INTRODUCTION
The menopausal transition is a progressive endocrinologic continuum that takes reproductive-aged women from regular, cyclic menses to a final menstrual period and ovarian senescence. With medical advancements, average life expectancy has increased, and most women can now expect to live at least one third of their lives in the menopause. Specifically, by 2020, approximately 43 million women will be aged 45 to 64 years (U.S. Census Bureau, 2014). Importantly, menopausal transition and the years of life spent in the postmenopausal state bring with them issues related to both quality of life and disease prevention and management (Lund, 2008).
DEFINITIONS
Menopause refers to a point in time that follows 1 year after the complete cessation of menstruation, and the postmenopause describes years following that point. The average age of women experiencing their final menstrual period (FMP) is 51.5 years, but a halt to menses from ovarian failure may occur at any age. Cessation before age 40, termed premature ovarian failure, is associated with an elevated follicle-stimulating hormone (FSH) level and variable causes described in Chapter 16. Of other definitions, the older words perimenopause and climacteric generally refer to the late reproductive years, usually late 40s to early 50s. These can be used with patients but less so in scientific settings. Here, the term menopausal transition (MT) is preferred (Harlow, 2012; Soules, 2001). Characteristically, MT begins with menstrual cycle irregularity and extends to 1 year after permanent cessation of menses. This reproductive aging with loss of follicular activity progresses within a wide age range (42 to 58 years). The average age at its onset is 47, and MT typically spans 4 to 7 years (Burger, 2008; McKinlay, 1992).
As chronological age is an unreliable indicator, guidelines for classifying reproductive aging have been proposed. The first classification of stages and nomenclature for female reproductive aging were developed in 2001 and updated in 2012 at the Stages of Reproductive Aging workshop (STRAW) (Harlow, 2012). These staging criteria are guides rather than strictly applied diagnoses. Every stage may not manifest in all women, or a stage may occur out of the expected sequence. Also, the age range and duration of each stage varies for given individuals.
In the STRAW system, the anchor stage is the FMP (Fig. 21-1). Five stages precede and two stages follow the FMP. Stage –5 refers to the early reproductive period, stage –4 to the reproductive peak, and stage –3 to the late reproductive period. Stage –2 is the early MT, and stage –1 is the late MT. Stage +1a is the first year after FMP, stage +1b reflects years 2 to 5 postmenopause, and stage +2 refers to the ensuing later postmenopausal years.
INFLUENTIAL FACTORS
Several environmental, genetic, and surgical influences may alter ovarian aging. For example, smoking hastens the age of menopause by approximately 2 years (Gold, 2001; Wallace, 1979). Chemotherapy, pelvic radiation, and ovarian surgery may also lead to earlier menopause. During MT, more erratic fluctuations in female reproductive hormones lead to an array of physical and psychological symptoms as outlined in Table 21-1 (Bachmann, 2001; Dennerstein, 1993). Diet, exercise, reproductive history, socioeconomic status, body mass index (BMI), mood, climate, and individual or cultural attitudes toward menopause may explain variations in reports of menopausal symptoms (O’Neil, 2011).
Menstrual pattern | Sexual dysfunction |
Shorter cycles (typical)Longer cycles (possible)Irregular bleeding | Vaginal drynessDecreased libidoDyspareunia |
Vasomotor | Somatic |
Hot flushesNight sweatsSleep disturbances | HeadacheDizzinessPalpitations |
Psychological/cognitiveWorsening PMSDepressionIrritabilityMood swingsPoor concentrationPoor memory | Breast pain/enlargementJoint aches and back painOthersUrinary incontinenceDry, itchy skinWeight gain |
PHYSIOLOGIC CHANGES
In the early MT (stage –2), a woman’s menstrual cycles remain regular, but the interval between cycles may be altered by 7 or more days. Typically, cycle lengths become shorter. Compared with younger women, FSH levels are elevated, and serum estrogen levels may be increased in the early follicular phase. Normal ovulatory cycles may be interspersed with anovulatory cycles during this transition, and conception can occur unexpectedly. The late MT (stage –1) is characterized by two or more skipped menses and at least one intermenstrual interval of 60 days or more due to longer and longer periods of anovulation (Soules, 2001). This overview of altered menstruation results from changes in several endocrine axes described next.
During the reproductive years, gonadotropin-releasing hormone (GnRH) is released in a pulsatile fashion by the arcuate nucleus of the medial basal hypothalamus. It binds to GnRH receptors on the pituitary gonadotropes to stimulate cyclic luteinizing hormone (LH) and FSH release. These gonadotropins, in turn, stimulate the production of the ovarian steroids: estrogen, progesterone, and also inhibin. Estrogen and progesterone exert positive and negative feedback on pituitary gonadotropin production and on the amplitude and frequency of GnRH release. Produced by granulosa cells, inhibin also exerts an important negative influence on FSH secretion from the pituitary. This tightly regulated endocrine system leads to regular, ovulatory menstrual cycles.
Beginning in the late 40s and in early MT (stage –2), FSH levels rise slightly and bring about an increased ovarian follicular response, which raises overall estrogen levels (Jain, 2005; Klein, 1996). The monotropic FSH rise is attributed to decreased ovarian inhibin secretion rather than diminished estradiol feedback. In perimenopausal women, estradiol production fluctuates with FSH levels and can reach higher concentrations than those observed in women younger than 35. Estradiol levels generally do not drop significantly until late in MT. Despite continuing regular cyclic menstruation, progesterone levels during the early MT are lower than in mid-reproductive aged women (Santoro, 2004). Testosterone levels do not vary appreciably during the MT. That said, sex hormone-binding globulin (SHBG) production declines after the menopause and may lead to relative increased levels of free or unbound estrogen and testosterone.
Women in late MT exhibit impaired folliculogenesis and an increasing incidence of anovulation compared with women in their mid-reproductive years. Also, during this time, ovarian follicles undergo an accelerated rate of loss until eventually, in late MT, the supply of follicles is depleted. These changes, including the earlier-described FSH level increase, reflect the reduced capability of aging follicles to secrete inhibin (Reyes, 1977; Santoro, 1996). As another indicator, antimüllerian hormone (AMH) is a glycoprotein secreted by the granulosa cells of secondary and preantral follicles and indirectly reflects the primordial follicle pool (Grynnerup, 2014). Circulating AMH concentrations remain relatively stable across the menstrual cycle in reproductive-aged women and correlate with the number of early antral follicles. AMH levels decrease markedly and progressively across MT (Hale, 2007). With ovarian failure in the menopause (stage +1b), ovarian steroid hormone release ceases, and the negative-feedback loop is opened. As a result, GnRH is released at maximal frequency and amplitude. In turn, circulating FSH and LH levels rise up to fourfold higher than those in the reproductive years (Klein, 1996).
Ovarian senescence is a process that has been shown to actually begin in utero within the embryonic ovary due to programmed oocyte atresia. From birth onward, primordial follicles continuously are activated, mature partially, and then regress. This follicular activation continues in a constant pattern that is independent of pituitary stimulation.
A more rapid depletion of ovarian follicles starts in the late 30s and early 40s and continues until a point at which the menopausal ovary is virtually devoid of follicles (Figs. 21-2 and 21-3). An average woman may experience about 400 ovulatory events during her reproductive lifetime. This represents a very small percentage of the 6 to 7 million oocytes present at the 20th week of gestation, or even the 1 to 2 million oocytes present at birth. The process of atresia of the nondominant cohort of follicles, largely independent of menstrual cyclicity, is the prime event that leads to the eventual loss of ovarian activity and menopause.
FIGURE 21-2
Transvaginal sonographic images of a pre- and postmenopausal ovary. A. In general, premenopausal ovaries have greater volume and contain follicles, which are seen as multiple, small, anechoic smooth-walled cysts. B. In comparison, postmenopausal ovaries have smaller volume and are characteristically devoid of follicular structures. (Used with permission from Dr. Elysia Moschos.)
FIGURE 21-3
Microscopic differences between a reproductive-age and menopausal ovary. A. Reproductive-age ovary. Note preponderance of primordial follicles. B. High-power image of primordial follicles. C. The menopausal ovary shows abundance of atretic follicles and persistent corpora albicans. (Used with permission from Dr. Raheela Ashfaq.)
As evidence, Richardson and colleagues (1987) performed a quantitative histologic study of the endometrium and ovaries of women in MT undergoing hysterectomy for benign indications. These were coupled with a single hormonal measurement and a reproductive history from the study women aged 44 to 55 years. The women who reported regular cycles had an average of 1700 follicles in a selected ovary compared with an average of 180 follicles in the ovaries of those who reported irregular cycles.
With advancing age, adrenal production of dehydroepiandrosterone sulfate (DHEAS) declines. In women aged 20 to 30 years, DHEAS concentrations peak, with an average of 6.2 μmol/L, and then decrease steadily. In women 70 to 80 years of age, DHEAS levels are diminished by 74 percent to 1.6 μmol/L. Other adrenal hormone levels fall with aging as well (Burger, 2000; Labrie, 1997). Androstenedione levels peak at ages 20 to 30 years and then decline to 62 percent of this peak level in women aged 50 to 60 years. Pregnenolone levels diminish by 45 percent from reproductive life to menopause. The ovary contributes to the production of these hormones during the reproductive years, but after menopause, only the adrenal gland continues this hormone synthesis.
Burger and associates (2000) prospectively studied 172 women during MT as a part of the Melbourne Women’s Midlife Health Project. By analyzing hormone levels longitudinally in these patients, no relationship between a woman’s final menstrual period and the decline in DHEAS levels was noted. Advancing age, regardless of menopausal status, determined DHEAS level decline.
Microscopic changes in the endometrium directly reflect systemic estrogen and progesterone levels and thus may change dramatically depending on the stage of MT. During early MT, the endometrium may reflect ovulatory cycles, which are prevalent during this time. During later MT stages, anovulation is common, and the endometrium will display an estrogenic effect that is unopposed by progesterone. Accordingly, proliferative changes or disordered proliferative changes are frequent findings on pathologic examination of endometrial biopsy samples. After menopause, the endometrium becomes atrophic due to lack of estrogen stimulation (Fig. 15-19).
During MT, abnormal uterine bleeding (AUB) is common, and Treloar (1981) found that menses were irregular in more than one half of all women in this transition. Paramsothy (2014) reported that AUB accounted for 14 percent of all hospitalizations from 1998 to 2005 among women aged 45 to 54. Anovulation is the most common cause of erratic bleeding during MT. However, because the time interval surrounding menopause is characterized by relatively high, acyclic estrogen levels and relatively low progesterone production, women in MT are at increased risk for developing endometrial hyperplasia or carcinoma. Estrogen-sensitive neoplasms, such as endometrial polyps and uterine leiomyomas, and pregnancy-related events are also considered. Many women in their late 40s do not consider themselves fertile and will cease contraception but will still have occasional ovulatory cycles. Contraception can be discontinued by all women at age 55. No spontaneous pregnancies above that age have been reported. Some women may still have menstrual bleeding above age 55, but ovulation is rare and any oocytes are likely poor quality and not viable (Gebbie, 2010).
In all women, regardless of menopausal status, the etiology of AUB should be determined as outlined in Chapter 8. As noted, endometrial cancer is suspected in any woman in MT with AUB. The overall incidence of endometrial cancer is approximately 0.1 percent of women in this group per year, but in women with AUB in MT, the risk increases to 10 percent (Lidor, 1986). Thus, endometrial biopsy is done to exclude malignancy.
Although endometrial neoplasia is the greatest concern during this time, endometrial biopsy frequently reveals a non-neoplastic endometrium displaying estrogen effects unopposed by progesterone. In premenopausal women, this results from anovulation. In postmenopausal women, unopposed estrogen may be derived from extragonadal endogenous estrogen production, which may result from increased aromatization of androgen to estrogen due to obesity. In addition, decreased SHBG levels lead to increased levels of free and therefore bioavailable estrogen (Moen, 2004). Less often, unopposed exogenous estrogen administration or an estrogen-producing ovarian tumor can also account for these effects in postmenopausal women.
Of the many menopausal symptoms that may affect quality of life, the most frequent are those related to thermoregulation dysfunction. Kronenberg (1990) tabulated all of the published epidemiologic studies and determined that vasomotor symptoms, also variably termed hot flashes, hot flushes, and night sweats, developed in 11 to 60 percent of menstruating women during MT. In the Massachusetts Women’s Health Study, the incidence of hot flushes increased from 10 percent during the premenopausal period to approximately 50 percent after menses cessation (McKinlay, 1992). Hot flushes begin an average of 2 years before the FMP, and 85 percent of women who experience them will continue to experience them for more than 1 year. Of these women, 25 to 50 percent will have hot flushes for 5 years, and >15 percent may experience them for >15 years (Kronenberg, 1990). More recent studies of duration indicate that women can expect hot flushes to continue, on average, for nearly 5 years after the FMP, while more than one third of women who experience moderate/severe hot flushes will continue to have them for more than 10 years after the FMP (Freeman, 2014). Longitudinal studies show that hot flushes are associated with low exercise levels, smoking, high FSH and low estradiol levels, increasing BMI, ethnicity, lower socioeconomic status, and prior premenstrual dysphoric disorder (PMDD) or depression (Gold, 2006; Guthrie, 2005).
Thermoregulatory and cardiovascular changes that accompany a hot flush are well documented. An individual hot flush generally lasts 1 to 5 minutes, and skin temperatures rise because of peripheral vasodilation (Kronenberg, 1990). This change is particularly marked in the fingers and toes, where skin temperature can increase 10 to 15°C. Most women sense a sudden wave of heat that spreads over the body, particularly the upper body and face. Sweating begins primarily on the upper body, and it corresponds closely in time with an increase in skin conductance (Fig. 21-4). Sweating has been observed in women during 90 percent of hot flushes (Freedman, 2001).
FIGURE 21-4
Physiologic changes (means) during a hot flush. A. Core body temperature. B. Respiratory exchange ratio. C. Skin temperature. D. Sternal skin conductance. Time 0 is the beginning of the sternal skin conductance response. (Reproduced with permission from Freedman RR: Biochemical, metabolic, and vascular mechanisms in menopausal hot flashes. Fertil Steril 1998 Aug;70(2):332–337.)
Increases in both awake and sleep systolic blood pressure are noted with hot flushes (Gerber, 2007). In addition, heart rate rises 7 to 15 beats per minute at approximately the same time as peripheral vasodilatation and sweating. Heart rate and skin blood flow usually peak within 3 minutes of the hot flush onset. Simultaneously with sweating and peripheral vasodilation, the metabolic rate also significantly rises. Hot flushes can also be accompanied by palpitations, anxiety, irritability, and panic.
Five to 9 minutes after a hot flush begins, the core temperature declines 0.1 to 0.9°C due to heat loss from perspiration and increased peripheral vasodilation (Molnar, 1981). If heat loss and sweating are significant, a woman may experience chills. Skin temperature gradually returns to normal, sometimes taking 30 minutes or longer.
The underlying physiologic steps leading to hot flushes remain an enigma, and some dysfunction of the thermoregulatory nucleus of the hypothalamus is likely. This nucleus regulates perspiration and vasodilatation, which is the primary mechanism of heat loss in humans. If exposed to higher temperatures, the nucleus activates these heat dissipation mechanisms. This maintains core body temperature in a regulated normal range, called the thermoregulatory zone. It is hypothesized that women who experience more severe vasomotor symptoms have a narrower thermoregulatory zone than those without symptoms. In these women, minimal changes in core body temperature induce shivering or hot flush.
Various hormones and neurotransmitters modulate hot flush frequency. Of these, estrogens play a vital role. Although there is no clear correlation between the two, estrogen withdrawal or rapid fluctuation in levels, rather than a chronically low estrogen concentration, is suspected (Erlik, 1982; Overlie, 2002). This hypothesis is supported by the fact that women with gonadal dysgenesis (Turner syndrome), who lack normal estrogen levels, do not experience hot flushes unless first exposed to estrogen and then withdrawn from treatment.
In addition to estrogen, Freedman and colleagues (1998, 2014) hypothesized that altered neurotransmitter concentrations may create a narrow thermoregulatory zone and a lowered sweating threshold. Norepinephrine is thought to be the primary neurotransmitter responsible for lowering the thermoregulatory setpoint and triggering the heat loss mechanisms associated with hot flushes (Rapkin, 2007). Plasma levels of norepinephrine metabolites are increased before and during hot flushes. Moreover, research shows that norepinephrine injections can increase core body temperature and induce a heat loss response (Freedman, 1990). Conversely, medications that decrease norepinephrine levels, such as clonidine, may reduce vasomotor symptoms (Laufer, 1982).
Estrogens are known to modulate adrenergic receptors in many tissues. Freedman and colleagues (2001) suggested that menopause-related declines in estrogen levels lower hypothalamic α2-adrenergic receptor concentrations. In turn, a decline in presynaptic α2-adrenergic receptor levels leads to increased norepinephrine levels, thereby causing vasomotor symptoms.
Serotonin is likely another involved neurotransmitter (Slopien, 2003). Estrogen withdrawal is associated with a decreased blood serotonin level, which is followed by upregulation of serotonin receptors in the hypothalamus. Activation of specific serotonin receptors has been shown to mediate heat loss (Gonzales, 1993). However, the role of serotonin in central regulatory pathways is complex because binding at some serotonin receptors can exert negative feedback on other serotonin receptor types (Bachmann, 2005). Therefore, the effect of a change in serotonin activity depends on the type of receptor activated. Of other potential candidates, β-endorphins and other neurotransmitters affect the thermoregulatory center and make some women more prone to hot flashes (Pinkerton, 2009).
Genetic polymorphisms and vasomotor symptom prevalence and severity may also be linked. Some polymorphisms are variants of genes encoding estrogen receptor alpha (Crandall, 2006; Malacara, 2004). Others are single nucleotide polymorphisms involved in the synthesis or metabolism of estradiol or in its conversion to more- or less-potent estrogens. Currently, it is unknown whether these genetic determinants exert their effects centrally or peripherally (Al-Safi, 2014).
In sum, studies suggest that reductions and significant fluctuations in estradiol levels lead to a decline in inhibitory presynaptic α2-adrenergic receptor concentrations and an increase in hypothalamic norepinephrine and serotonin release. Norepinephrine and serotonin lower the setpoint in the thermoregulatory nucleus and allows heat loss mechanisms to be triggered by subtle changes in core body temperature. This current theory of hot flush pathogenesis underlies many of the treatment options discussed in Chapter 22.
Several risk factors have been associated with an increased probability of hot flushes, including early menopause, surgical menopause, race/ethnicity, BMI, a sedentary lifestyle, smoking, and use of selective estrogen-receptor modulators (SERMs). Moreover, women exposed to high ambient temperatures may experience more frequent and severe hot flushes (Randolph, 2005). Of risks, surgical menopause is associated with a 90-percent probability of hot flushes during the first year after oophorectomy, and symptoms can be more abrupt and severe than those associated with natural menopause. Among racial and ethnic groups, hot flushes appear to be more common and more bothersome in African-American than in white women and are more common among white than among Asian women (Gold, 2001; Kuh, 1997; Thurston, 2008). These racial/ethnic differences in vasomotor symptoms persisted even after controlling for key factors such as BMI, estradiol level, hormone use, smoking, education level, and economic hardship (Al-Safi, 2014). The effect of BMI on hot flush frequency is not clear (Da Fonseca, 2013; Hunter, 2012; Wilbur, 1998).
The skeleton consists of two bone types (Fig. 21-5). Cortical bone is the bone of the peripheral skeleton (arms and legs), and trabecular bone is the bone of the axial skeleton, which includes the vertebrae, pelvis, and proximal femur. Peak bone mass is influenced by genetic and endocrine factors, and opportunity in the younger years for acquiring bone mass is brief (American College of Obstetricians and Gynecologists, 2012). Almost all bone mass in the axial skeleton will be accumulated in young women by late adolescence, so the years immediately following menarche are especially important (Sabatier, 1996; Theintz, 1992). Calcium supplementation in prepubertal and pubertal girls improves bone accrual (Bonjour, 2001; Stear, 2003). Accordingly, osteoporosis prevention with weight-bearing exercise and vitamin D and calcium intake ideally begins in adolescence (Recker, 1992). Following adolescence, bone resorption is normally coupled to bone formation such that positive bone balance is achieved when skeletal maturity is attained, typically at age 25 to 35 years. Thereafter, bone mass declines at a slow, steady rate of approximately 0.4 percent each year. During menopause, the rate increases to 2 to 5 percent per year for the first 5 to 10 years and then slows to 1 percent per year. The subsequent risk of fracture from osteoporosis will depend on bone mass at the time of menopause and the rate of bone loss following menopause (Riis, 1996).
Normal bone is a dynamic, living tissue that is in a continuous process of destruction and rebuilding. This bone remodeling, also described as bone turnover, allows adaptation to mechanical changes in weight bearing and other physical activities. The process of bone remodeling involves a constant resorption of bone, carried out by multinucleated giant cells known as osteoclasts, and a concurrent process of bone formation, completed by osteoblasts (Fig. 21-6).
FIGURE 21-6
Bone remodeling. A. Osteoclasts resorb matrix, whereas osteoblasts deposit new lamellar bone. Osteoblasts that are trapped in the matrix become osteocytes. Others undergo apoptosis or form new, flattened osteoblast lining cells. Osteoblasts produce the proteins RANKL and OPG. When RANKL binds to RANK, the receptor on the surface of osteoclast progenitor cells, this promotes those cells’ development, activity, and survival as osteoclasts. This leads to bone resorption. OPG serves as a counterbalance. OPG binds to RANKL and thereby, RANKL is incapable of binding with RANK to promote osteoclast development. Through this mechanism, bone resorption is limited. B. With hypoestrogenism, RANKL production is increased. Excessive levels of RANKL outnumber those of OPG and osteoclast development and bone resorption is favored.
Activated osteoclasts secrete hydrochloric acid and collagen-degrading enzymes onto the bone surface, resulting in bone mineral dissolution and degradation of the organic matrix. After detaching from the organic matrix, the osteoclasts can relocate and begin resorption at another site on the bone surface or undergo apoptosis. Increased osteoclast activity in postmenopausal osteoporosis is mediated by the receptor activator of nuclear factor (RANK) ligand pathway. In this pathway, RANK, RANK ligand, and osteoprotegerin (OPG) are three major components (Table 21-2).
RANK ligand (RANKL)Protein expressed by osteoblasts/bone lining cellsBinds to RANK on osteoclasts Activation of RANK promotes osteoclast formation, function, survivalRANKExpressed by osteoclasts and their precursorActivated by RANKL bindingOsteoprotegerin (OPG)Protein secreted by osteoblasts/bone lining cells Natural inhibitor of RANKLBlocks RANKL-mediated activation of RANK to balance bone remodeling |
Of these, RANK ligand is expressed by osteoblasts (Bar-Shavit, 2007). This ligand binds to the RANK receptor on osteoclasts and osteoclast precursors. Binding promotes osteoclast formation, function, and survival. RANK ligand is the common regulator of osteoclast activity and ultimately of bone resorption. OPG is also secreted by osteoblasts and is a natural inhibitor of RANK ligand. OPG blocks RANK ligand-mediated activation of RANK and thereby limits osteoclast resorption activity. This balances bone remodeling (Kostenuik, 2005). Many different factors can affect osteoclast activity, but RANK ligand is required to mediate their effects on bone resorption. Cytokines and certain hormones stimulate the expression of RANK ligand by osteoblasts and other cells. One negative regulator of this process is estrogen, which limits the expression of RANK ligand from osteoblasts. Another regulator is OPG, a natural inhibitor of RANK ligand that sequesters and neutralizes the effects of RANK ligand.
In healthy premenopausal women, estrogen limits osteoblast expression of RANK ligand. The OPG binds to RANK ligand to further limit its availability to stimulate osteoclasts. The remaining RANK ligand binds to osteoclast precursors, which fuse and form differentiated osteoclasts for bone resorption. This is followed by the appearance of osteoblasts resulting in bone formation. In sum, resorption and formation are balanced in premenopausal women.
In postmenopausal women, decreased estrogen levels lead to increased RANK ligand expression, which may overwhelm the natural activity of OPG. Studies show that estrogen may indirectly inhibit RANK ligand expression and stimulate OPG expression. Thus, the reduction in estrogen levels associated with menopause may lead to increased RANK ligand and decreased OPG. Bone resorption follows, but osteoblasts can only partially fill resorption cavities. This results in a chronic imbalance of formation and resorption, which leads to ongoing bone loss over time. Thus, increased RANK ligand after menopause leads to excessive bone resorption and potentially postmenopausal osteoporosis (Sambrook, 2006).
Osteoporosis is a skeletal disorder that progressively reduces bone mass and strength (typically in trabecular bone) and leads to increased fracture risks. Changes to the microstructure of bone in women with postmenopausal osteoporosis include increased cortical porosity, decreased bone mass, disrupted trabecular architecture, reduced cortical thickness, and lower mineral content of bone. Osteopenia is a precursor to osteoporosis, and the National Osteoporosis Foundation (2014) estimates that more than 10 million Americans currently have osteoporosis and another 33.6 million have osteopenia of the femoral neck.
Fracture is a frequent consequence of osteoporosis. The vertebrae, femoral neck, and wrists are most commonly fractured, and epidemiologic studies estimate that the remaining lifetime risk of common fragility fractures in white women after age 50 approximates 15 percent at each of these sites (Holroyd, 2008; Kanis, 1994). Nearly 1.5 million Americans experience osteoporotic fractures each year. Worldwide, 9 million osteoporotic fractures are estimated per year (Johnell, 2006; Lund, 2008).
Fractures are associated with significant morbidity and mortality, and the risk of dying following a clinical fracture is reportedly twofold higher than for persons without fractures. The overall mortality rate from femoral neck fracture alone approximates 30 percent. In addition, only 40 percent of those who sustain a femoral neck fracture are capable of returning to their prefracture level of independence. As such, clinicians ideally educate patients regarding bone loss prevention, screen to identify bone loss early, and work with patients to implement effective management plans for osteoporosis or osteopenia.
A major proportion of bone strength is determined by bone mineral density (BMD), which is grams of mineral per area and volume of bone. However, bone quality, bone strength, and fracture risk are also affected by bone remodeling rates, bone size and geometry, microarchitecture, mineralization, damage accumulation, and matrix quality. Unfortunately, all of these are more difficult to accurately assess (Kiebzak, 2003).
Primary osteoporosis refers to bone loss associated with aging and menopausal estrogen deficiency. As estrogen levels fall after menopause, its regulatory effect on bone resorption is lost. As a result, bone resorption is accelerated and is usually not balanced by compensatory bone formation. This accelerated bone loss is most rapid in the early postmenopausal years (Gallagher, 2002). If osteoporosis is caused by other diseases or medications, the term secondary osteoporosis is used (Stein, 2003).
The amount of bone at any point in time reflects the balance of the osteoblastic (building) and osteoclastic (resorbing) activities, which are influenced by numerous stimulating and inhibiting agents (Canalis, 2007). As noted earlier, both aging and a loss of estrogen lead to a significant increase in osteoclastic activity. Also, decreased dietary calcium intake or impaired calcium absorption from the gut lowers the serum level of ionized calcium. This stimulates parathyroid hormone (PTH) secretion to mobilize calcium from bone by stimulation of osteoclastic activity (Fig. 21-7). Increased PTH levels stimulate vitamin D production. In turn, elevated vitamin D concentrations raise serum calcium levels by several effects: (1) stimulates osteoclasts to remove calcium from bone, (2) increases intestinal calcium absorption, (3) stimulates renal calcium reabsorption, and lowers PTH production by the parathyroid glands (Molina, 2013).
FIGURE 21-7
Vitamin D metabolism. Provitamin D (7-dehydrocholesterol) in the skin is converted to cholecalciferol by ultraviolet (UV) light. Cholecalciferol and ergocalciferol (from plants) are transported to the liver, where they undergo hydroxylation to form the major circulating form of vitamin D. A second hydroxylation step occurs in the kidney and results in the hormonally active vitamin D [1,25(OH)2D3], also known as calcitriol. This activation step is mediated by 1α-hydroxylase and is regulated by parathyroid hormone (PTH), Ca2+ levels, and vitamin D [1,25(OH)2D3]. The activity of 1α-hydroxylase is stimulated by PTH and inhibited by sufficient levels of Ca2+ and 1,25(OH)2D3. Vitamin D increases bone resorption, Ca2+ absorption from the intestine, and renal Ca2+ reabsorption, but it decreases PTH production by the parathyroid glands. The overall effect of vitamin D is to increase plasma Ca2+ concentrations. This rise in plasma Ca2+ levels inhibits 1α-hydroxylase and favors hydroxylation at C-24. This leads to synthesis of an inactive vitamin D metabolite—24,25(OH)2D3. (Reproduced with permission from Molina PE: Endocrine Physiology, 4th ed. New York: McGraw-Hill; 2013.)
In normal premenopausal women, this series of events leads to increased serum calcium levels, and PTH levels return to normal. In menopausal women, estrogen deficiency creates a greater responsiveness of bone to PTH. Thus, for any given PTH level, relatively more calcium is removed from bone.
BMD is the standard used for bone mass determination and is assessed with dual-energy x-ray absorptiometry (DEXA) of the lumbar vertebrae, radius, and femoral neck (Fig. 21-8) (Marshall, 1996). The lumbar vertebrae contain primarily trabecular bone, and this bone type forms 20 percent of the skeleton. Trabecular bone is less dense than cortical bone and has a faster bone remodeling rate. Therefore, early rapid bone loss can be determined by evaluation of this site. Cortical bone is denser and more compact bone and makes up 80 percent of the skeleton. It is most abundant in the long-bone shafts of the appendicular skeleton. The greater trochanter and femoral neck contain both cortical and trabecular bone, and these sites are ideal for the prediction of femoral neck fracture risk in older women (Miller, 2002).

Full access? Get Clinical Tree
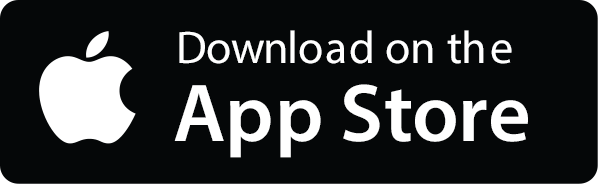
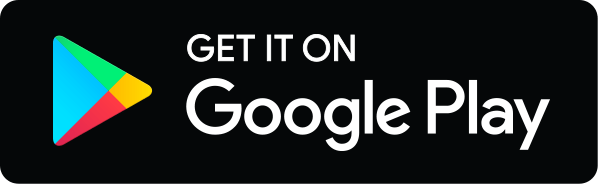