection [26].
Figure 5.4 Implantation.
hCG is an autocrine and paracrine regulator and its interaction with LH/hCG endometrial receptors has been implicated in decidualization [27–28]. hCG is a heterodimeric glycoprotein composed of two subunits, α and β, and shares a common α-subunit with all three pituitary glycoprotein hormones. Their specific bioactivity relates to differences in their β-subunits. LHβ and hCGβ are very similar (82% homology), but differ in terms of receptor affinity and half-life, with that of LH being much shorter, i.e., 21 minutes compared with 12 hours for hCG [29]. Circulating hCG, as well as LH, exists as a mix of isoforms. The sources of the various isoforms appear to differ, produced by the forming blastocyst, chorionic villi, and extravillous cytotrophoblast [30]. In clinical practice, hCG dynamics are used to investigate the early stages of pregnancy, as concentrations normally double every 48 hours in the first 6 weeks of pregnancy [31]. Pregnancy tests detect the presence of the hCG that is excreted in the urine of pregnant women as early as 1–2 days after implantation occurs. One of the major functions of hCG is a luteal rescue from luteolysis [32].
hCG maintains the weight of the corpus luteum (CL) [33], whereas, at the cellular level, hCG also preserves luteal cell size, morphology, and luteal secretions [34]. The steroidogenic function of the CL during early gestation is similar to its function during the menstrual cycle, inducing an increase in circulating P4 and E2. Despite the stimulus of exponentially increasing levels of hCG, the production of P4 by the CL appears to be maintained at a relatively constant level [35]. Increasing trophoblastic production of P4 allows pregnancy to progress independently of the CL [36]. CL peptidergic function, as indicated by relaxin and inhibin A luteal production, is also markedly enhanced and is dependent on hCG stimulation [37]. Relaxin acts on several tissues, including the embryo and uterus, and may optimize maternal–fetal function and maternal adaptations to pregnancy [38].
Endometrial thickness is the most investigated marker of endometrial receptivity and has become a classic example of the limited benefit of means in the context of endometrial receptivity. Endometrial thickness greater than 7 mm (7–14 mm) and endometrial volume greater than 2 ml, with triple line pattern and endometrial blood flow, all measured on the day of hCG trigger injection, is the prognostic profile for endometrial receptivity in in vitro fertilization (IVF) treatment [39]. Histological criteria for endometrial dating can observe a delay in the secretory phase and detect receptivity [40]. Higher clinical pregnancy and live birth rates were observed in women with delayed embryo transfer as manifested by pinopodes formation, compared with women with standard IVF embryo transfer [41]. A higher score of pinopode formation was associated with higher clinical pregnancy rates compared to lower scores [42]. Endometrial receptivity array (ERA) is a molecular diagnostic test based on microarray technology that classifies endometrial biopsies into receptive, pre-receptive or proliferative, based on the expression of 238 selected genes from a tissue sample accepted after endometrial biopsy [43]. Women then undergo personalized embryo transfer (pET), with the frozen–thawed embryo transfer timed according to the receptive status as identified by ERA, however inconvenient the results, for now a day, accepted with ERA and pET [44–45]. A significant association between various Doppler signals in the endometrium and pregnancy rates has been reported; however, its clinical relevance remains uncertain [46].
For endometrial receptivity and implantation, a cross-talk between the blastocyst and the endometrium is essential. This cross-talk is based on the secretion of cytokines and growth factors, cell-derived polypeptides, and proteins that have the capacity to bind to specific cell surface receptors and may act as potent intercellular signals regulating functions of endometrial cells. They regulate cell proliferation, differentiation, and apoptosis, by autocrine, paracrine, and endocrine mechanisms. Cytokines produced by the uterine mucosa and the embryo may play a role in maternal–embryonic interactions, augmenting endometrial receptivity by regulation of the expression of adhesion and anti-adhesion proteins [47]. Among the main cytokines and growth factors that have been implicated in implantation by facilitating endometrial receptivity are: leukemia inhibitory factor, interleukin-1 (IL-1), heparin binding-epidermal growth factor, granulocyte-macrophage colony-stimulating factor-1 (GM-CSF-1), insulin-like growth factor (IGF) binding protein-1, and keratinocyte growth factor [48–54]. Trophoblast invasion is an active enzymatic process. Human cytotrophoblastic cells are invasive because of their capacity to secrete metalloproteinases [55] and collagenase (type IV) that allow the blastocyst to penetrate the wall of the uterus, usually the posterior wall [56]. Embryo cross-talk secretions include interleukins (IL-1, IL-6, IL-10), GM-CSF, IGF-II, and interferon. The embryo/trophoblastic extracellular vesicles contain a variety of RNA and miRNA species that have diverse targets on both epithelial and stromal endometrial cells [57–58]. The target genes of these miRNAs are predicted to mediate cellular activities, such as adhesion and migration, suggesting that embryos could potentially modify the expression of the endometrial genome so that it improves trophoblast adhesion. The cytokine GM-CSF is expressed by epithelial cells of the uterus, reaching a maximum during the mid-secretory phase [59], and promotes implantation competence. The secretion of uterine GM-CSF is induced by hCG [60]; however, the embryonic origin of GM-CSF also exists [61]. GM-CSF deficiency is associated with altered implantation and placental development, elevated intrauterine growth retardation, preeclampsia, and increased miscarriage [62].
Does implantation exist in the uterus only? The embryo can implant outside of the endometrium, including in the Fallopian tube, or peritoneum wall, liver, or other places reached by intensive blood supply needed for implantation. Implantation of the embryo in a location other than the uterus is referred to as an ectopic pregnancy (from the Greek ectopos – “displaced”).
Chronologically, one of the earliest promoters of trophoblast invasion seems to be the inner cell mass (ICM) of the embryo itself. Extracellular vesicles produced by the embryonic stem cell of the ICM can trigger trophoblast invasion. As the blastocyst further develops, with gastrulation, the ICM forms a structure of epiblast (forms the allantois) and primitive endoderm, with two cavities called the amniotic cavity (visualization of amniotic sac by ultrasound at ~5 weeks of pregnancy) and yolk sac (visualization at ~6 weeks of pregnancy). The yolk sac is most important in animals such as birds that depend heavily on yolk as a nutrient for the developing embryo. Because the uterine lining provides nutrients to the developing embryo in humans, the function of the yolk sac is not nutritive. Instead, it has other functions, including the production of blood cells. The amniotic cavity becomes a fluid-filled, shock-absorbing sac in which the embryo floats during development. It is the ICM that eventually forms the tissues of the offspring’s body. The trophectoderm (also known as the trophoblast) forms the support structures. The chorion develops from the trophoblast to become a fetal membrane in the placenta. The chorionic villi are extensions of the blood vessels of the chorion that bring the embryonic circulation to the placenta. The placenta anchors the developing embryo to the uterus and provides a “bridge” for the exchange of nutrients and waste products between the mother and embryo.
Placenta
The placenta is a highly specialized structure that has transient, but very important functions during pregnancy. It is composed of tissues from mother and embryo and functions as an excretory, respiratory, and endocrine organ. During early development, the capacity of trophoblast cells (primitive syncytium) to invade deeply into the maternal uterine tissue, reaching as far as the myometrium, and their ability to remodel the maternal vasculature are a key to ensuring the formation of a functional placenta. The primitive syncytium secretes enzymes that digest and loosen the surrounding decidua, thereby allowing expansion of the primitive syncytium into the ensuing spaces.
Placental tissue normally separates the maternal and fetal blood supplies so that no intermixing occurs, and serves as an effective barrier that protects the developing embryo from many harmful substances. Unfortunately, toxic substances such as alcohol and some infectious organisms can penetrate this protective placental barrier. The nutrient-supplying function of the placenta is its most appreciated role. Defects in placental nutrient transport capacity usually manifest relatively late in gestation, i.e., in the second or third trimester in humans, and result in fetal growth retardation, which is often accompanied by other complications such as pre eclampsia. Gene knockouts causing placental abnormalities in mouse models are significantly more likely to exhibit brain, heart, and vascular system defects compared with knockouts that do not affect placentation [63]. This mouse model revealed that the placenta is morphologically abnormal in about two-thirds of embryonic lethal mutant mouse lines [64]. The causative reasons for this developmental co-association remain unknown.
The placenta is embedded within the decidua, the maternal component of the maternal–fetal interface (the endometrium). At predecidualization differentiation of endometrial fibroblast-like cells into enlarged and granulated decidual stromal cells, and an influx of leukocytes can be observed [65]. During decidualization, there is both fetally and maternally mediated remodeling of the spiral arteries so that the placenta becomes bathed in maternal blood, which facilitates the exchange of nutrients, gases, and waste. Remodeling by fetal trophoblasts and maternal leukocytes results in the dilation of the spiral arteries; this decreases the force and maximizes the volume of the maternal blood bathing the placenta [66]. This remodeling can be divided into stages of trophoblast invasion-independent vascular changes (maternal activation of local decidual artery renin–angiotensin system), vascular remodeling induced by perivascular interstitial trophoblasts (utero-placental arteries within the implantation region are invaded by extravillous trophoblast cells), and trophoblast infiltration of vessel walls (infiltration of the arterial wall by endovascular trophoblast). The utero-placental arteries undergo further dilation, reaching up to several times the original diameter of the lumen. Trophoblast infiltration of the media smooth muscle coincides with a loss of elastic fibers. At the same time, angiogenesis is induced by cytotrophoblasts (via vascular endothelial growth factor and proliferation) and vasodilation is induced by NK cells. The stromal cells of the endometrium respond to all these signals and transform into decidual cells.
The definitive structure of the placenta is composed of villous trees and is established by the third week of gestation. A single layer of contiguous multinucleated syncytiotrophoblasts lines the outermost surface of the human placental villous trees and acts as the major cellular barrier between the fetal compartment and maternal blood. Underlying the syncytiotrophoblast layer are undifferentiated, mononucleated cytotrophoblasts, which are progenitor trophoblast cells which can fuse to replenish the syncytiotrophoblast layer or differentiate into mononucleated extravillous trophoblasts, which are located at the tips of the anchoring villi. During the first trimester, the human placenta is hemodichorial, with two layers of trophoblasts separating the fetal and maternal bloodstreams. As the placenta grows, the underlying cytotrophoblast layer thins and becomes dispersed; thus, the human placenta of the second and third trimesters is essentially hemomonochorial, with only a single layer of syncytiotrophoblasts. The syncytiotrophoblasts also function as the main endocrine cells of the placenta, producing hCG and P4 that support pregnancy [67]. During the first 8 weeks of gestation, syncytiotrophoblast secretion of hCG is required to “rescue” the CL from its impending demise and hCG extends the functional life span of the CL in early pregnancy by the production of P4 [32]. At around 8 weeks of gestation, the luteo-placental shift occurs, as the placenta is producing enough hormones to support pregnancy. At this time, the volume of the CL decreases, and rising concentrations of the placental pregnancy-associated plasma protein-A can be detected in the serum, suggesting increasing placental function [68]. The CL loses its functional integrity between 8 and 10 weeks of gestation.
Extravillous trophoblasts physically anchor the human placenta to the decidua. In the first trimester, extravillous trophoblasts act as a plug for the spiral arteries, thus creating a hypoxic environment by excluding the oxygen-rich maternal blood. During the transition from the first to the second trimester, the extravillous trophoblast plug is eroded, and the intervillous space becomes flooded with maternal blood. The direct contact between maternal blood and syncytiotrophoblasts allows for efficient gas, nutrient, and waste exchange, which is maintained throughout the rest of pregnancy. Because direct contact between the maternal blood and the placenta does not occur until the end of the first trimester, this event distinguishes the early (first trimester) from the later (second and third trimesters) stages of pregnancy.
Placental tissue also has endocrine functions, as it secretes large amounts of hCG produced already in the blastocyst stage. hCG secretion peaks at about 8–9 weeks after fertilization, then drops to a steady low level by about week 16. The function of hCG, in general, is to act as a gonadotropin and stimulate the CL to continue its secretion of E2 and P4. As the placenta develops, it begins to secrete its own E2 and P4, corresponding with a decrease in hCG secretion, which reduces luteal secretion of these hormones. After about 3 months of pregnancy, the CL has degenerated, and the placenta has completely taken over the work of secreting the hormones needed to sustain the pregnancy.
Placental immune system.
In addition to stromal cells, a remarkably large portion (~40%) of the decidua is composed of maternal leukocytes. In the first-trimester decidua basalis (the site of implantation and trophoblast invasion) decidual NK (dNK) cells comprise the majority (~70%) of immune cells, followed by decidual macrophages (20–25%) and T cells (3–10%) [26]. Most of our understanding of maternal leukocytes at the maternal–fetal interface has been determined in mouse studies and correlated to observations in human patient samples. dNK cells are the largest population of maternal leukocytes and a unique tissue-specific population that accumulates at the maternal–fetal interface, where they contribute to decidualization and implantation [69]. The dNK cells of pregnancy are phenotypically distinct from peripherally circulating NK cells. There is a cyclic enrichment of NK cells in the uterus after ovulation, which is sustained during pregnancy. dNK cells produce a vast array of growth factors, angiogenic factors, and cytokines [70]. Through these secretions, they help remodel the decidua and spiral arteries, promote trophoblast invasion, and increase the availability of maternal blood at the implantation site [71]. In patients with unexplained infertility, endometrial biopsies have found substantially fewer NK cells than in fertile women [72].
Decidual macrophages are the primary antigen-presenting cells at the maternal–fetal interface in early pregnancy [26]. Like uterine NK cells, levels of uterine macrophages rise and fall with the menstrual cycle and then increase upon fertilization [73]. Decidual macrophages have many functions during pregnancy. Like dNK cells, they aid in remodeling the spiral arteries and trophoblast invasion [74]. Decidual macrophages are proposed to perform “cleanup” functions by phagocytosing apoptotic trophoblasts, which prevents activation of pro-inflammatory pathways in the decidua [75].
Observational experiments have identified regulatory T (Treg) cells in the human decidua, and cases of human infertility, recurrent spontaneous abortions, and other pregnancy complications have been inversely correlated with Treg cell frequencies or functionality [76].
Maternal tolerance, which permits a mother to carry the fetus to term despite the presence of foreign fetal antigen, is a poorly understood phenomenon that seems to defy some of the basic tenets of immunology. For a successful pregnancy, maternal tolerance must be established, and the failure of maternal tolerance is correlated with preeclampsia and miscarriage [77]. In general, tolerance is mediated by the restriction and modulation of leukocytes that permeate the maternal–fetal interface. Maternal tolerance may occur through species-specific mechanisms. In humans, placental extravillous trophoblasts express human leukocyte antigen-G (HLA-G), a nonclassical MHC molecule, which has been suggested to have a role in the escape of the cells from immune recognition and destruction. HLA-G interacts directly or indirectly, via HLA-E, with specific killer inhibitory receptors (Fas) present on the surface of leukocytes, thereby protecting the fetal cells from maternal immune attacks [78]. dNK cells interact with HLA-G+ invasive trophoblastic cells, which may result in apoptosis of leukocytes [79].
The placenta secretes antiviral molecules that broadly function to restrict viral infections. The human placenta can also actively transport protective antibodies to the fetus. Transplacental passage of maternal humoral immunity in humans begins at week 16 of gestation and increases during the course of pregnancy so that, at term, the fetus has a greater serum concentration of maternally derived IgG than its mother [80].
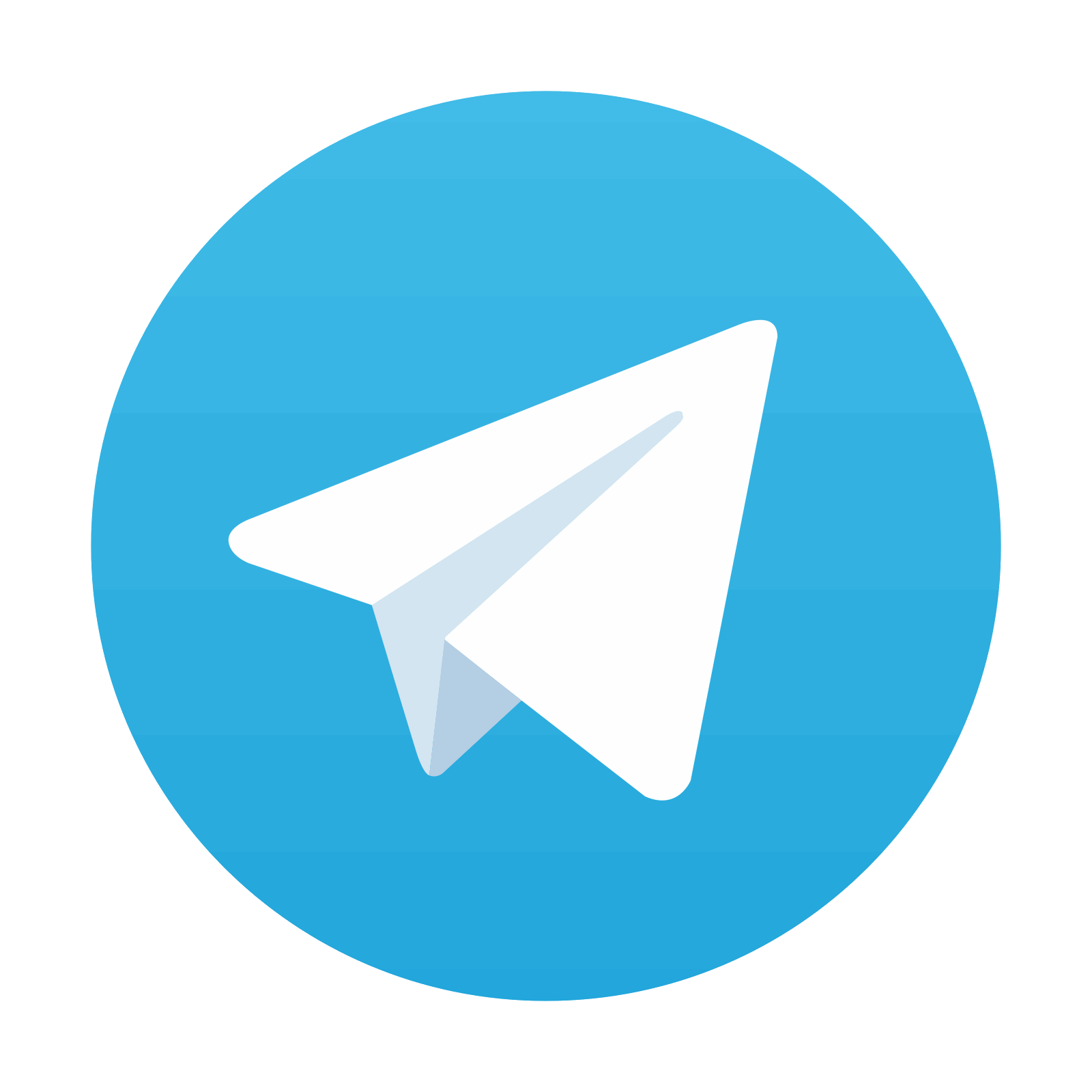
Stay updated, free articles. Join our Telegram channel

Full access? Get Clinical Tree
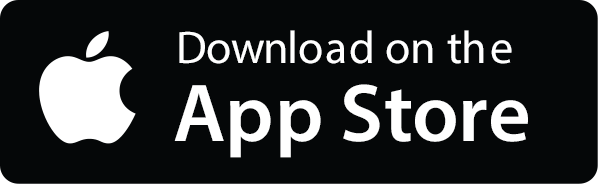
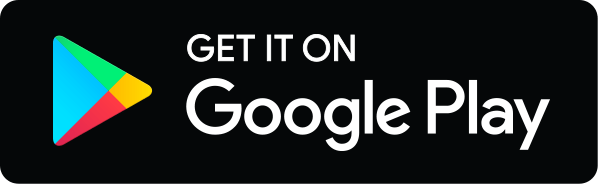
