Abstract
Before in vitro fertilization (IVF) was established as a standard treatment for infertility, there was no concern about the laboratory structure and its environment, and how it should be organized. Today, specific standards and legislations exist and the laboratory should also meet international legislations, if cross-border treatments are to be performed. In order to establish a high-quality assisted reproductive technology (ART) clinic, a multidisciplinary skilled task force needs to be formed, to guarantee that all aspects concerning the design of the clinic, selection of equipment and disposables, as well as logistic treatment procedures are taken into consideration.
Structure of IVF Laboratory
Before in vitro fertilization (IVF) was established as a standard treatment for infertility, there was no concern about the laboratory structure and its environment, and how it should be organized. Today, specific standards and legislations exist and the laboratory should also meet international legislations, if cross-border treatments are to be performed. In order to establish a high-quality assisted reproductive technology (ART) clinic, a multidisciplinary skilled task force needs to be formed, to guarantee that all aspects concerning the design of the clinic, selection of equipment and disposables, as well as logistic treatment procedures are taken into consideration.
The IVF laboratory should be adjacent to the operating room where the clinical procedures are performed. Careful attention should be paid to the location of the building in which the IVF laboratory is to be constructed. Also possible sources of particulate and chemical pollution, e.g., parking garages, dry cleaners, foundries, and petroleum processing facilities, should be taken into account [1]. If known pollution sources are identified, this might warrant additional measures to reduce those pollutants within the laboratory environment. In addition, the clinic must actively prevent the exposure of oocytes and embryos to environmental embryotoxic contaminants from within the clinic, building materials, ventilation, laboratory furniture, light and gas sources, disposables, detergents and cleaning agents, and clothing materials; therefore restricted access should be enforced [2–8]. Moreover, the laboratory should provide optimal conditions for gamete and embryo development with high implantation and low miscarriage rates. The workstations should be of the highest quality, preferably antibacterial, easy to clean, resistant to cleaning detergents and disinfectants, and non-embryotoxic. The physical factors of temperature and relative humidity must be maintained within defined limits, and the osmolality, pH, and composition of culture media must be controlled. Critical equipment, including incubators and cryotanks, should be continuously monitored and equipped with alarm systems. An automatic emergency backup power system must be in place for all such equipment. Nicknamed “dirty” rooms should be separated from the ART suite and include gas cylinder rooms, storage rooms for bulk supplies, fixation and staining rooms, office space for laboratory staff, changing rooms, and janitorial closets. The cryobank itself should also be separate from the ART suite, due to the requirement for continuous extraction ventilation. Maintenance of a clean environment in the ART suite is paramount and requires strategies to reduce outside infiltration.
Local fire protection regulations often apply to gas and cryobank rooms, as they contain significant volumes of volatile liquids. As a result, architects might request the presence of firebreak dampers in ventilation ducts. However, this must be avoided to prevent the risk of anoxia or carbon dioxide intoxication in case of accidental closure of ventilation dampers without an alternative fresh air supply.
Staff must use protective laboratory clothing, preferably with low particle shedding. Hairnets and nontoxic, non-powdered gloves and masks should be used, where appropriate. Needles, glassware, and other sharps should be handled with extreme caution and discarded into sharps containers. Equipment should be repaired and annual services must be registered. The most sensitive and vital equipment must be connected to an uninterrupted power supply to avoid disruption of work, recalibration, and damage to the equipment or gametes and embryos.
All laboratory staff must be trained to use standard operational procedures (SOPs) and quality controls (QCs) [9]. Their competency must be routinely tested. Vaccination of all personnel against hepatitis B or other viral diseases, for which a vaccine is available, is recommended. All body fluids (blood, follicular fluid, semen, etc.) should be treated as potentially contaminated.
A wide range of factors can affect the outcome of IVF. We should keep in mind that no two laboratories practice identically [10]; therefore, within the laboratory, the gametes and embryos can be affected by factors associated with the laboratory environment, equipment, contact materials, methodology, and the staff. The patients themselves represent a source of influence, as their own biology affects the potential of their gametes and resultant embryos. Clinical factors, such as the stimulation, oocyte retrieval, and embryo transfer procedures and luteal phase support, also influence outcomes.
Air and Ventilation
Air supply vents should be located in the ceiling and return ducts should draw from close to floor level. The effectiveness of the clinic’s heating, ventilation, and air conditioning (HVAC) system (air change effectiveness, contamination reduction effectiveness, controlling particulates, microorganisms, and volatile organic compounds [VOCs] within the critical areas) should be evaluated; perforated diffusers ensure effective mixing of the inlet air and a cleaner environment. An effective air filtration system is essential for achieving optimal laboratory performance, and can bring a significant decrease in VOC and aldehyde concentrations. Not all VOCs are toxic to gametes and/or embryos; examples of nontoxic VOCs include silicones, which are used in all incubator gaskets and tubing, and high-molecular-weight alkanes, such as paraffinic oil, which is stable and nonreactive, and is in use to cover medium with gametes/embryos. A range of organic compounds are typically found in ART laboratories, including d-limonene and α-pinene, common ingredients in colognes and cleaning products, which are highly oil-soluble and hence should not be used, but because they are unreactive under the conditions of culture, they do not seem to be biologically significant contaminants. Ethanol, however, which is one of the two most common contaminants in ART facilities, can be metabolized into acetaldehyde, and isopropyl alcohol can be metabolized into formaldehyde, both of which are biologically detrimental. According to the Hazardous Substance Data Bank, many of the aldehydes commonly found in ART laboratories, e.g., formaldehyde, acetaldehyde, propionaldehyde, butyraldehyde, benzaldehyde, and n-hexaldehyde, are known carcinogens and mutagens. Thus, among modern ART laboratories, i.e., those built using clean-room concepts, the mean and upper 95% confidence limit for total VOC levels are 339.5 μg/m3 and 1213.9 μg/m3, respectively [11]. Medium density fiberboard (MDF) is a significant source of formaldehyde and should not be used in ART laboratory cabinetry. The fixation solution for pathology biopsy samples (10% formalin) can also be a source of formaldehyde. Styrene, the monomer used in the production of polystyrene, can also be found in ART laboratories because of incomplete polymerization during the manufacturing process. Release of styrene molecules occurs when new packages of plasticware are opened.
The olfactory method, i.e., sense of smell, is not a reliable analytical method to detect organic compounds. The smell of odor is susceptible to inter-individual differences in sensitivity, it is highly dependent on the substance and the amount in the environment, and the odor threshold for some substances exceeds the potential level of toxicity in an ART environment. Methods to clean air and reduce VOC levels include centralized units or free-standing towers with activated carbon, potassium permanganate, photocatalytic oxidation (system that works with titanium oxide), high-efficiency particulate air (HEPA) filter, and positive air pressure. Installation of an independent active ventilation system is recommended.
Old IVF laboratories which moved to new clean-room facilities benefited from an increased proportion of high-quality embryos [12]. Laboratories with a carbon air filter and HVAC central filter system showed decreased VOCs and improvements in laboratory performance [13–14].
Generally accepted standards for air quality are fewer than 352 000 particles larger than 0.5–10 µm per m3 (equivalent to <10 000 such particles per cubic foot), fewer than 10 cfu/m3, and fewer than two spores/m3 “at rest.” Total VOCs should be lower than 500 µg/m3 (~400–800 ppb total VOC, depending on molecular species) and lower than 5 µg/m3 aldehydes [11].
Air change of a minimum of 15 total air changes per hour are necessary for IVF laboratories, including 3 fresh air changes per hour, i.e., 20% outside air. Recommended air movement out of the room is at minimum positive pressure of 15 Pascal, with an ideal target of 30–50 Pascal. This can be attained through a cascade of overpressure across several rooms, e.g., external space to access vestibule to IVF laboratory, or recovery area to access vestibule to procedure room to IVF laboratory, to avoid too great a differential pressure between immediately adjacent rooms. A double-door pass-through between the IVF laboratory and the procedures room (if the procedures room is not as clean as the IVF laboratory) must be air-tight to preserve room air pressure differentials and reduce the number of new materials being brought directly into the laboratory.
Most benches come with thick, high-efficiency particulate air – HEPA (99.99% efficiency in removing 0.3 µm particles) – or ultra-low particulate air – ULPA (99.999% efficiency in removing 0.1–0.3 µm particles) – filters of a high quality that do not easily block and can therefore be used for a very long time before the filter must be replaced. HEPA, carbon, and VOC filters improve air quality [15] and provide the workstation with a clean-room environment, which improves IVF success [16]. The prefilters on top of the benches and the large HEPA/ULPA filters should be easily exchangeable. When a new bench is installed, the distributor must ensure that the filters have not been damaged during the transportation. Some benches can also be fitted with activated carbon filters for partial removal of VOCs.
Laminar Flow Workstation
The vertical laminar flow is the most commonly used workstation within the ART laboratory. The biological safety laminar flow (Class II) protects both the product and operator. Ventilation speed should be controlled for optimal efficiency. If not controlled, there is no guarantee that the work area meets the standards necessary for ensuring culture sterility during the various procedures.
The laminar flow cabinet should run continuously, to ensure the cleanliness of the laboratory. If a bench is switched off and started up again, it initially (15–20 minutes) releases a substantial amount of debris that contaminates everything within the bench. Moreover, turbulence is induced behind each object, which increases the risk of contamination. All this can be avoided with programmable benches, where turn off and on times can be programmed so that all parameters (temperature and ventilation control) are at optimum when work begins in the morning.
A high-quality stereomicroscope (binocular), built-in in a laminar flow cabinet, is commonly used to identify and handle cumulus–oocyte complexes, and for evaluation and selection of embryos for embryo transfer and cryopreservation. The binocular should be fitted with an adjustable ergonomic tri-ocular tube, so that a camera can also be connected to a TV monitor, within or outside the cabinet. The space between the light source and the objective should allow easy handling of dishes with gametes, zygotes, and embryos; a start point of 0.7–0.8 magnification objective is recommended. The combination of a high-quality stereomicroscope, a camera, and a light source with angled and adjusted mirrors allows a quality picture displayed on a high-resolution screen to be obtained, enabling evaluation of organelles as small as pronuclei and vacuoles. It is very important that the laminar flow cabinet has dampers, to avoid vibrations. Positioning of the high-resolution screen on the outside of the cabinet allows for dual control and evaluation, and is useful for teaching purposes.
Integrated built-in mini-incubators, with individual small doors and a gas inflow purge, for quicker recovery and better maintenance of the culture conditions, are recommended.
The heated surface should provide an equal temperature over the entire surface area. The temperature of all heated surfaces of the laminar flow cabinet must be calibrated with a sensitive temperature probe measuring the most commonly used dish, and not the surface of the bench. In some cabinets, the surface is coated with a silver-ion-impregnated antibacterial/antimicrobial coating, which prevents growth of bacteria and reduces the risk of contaminations.
Cleaning is performed at the end of the day, with special embryo-tested cleaning detergents. However, in between patients and if spillage occurs, the surface is wiped and then rinsed with water for injection and finally cleaned with a detergent. If the surface becomes sticky or greasy from the detergent, it should be cleaned again with water for injection.
ICSI Workstation
A micromanipulator should be installed on an anti-vibration table. During handling and injection of oocytes, it is advisable to use MOPS- or HEPES-buffered media, with an oil overlay. Exposure of oocytes to microscope light of the micromanipulator should be minimized during intracytoplasmic sperm injection (ICSI) or other procedures.
Incubators
Traditional “big-box” incubators, whether they are carbon dioxide-only or tri-gas, for reduced oxygen, regulate the gas levels by the addition of carbon dioxide and nitrogen. Most laboratories average an oxygen calibrate between 4.5% and 5.5%; as for carbon dioxide, between 5.5% and 6.5% [10]. Clearly, this type of incubator is susceptible to the inclusion of VOCs that are circulating in the laboratory. In contrast, modern benchtop incubators include smaller individualized chambers to help maintain stability of the culture environment, and generally function by flushing the incubator chambers with gases, either premixed in outsourced cylinders or mixed inside the benchtop incubator [17]. As the incubator flushes the chambers with the mixed gas, any ambient room air is flushed out of the chamber, thereby leaving the tanks and in-line filters as the only source of contaminants inside the incubation chamber [11].
The connecting gas tube must be of nontoxic and VOC-free material, leak proof, and easily fitted to the gas reduction inlet valve. The concentration of carbon dioxide must be calibrated (~5–6%) to that of the clinic’s choice of culture media and the concentration of oxygen should be low, between 4.5% and less than 5.5% [10], although 5–6% oxygen is also tolerated [18]. This low oxygen concentration, compared with that of natural air oxygen (~21%), has been shown to ensure the development of high-quality embryos [19–20], and high implantation rates, clinical rates, and live birth rates [21–22]. Since the incubator door (in traditional, large incubators) is frequently opened, the culture conditions are never optimal, which affects the outcomes of the treatments. Such external disturbances have been reduced by table-top incubators which have individual chambers for each patient.
Minimal handling and removal of dishes from the confines of the incubator results in more stable gas levels (culture pH) and temperature. Furthermore, reduced handling helps avoid potential sheer stress caused by pipetting, and other risks associated with transporting embryos across the laboratory or between dishes.
Many of these new-generation incubators lack humidity. The aim of removing humidity in modern embryo culture incubators, including most time-lapse systems, is to reduce contamination associated with moisture in the incubator. However, if conditions are not optimized to avoid loss of humidity, evaporation of culture media may occur [23]. Embryo culture for 5 days in a single-step medium with no replacement demonstrated that use of dry chambers yielded lower day 3 embryo development, lower blastocyst formation, and reduced clinical pregnancy and implantation rates compared with use of humidified chambers [24–25]. Non-humidified conditions could be prevented by adding a plate with sterile water to each chamber with achievement of humidity levels around 40–45% [26]. The amount of oil that sits above the medium may prevent evaporation; it has been shown that 3 ml of oil results in more evaporation compared with 5–7 ml in the same sized dish [27]. The type of oil also affects the evaporation rate, with heavier and denser oil more effectively reducing evaporation than lighter oil; a difference in density of 0.04 g/ml was shown to have a significant effect on evaporation [28]. Nomenclature of many commercial IVF oils is unclear, and it is therefore difficult to determine the densities of commercial embryo culture oils for comparison. Therefore, when media evaporation occurs, the embryos are not exposed to the same components or concentrations of media solvents as originally designed. For example, recommended starting osmolality should be in the range of 255–265 mOsm/kg [29]. Osmolality exceeding 300 mOsm/kg inhibits embryo development. Evaporation of media during culture in dry incubators varies with media drop size. Media evaporation can also occur in modern embryo culture dishes made for time-lapse culture incubators. Use of different dishes yields differing amounts of evaporation.
Incubators are the main source of magnetic fields in a laboratory. Most people do not differentiate between static magnetic fields (those coming from earth) and dynamic magnetic fields (those induced by electric currents going through wires). Works which studied the effect of both types are limited, with conditions either extremely low or high (high expression of magnetic field in Gauss is small when it is expressed in Tesla) compared with those naturally encountered in the laboratory. The statement that reproductive cells are more sensitive to electro pollution remains therefore more a concern than a proven fact.
Time-Lapse Imaging Incubators
Embryo morphology is not always a robust and absolute indicator for implantation potential as, sometimes, the best looking blastocyst fails to produce pregnancy, while a morphologically suboptimal embryo can develop into a healthy baby. Finally, on average, only one-third of all cycles results in a pregnancy [30].
Morphological evaluation of the embryos at specific time points has been the method of choice for embryo selection for decades [31] and remains the primary line of embryo assessment during IVF cycles. Historically, morphological evaluation included the measurement of features, such as pronuclear size [32], multinucleation in early cleavage stages [33], blastomere fragmentation [34], or blastocyst morphology [35]. However, this evaluation method poses limitations, not only arising from the subjectivity of the embryologist, but also because of the evaluation system itself, which views embryo development statically. Moreover, this approach usually requires the physical removal of the embryos from the incubator, and exposes them to fluctuations in temperature, pH, and oxygen levels. Embryo evaluation is typically based on a few discrete time points, leaving the events occurring in between the observations largely unknown; the status of an embryo can change markedly within just a few hours [36]. While multiple observations increase the robustness of embryo evaluation, they impose multiple disturbances to the culture environment, possibly stressing the embryo and reducing its potential to develop and implant. These limitations can be overcome with time-lapse systems, which enable assessment of dynamic changes of embryo morphology occurring during the preimplantation period of a 5-day culture. The key advantages of time-lapse technology include the provision of an improved culture environment based on embryo evaluation without removal from the incubator, and collection of objective and accurate algorithm information about the kinetic and morphological changes and abnormalities an embryo undergoes in vitro [37]. Use of a time-lapse system has led to a 20% improvement in pregnancy rates [38–39].
Analysis of initial events of embryo development revealed that pronuclei breakdown occurred significantly later in embryos that resulted in a live birth, and never earlier than 20 hours 45 minutes after fertilization [40]. It was observed that ability to reach the blastocyst stage could be predicted by the timing of early developmental stages: first cytokinesis 0–33 minutes, a time interval between first and second mitoses of 7.8–14.3 hours, and a time interval between second and third mitoses of 0–5.8 hours [41]. Two- to five-cell cleavage timing and intervals during two cleavages (t5 – the timing of the cleavage to five cells, and s2 – duration of the transition from a two-cell to a four-cell embryo, cc2 – duration of the second cell cycle) were shown to be the most predictive parameters for embryo viability and implantation [36; 38]. An association between aneuploidy and irregular division, start time of cavitation (tSB), expansion (tEB), tEB-tSB interval was reported [42–43]. Furthermore, presence of at least two abnormal cleavage features was associated with a poor prognosis for embryo chromosomal status [42]. Using morphokinetics, it has been possible to demonstrate associations between various cleavage-stage kinetic parameters and the ability of the embryos to reach the blastocyst stage [44]. Blastocyst transfer yields higher implantation rates than transfers at the cleavage stage, but this outcome must be balanced against the possible disadvantages of longer culture, such as the risk of canceled cycles and concerns over the possible epigenetic effects of prolonged in vitro culture [45].
The Eeva (early embryo viability assessment) test is facilitated by dark-field microscopy and cell-tracking software algorithms (based on P2 [t3-t2] and P3 [t4-t3], which categorize embryos into groups with either high or low likelihood of forming “usable blastocysts”). Eeva significantly improved the specificity and the positive predictive value of usable blastocyst predictions compared with morphology evaluation alone [46].
Observation by time-lapse imaging showed that embryos with complex aneuploidies presented delays on the first two cleavages as well as prolonged transitions between the two- and four-cell stages [47]. In some countries where preimplantation genetic testing for aneuploidy (PGT-A) is not permitted, usage of time-lapse systems provides key information on embryo development supplying a cheaper, faster, and less invasive means of evaluating embryo ploidy status compared with PGT-A [48].
Significance of the time-lapse system as a benefit regarding embryo ploidy and live birth is still a contentious issue. When no differences in the ploidy at early stages of embryo development are determined, the initiation of cavitation and the timing of the formation of a full blastocyst have been defined as parameters relevant to ploidy [48]. While most studies found significant morphokinetic differences between euploid and aneuploid embryos, none of them provided sufficient evidence to recommend the clinical use of time-lapse technology for embryo ploidy assessment [49]. It was also suggested that investing in time-lapse systems and changing the daily routine would not lead to clinical benefits [50–51], and there are currently no high-quality data to support the clinical use of this technology for selection of preimplantation embryos [52–53]. Existing data do not yet provide any certainty on the improvement in live birth rates using embryo selection by time-lapse monitoring. However, studies based on large sample sizes, which include several centers from different countries, strongly support time-lapse monitoring as a strategy for embryo selection. Nevertheless, general conclusions cannot be made at the moment, as the studies were carried out in selected populations and the quality of some studies included can be questioned [54]. To augment general concerns, time-lapse incubation and conventional incubation have shown similar cumulative live birth rates [55]. The use of techniques designed to maximize the number of live births and minimize the suffering of women who undergo failed embryo transfers is a moral obligation.
Time-lapse technology has significant relevance for other laboratory activities. For example, differences in embryo morphokinetics, detected by time-lapse technology, may be valuable endpoints for determining the compatibility of consumables, cryopreservation protocols, and devices introduced in the IVF laboratory [56].
Gas Supply
Gas cylinders should be located outside the laboratory. There should be an automatic change-over system and sufficient cylinders stocked for immediate replacement. High-purity gas and in-line HEPA and VOC filters are highly recommended [57]. It might be helpful to insist that IVF laboratories purchase new cylinders from the gas supplier, for their exclusive use, thereby assuring that they are relatively clean. When refilling, the supplier should confirm that the same cylinder was not used for acetylene, for example, before it was filled with carbon dioxide. The compressed gases may contain high levels of organic contamination. It is therefore essential that the gas quality be of the highest purity (medical grade for both nitrogen and carbon dioxide) and has the lowest concentration of contaminants [58]. For many gas companies, “medical grade” only means that the cylinders have only been used for medical gases at medical facilities, and never used for industrial gases or at industrial locations. Many clinics also pre-clean the inlet gas via an in-line HEPA, charcoal, and potassium permanganate filter, which can minimize levels of contaminants that reach the incubator before it is heated and humidified. Evidence is lacking regarding the effectiveness of the different types of in-line filters or the frequency of change-out (monthly, quarterly, or even less frequent changes being recommended). Polyvinyl chloride (PVC) was found to be the most detrimental material to gametes and embryos [59]. However, polytetrafluoroethylene (PTFE)-Teflon gas tubes and phthalate-free manufactured products (e.g., Tygon) are not detrimental to gametes and embryos. Gas supply lines can be made of stainless steel, copper (braised, not soldered), or Tygon.
The gas rooms for compressed gases and liquid nitrogen (LN2) should be separate from, but ideally adjacent to, the ART laboratory suite. All gas lines into the IVF laboratory suite must be sealed at the piping–wall interface. Adequate ventilation and low oxygen alarms should be installed. Protection devices (e.g., glasses, face shield, cryo-gloves, apron, footwear) should be used during LN2 handling. All staff dealing with LN2 should be trained in safety aspects of its use.
Oil Overlay
Oil overlay is commonly used to reduce loss of carbon dioxide and evaporation of medium drops, and to minimize humidity and temperature deviations of cultures when removed from the incubator for procedures. Although shown to be effective for the latter two points, oil overlay has been shown to have only a negligible impact on preservation of carbon dioxide levels, as it has been shown to cause substantial slowing of medium re-gassing [60].
Once VOCs penetrate the culture, it is difficult to remove them, but it is possible to reduce their concentration. VOCs can be either hydrophobic, e.g., benzene, styrene, or hydrophilic, such as ethanol, acrolein, formaldehyde, and glutaraldehyde. Hydrophobic VOCs can partition into the embryos’ membranes as they are also oil-like phases. Overlaying the culture medium with oil results in the preferential partitioning of hydrophobic VOCs into the oil phases, thereby reducing their concentration in the aqueous medium [11]. In regular culture dishes, the surface area of the exposed oil is large compared with the depth of the oil, favoring rapid dissolution of air VOCs into the oil. Dissolution of carbon dioxide through the oil into the medium could be affected in a similar manner, likely explaining the need for preincubation to achieve medium pH equilibration [60].
Virus Contamination via LN2
Virus transmission from one sample to another via LN2 containers is one of the most disputed risks, the potential hazard of contamination and disease transmission through cryopreservation. Viruses cannot “jump out” of the solidified drop of a frozen sample (sperm, oocytes, or embryo) to the liquid phase during storage in LN2. No virus transmissions were reported in over 600 000 cryopreserved samples of IVF cases in 40 countries over the last 20 years. Not a single report of infection supposedly caused by LN2-mediated disease transfer has been reported in human. Only one report of disease transmission in bovine has been documented [61]; however, this finding cannot be clearly attributed to the applied cryopreservation and storage conditions. Under experimental settings, such possibility of infection transmission exists [62]. As an alternative to the LN2 phase, the vapor phase of LN2 has been suggested as a safer method for the storage of gametes and embryos.
Laboratory Walls
The walls of the IVF laboratory must run from the concrete floor up to the underside of the concrete of the floor above (often described as “slab-to-slab”), and all perforations must be completely sealed. Suitable materials for walls are true clean-room modular panels with powder-coated antiseptic (with silver ions), metal, gasketed interfaces, or plasterboard coated with VOC-free paint. Standard paints used to seal the wall and ceiling surfaces in laboratories may be a significant source of VOCs. Low-VOC or VOC-free paints are now available [63].
Laboratory Floors
Sheet vinyl with impervious sealed joints must be used for floors. PVC with a metal grid for antistatic floorings is designed for premises where an electrostatically conductive design is required. They have high chemical resistance, suitable for underfloor heating, ease of maintenance, and resistance to the effects of chairs. In areas in which large volumes of LN2 are used, a non-thermally fragile floor covering, such as a stainless-steel tread plate, should be considered. Polished concrete and epoxy (VOC-free) floor coating are also flooring options. They are low maintenance and very durable (in terms of LN2).
Countertops
Nonporous materials that do not release VOC should be used for countertops. Suitable materials include epoxy, Corian, and Trespa. Use of manufactured wood products, such as MDF, linoleum, or oil-based paints is not recommended, as they have all been demonstrated to be embryotoxic [11].
Desktop computers in operation are known to emit VOCs and formaldehyde. A minimum number of computers should be used in the ART suite and they should be switched off when not in use [11].
Laboratory Lighting
All light fittings are recommended to be of yellow light. Warm white light has a yellow-white color, which is less damaging to embryos than cool white light. Light emitted at 400–500 nm (blue light) appears to be more harmful than longer wavelengths (green, orange, or red light) of visible light, and results in oxidative stress [64]. The time-lapse systems with 625–636 nm (using a red LED) combined with very short exposure times reduce stress on the embryo. More than 90% of the light on the embryo is from microscopes. The light tube should be positioned so it can easily and safely be replaced when needed.
Temperature
Working temperature in the laboratories should be stable and maintained in a range comfortable to the staff, typically within the range of 20–24°C (68–73°F), depending on the region. Keeping the temperature within a narrow range facilitates equipment calibration and operation. The total heat output of the laboratory equipment and staff must be considered. Adequate provision must be made for heating and cooling of the incoming fresh air according to local climatic requirements.
While a static (rather than dynamic) core temperature of 37°C in the incubators and on work station surfaces is not optimal, it has become mainstream. In vivo temperature of the reproductive system is biphasic, increasing in the luteal phase; with the caudal region of the oviduct 1–2 degrees cooler than the cranial portion [65]; i.e., in vivo the embryo is subjected to fluctuation of temperature. That would obviously still require close monitoring of equipment temperature. It is known that differences exist in microscope stage warmers and incubators. It is possible that some heat retention devices or practices in successful clinics are performing more optimally than others. It is also known that display temperatures should not be relied upon and incubators should be measured by an external standard [17]. All traditional disposable plastic dishes do not allow the base of the dish to come into direct contact with the microscope stage, so there is always an air gap. Because air is a poor conductor of heat, this air gap greatly reduces the efficacy of heating stages, allowing the medium in dishes to cool below the temperature at which the heated surface is set.
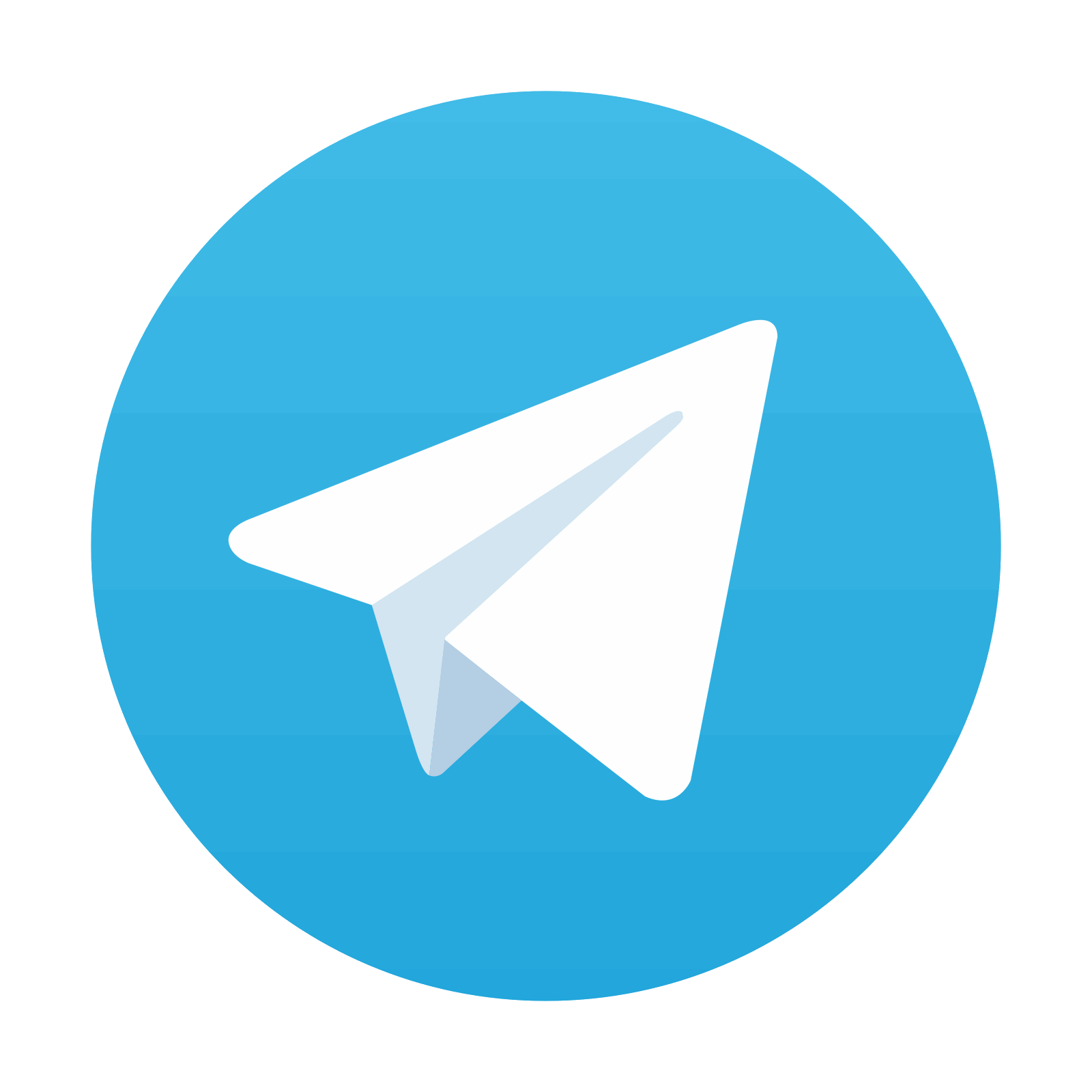
Stay updated, free articles. Join our Telegram channel

Full access? Get Clinical Tree
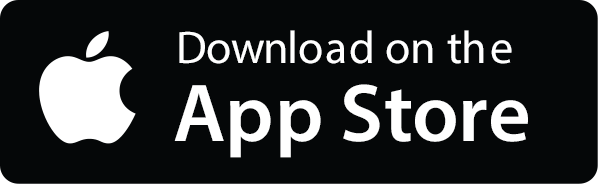
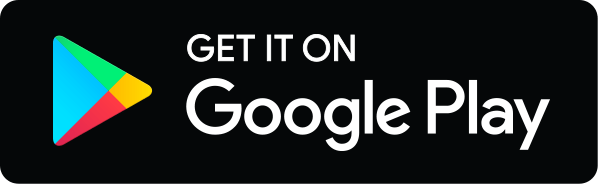