Abstract
The risk for transmission of genetic disorders to offspring has been a critical obstacle for couples known to be carriers for severe genetic disorders. To prevent the birth of an affected child, prenatal testing by amniocentesis or chorionic villus sampling (CVS) is offered. An alternative approach, available with assisted reproductive technology (ART), is preimplantation genetic testing – PGT – enabling testing of the embryo for the familial disorder before the corresponding embryo is transferred to the uterus of the mother.
Introduction to Preimplantation Genetic Testing
The risk for transmission of genetic disorders to offspring has been a critical obstacle for couples known to be carriers for severe genetic disorders. To prevent the birth of an affected child, prenatal testing by amniocentesis or chorionic villus sampling (CVS) is offered. An alternative approach, available with assisted reproductive technology (ART), is preimplantation genetic testing – PGT – enabling testing of the embryo for the familial disorder before the corresponding embryo is transferred to the uterus of the mother.
The concept of PGT (until recently termed “preimplantation genetic diagnosis – PGD”) emerged in the early 1960s when sexing of rabbit embryos at the blastocyst stage was attempted [1]. The first clinical applications of PGT were reported in 1990, with the first pregnancies obtained following blastomere biopsy and sex determination by polymerase chain reaction (PCR) in couples at risk of having children with X-linked disorder [2]. Since then, the list of diseases for which PGT has been used is rapidly growing, and thousands of children have been born following intracytoplasmic sperm injection (ICSI)-PGT cycles, with no identified adverse neonatal, obstetrical, or cognitive risks. Blastomere biopsy for PGT has no added adverse effects compared with ICSI pregnancies [3–5].
Initially, the indication for PGT focused on preventing the birth of a child affected with a severe familial disorder associated with a monogenic mutation or chromosomal rearrangement, carried by the parents, e.g., cystic fibrosis (CF), thalassemia, fragile X, spinal muscular atrophy (SMA) [6–7], as well as structural chromosomal aberrations, mainly translocations that can be diagnosed by the use of different technologies [8–10]. Theoretically, PGT can be performed for any genetic condition for which the gene sequence is available, and the familial causative mutation has been identified. However, in the past decade, PGT has been widely performed for chromosomal screening during in vitro fertilization (IVF) cycles to enhance pregnancy and take-home baby rates. In these cases, an aneuploidy screening is performed without any specific parental genetic indication but with the hope that since chromosomal abnormalities represent one of the significant causes of implantation failures and spontaneous abortions, a selective transfer of a euploid embryo will increase implantation rate and reduce spontaneous abortion, due to the enhanced viability of the euploid embryo. Theoretically, an embryo diagnosed as euploid has a higher chance to be implanted, likely reducing the number of attempts until a viable pregnancy and healthy offspring is achieved [11].
The increasing use of chromosomal screening during IVF treatments has recently promoted a modern classification and subdivision of PGT into three major branches: PGT for monogenic disorders (PGT-M), PGT for structural chromosomal rearrangements (PGT-SR), and PGT for chromosomal screening for aneuploidy (PGT-A).
Steps in Preimplantation Genetic Testing
Method of Fertilization for PGT
As PGT is performed using a very limited amount of genetic material, aspirated oocytes should be denuded from the surrounding maternal cumulus cells in order to prevent maternal contamination. The fertilization is regularly performed by ICSI instead of insemination, in order to avoid paternal contamination of sperm cells remaining attached to the zona pellucida (ZP). During the biopsy procedure, it is most important to make sure that perforated ZPs are free of parental cells that might lead to misdiagnosis [12].
Biopsy
Biopsy of preimplantation embryos consists of two main steps: the creation of an opening in the ZP and removal of polar bodies (PBs) or embryonic cells. For PGT, embryos are subjected to biopsy in the IVF laboratory using an inverted microscope and micromanipulator with holding and biopsy pipettes on hydraulic arms. The ZP is perforated using an in-contact laser beam and the genetic origin is aspirated by gently pulling the biopsy pipette [13–15]. When blastomeres are biopsied from a cleavage-stage embryo, it is highly recommended to make sure that the cell contains genetic material, i.e., a nucleus, which is fundamental for DNA analyses. However, nuclei are not always visible due to rapid mitotic divisions of early embryonic stages and blastomeres are characterized by a rapidly dissolving and regenerating nuclear membrane. Several methods have been described for ZP opening and genetic material removal, depending on the stage and morphology of the oocyte/embryo biopsied. Removal of genetic material (PB or blastomere) by aspiration into the biopsy micropipette is the most widely used method and is applicable for all stages of biopsy (mature oocytes and embryos at cleavage or blastocyst stages). Blastomere removal by extrusion or flow displacement can also be applied at any stage of the embryo. While cleavage-stage biopsy was the most widely practiced form of embryo biopsy for over a decade [16], its clinical use has now been reduced compared with the blastocyst-stage biopsy. Nevertheless, the preferred stage for biopsy remains under substantial debate. For blastocyst biopsy, aspiration and excision with a laser can be used as well as an aspiration in combination with the mechanical cutting of the trophectoderm (TE) cells. It is critical to be extremely accurate in matching between the numerical mark of the embryo and numerical mark of a corresponding isolated biopsied cell, in order to prevent mismatch at diagnosis of different embryos. Double-checking by two embryologists is highly recommended at this stage. After a biopsy, the embryo is carefully washed, returned to the culture medium and incubated or cryopreserved until embryo transfer.
Zona Pellucida Opening
A sampling of any genetic material from the embryo (the first and second PBs, blastomere, or TE) is only possible following oocyte/embryo ZP-envelope perforation. Three methods have been developed for this purpose: mechanical, chemical, and laser-aided.
Mechanical opening.
The mechanical method was the first method used to open the ZP and is still applied clinically, although in a very limited number of centers. The method involves creating slits in the ZP, using a sharp micropipette, and scratching the strung ZP by a sharp micropipette onto the holding one. In this way, a V-shaped triangular or square flap opening for biopsy in the ZP is created [17].
Chemical opening.
This type of biopsy is practically no longer in use. It used to utilize acidic Tyrode’s solution (pH = 2.3), which is aspirated into the biopsy pipette. The pipette is lowered to close proximity to the embryo to dissolve the ZP locally. When the breach is opened, the excess of acidic solution in the medium, close to the embryo, is aspirated by the same biopsy pipette. The method was mainly used for cleavage-stage embryo biopsies up to two decades ago and was replaced by other methods due to the potential toxicity of the acid Tyrode’s on embryo viability [18].
Contact laser opening.
In 1990, a laser (ultraviolet [UV] contact) neodymium:yttrium–aluminium–garnet (Nd:YAG) with a potassium titanyl phosphate crystal (the working wavelength to 532 nm) was introduced, and used to drill the ZP of oocytes and embryos [13]. The introduction of the erbium:yttrium–aluminium–garnet (Er:YAG) laser occurred in 1992; this worked at a wavelength of 2900 nm and consequently avoided potential damage to the embryos’ genetic structure [19]. The subsequent years saw the emergence of noncontact lasers working in the infrared or near-infrared range (800–1500 nm), further away from the DNA absorption peak of 260 nm. The first laser with a significant impact on the advancement of ART was the indium–gallium–arsenic–phosphorous (InGaAsP) semiconductor diode laser, operating at 1480–2100 nm (infrared) [14; 20], which is still commonly used today. This laser avoided the mutagenicity and contamination that had previously been related to UV contact lasers, and has become a valuable tool for ART. At present, the laser is the most popular method of ZP opening for PB, cleavage-stage, and blastocyst biopsy. The method involves the use of a guided noncontact laser beam, which can be adjusted to create a ZP opening of the desired size, accurately and rapidly. Exposure to the laser should be minimal and efforts should be made to avoid damage of embryonic cells and impairment of embryo development. In the case of PB or cleavage-stage biopsy, the size of the ZP opening should be adapted to the size of the biopsied material. The hole must be extensive and allow the safe pulling and collection of PB or blastomere, while also avoiding the significant rupture of embryo envelope or remaining cells.
Genetic Material Aspiration
During the opening procedure of the ZP, the position of the embryo is selected such that a blastomere intended for nucleation is at the biopsy-opening side “3 o’clock position.” For sample aspiration, a 30–40 µm-diameter biopsy pipette is used (larger diameters are used for blastomeres and TE and smaller ones for PB biopsy). The biopsy pipette is carried close to the opening in the ZP. The outer edge of the blastomere/PBs and the tip of the aspiration pipette are kept in focus. Using very gentle aspiration, the blastomere/PB is slowly drawn into the pipette and then released into the medium. Extreme care is required to avoid cell membrane rupture and damage to the biopsied cell or the surrounding blastomeres within the embryo. Aspiration of the blastomere with a visible nucleus and proximal to the hole of the ZP is preferred.
The efficient transfer of biopsied cells to reaction tubes, i.e., “tubing,” is a critical step and a determining factor for a successful PGT cycle. In principle, tubing requires careful and accurate handling of embryonic cells to ensure the placement of the genetic material in the tube and to prevent contamination with DNA from exogenous cells.
A sampling of the embryo for genetic testing can be performed using three follow methodologies:
1. Polar body biopsy.
During oocyte maturation and meiosis completion, the oocyte extrudes two PBs one after another. Follicle-stimulating hormone and luteinizing hormone surges promote random segregation of homologs from each of the chromosomal pairs to the first PB and the oocyte, while the sister chromatids of the remaining chromosomes are segregated to the second PB following fertilization [21–22].
Biopsy of first and second PB is performed immediately following fertilization and after the appearance of the pronuclei, respectively, and serves as an alternative to blastomere biopsy. The oocyte is secured by the holding pipette at the “9 o’clock” position, with the PB at the “6 or 12 o’clock” position. The opening of the ZP is made at the “3 o’clock” position and the PB is aspirated with an aspiration/biopsy micropipette. PB biopsy does not adversely affect either fertilization or cleavage. The second PB is removed by introducing the biopsy micropipette through the slit previously made to remove the first PB. This step is delicate, as connections may still exist between the second PB and the oocyte membrane [23–24].
This biopsy strategy was initially suggested in order to determine the genetic status of the embryo by sampling genetic material which is not a part of the developing embryo. Later, due to regulations that prohibited embryo biopsy due to religious or moral laws in some countries (Switzerland, Austria, Germany, and Italy), PB biopsy became common, as it only tests the unfertilized oocytes and fertilized oocytes at the stage before they are considered a zygote.
The advantages of PB biopsy include both minimal impairment of the embryonic cellular array, allowance of two additional days for genetic analysis, and enablement of fresh embryo transfer. However, the use of a meiosis oocyte derivate as a source of genetic material is now less practiced due to the following disadvantages: the need for two sequential biopsies and sometimes, when the first PB is homozygous and resulting data are insufficient, a third biopsy is required. Such repetitive and prolonged exposures of the embryo to unmonitored conditions may be harmful to development. Also, the biopsied genetic material is extremely small and sticky, rendering it challenging to be sampled and collected. The most critical limitation of this technique is the absence of paternal genetic material, thereby rendering it irrelevant for testing for paternal-dominant disorders. For this reason, for recessive mutations, 50% of the embryos, instead of 25%, will have to be discarded. For PGT-A, this biopsy technique also lacks information on postmeiotic chromosomal errors that occur in the first mitotic embryonic divisions. Another disadvantage lies in the fact that the embryo is diagnosed by the elimination of the observed three alleles, diagnosed both in the first and second PBs, out of all four maternal known alleles. This is highly inferior when compared with the direct embryo testing of blastomeres and TE biopsies [25].
2. Blastomere biopsy.
Biopsy of a single cell from a cleavage-stage embryo, usually performed 72 hours after fertilization, is still a technique commonly used for the diagnosis of monogenic disorders. This stage is particularly essential for those of paternal origin and recessive disorders that cannot be diagnosed by PB analysis. For blastomere biopsy, quality cleavage-stage embryos composed of at least six cells and at the pre-compaction stage are exposed to a bivalent cation-deficient medium (Ca2+/Mg2+ free) in order to attenuate tight junctions and disrupt cell adhesions [26]. Following a biopsy, the Ca2+/Mg2+-free medium is rinsed off the embryo with culture medium, at least once, and the embryo is cultured according to standard IVF culture conditions [16; 18]. Embryos with fragmentation exceeding 25% are generally not biopsied and may be cultured to the blastocyst stage for biopsy.
The advantage of this biopsy approach is the direct testing of the embryo (in contrast to “elimination diagnosis” when PBs are tested). The biopsy schedule on day 3 of development allows fresh embryo transfer and, also, in case of amplification failure or inconclusive results, it is still possible to re-biopsy the embryo on day 5 if development to blastocyst stage is accomplished.
The disadvantage of this type of biopsy lies in the limited amount of DNA, resulting in enhanced allele dropout (ADO) rates and amplification failures. For a reliable and accurate analysis, a diagnostic system should be calibrated for single-cell multiplex nested PCR protocols. Besides, blastomere biopsy at this embryo-development stage is not suitable for PGT-A, mainly because of the insufficient amount of DNA required for full chromosomal constitution representation following whole genome amplification. Also, this embryonic stage is characterized by higher rates of mosaicism, compared with the blastocyst stage; as a result, biopsy at this stage may lead to rejection of a euploid embryo due to trisomy or monosomy of the blastomere that represents only the biopsied cell and not the whole embryo [27].
3. Trophectoderm biopsy.
This type of biopsy is the most commonly used for PGT-A cycles and the most suitable means of chromosomal diagnosis using advanced molecular methods such as chromosomal microarray (CMA) and next-generation sequencing (NGS). It is also considered to be safer for the embryos, since biopsied cells are carefully taken from the TE lineage cells, without affecting the inner cell mass (ICM), which is destined for fetal development.
Determination of the fate of embryos based on TE sampling is rooted in the assumption that cells from the blastocyst TE represent the comprehensive chromosomal status of the embryo compared with embryonic cells biopsied from day 3 embryos. Also, due to significantly higher amounts of DNA, TE biopsy is considered to be more reliable and successful than blastomere biopsy for PGT-A [27–30]. Therefore, the estimated rate of inconclusive diagnoses is expected to be below 5%. Moreover, whole-genome amplification (WGA) products of blastocyst biopsies enable running multiple analyses for different indications from the same biopsied cells. Such analyses are essential when both a chromosomal aberration and a monogenic disorder should be diagnosed [31].
For biopsy of TE, one of the following procedures can be performed: slitting a hole in the ZP of good-quality embryos by a laser beam on day 3, and 2 days later, slashing of the tail end of the TE of an expanded blastocyst, which hatched from the perforated ZP, using the laser beam. Alternatively, the ZP opening may be performed early on the day of blastocyst formation, followed by a period of culture to allow herniation of TE cells from the ZP and TE cell removal. The third option is to simultaneously perform ZP opening and TE cell excision on the day of full blastocyst expansion [32]. The biopsy procedure can vary, depending on the morphology and quality of the blastocyst, expansion grade, and the position of the ICM. A biopsy is performed in a HEPES-buffered medium. The Ca2+/Mg2+-free medium should not be used. TE cells are aspirated into the biopsy pipette with gentle suction. Laser pulses are directed at the junctions between cells, to either excise the aspirated cells from the blastocyst or to minimize cell damage while detaching. Biopsied TE cells are mechanically released from the pipette, by making a quick flicking movement of the biopsy pipette against the holding pipette. To avoid cross-contamination during biopsy, it is highly recommended to change the biopsy pipette for each blastocyst. Blastocysts are cryopreserved immediately after the biopsy.
The advantage of blastocyst biopsy is a collection of multiple TE cells for analysis, allowing more reliable results, without invading the ICM (Figure 17.1). However, this type of biopsy suffers from several disadvantages, the most prominent one being the need to freeze the embryos immediately after biopsy before the results of diagnosis are obtained. Also, while feasible, the prolonged culture required for blastocyst formation (day 5, 6, and even 7) has been implicated in lower implantation rates. Although reaching the stage of expanded blastocyst formation serves as a selective parameter and as an excellent positive predictor for pregnancy, prolonged culture also might be harmful to embryos at the genetic and epigenetic levels [33–35]. Another essential drawback is if, for some reason, diagnosis is failed or inconclusive, re-biopsy of thawed embryos is exceptionally complicated. In that case, the embryo should be thaw, re-biopsied and frozen again, and all these procedures impair further embryo survival.
Figure 17.1 Biopsy of a blastocyst.
General Recommendations on Labeling and Witnessing throughout the Biopsy
Double witnessing is recommended during the following stages: (a) immediately after biopsy, to confirm the embryo and sample number match by two embryologists; (b) during spreading or tubing, to confirm that the sample identification matches the labeling on the relevant slide or tube; (c) further embryo culture, when placing the embryo into the labeled culture dish, and (d) in case of cryopreservation, after the biopsy and before acquiring the genetic analysis results, label the cryopreservation device with specific identification of the embryo.
In general, biopsied oocytes and embryos must be cultured or cryopreserved individually, with an identification system to ensure tracking of the biopsy sample and unambiguous post-diagnostic identification.
Principles of PGT Analyses
In general, for PGT analyses, all blastomeres or TE samples biopsied from tested embryos and intended for PGT-M, PGT-SR, or PGT-A are rinsed in a fresh culture medium. The isolated blastomere or TE is then rinsed twice in phosphate-buffered saline supplemented with 10% polyvinylpyrrolidone to prevent the stickiness of the samples and attachment to the aspirating capillary. After the last rinse, the genetic material representing the embryo is gently transferred to the bottom of a PCR tube under sterile conditions. The tubes, with a single embryonic cell in each of them, are immediately incubated at +70°C for 10 minutes to allow DNase inactivation. This stage is pivotal for the integrity of the DNA sample. The samples are then transferred to -80°C, for at least 1 hour. This step is aimed to facilitate the exposure of covered and condensed DNA molecules to the subsequent ingredients and enzymes of the amplification reactions [36].
In the biopsy work area and the first PCR room, protective clothing (preventing genetic contamination of the sample) should be worn, including a full one-time surgical dress, hair cover, face mask covering both the nose and mouth, and shoe covers. Gloves should be worn at all times and changed frequently, whenever coming in contact with a nonsterile object. The tubing (preamplification) area should be in a DNA-free environment. However, due to IVF good laboratory practices, DNA decontamination protocols cannot be applied, and benches, binocular area, and other surfaces are thoroughly cleaned with water and ethanol 70%. Since such DNA decontamination is suboptimal, it is recommended to design a particular area dedicated to embryo biopsy, in proximity to or within the IVF laboratory.
PGT-M
PGT-M refers to the analysis of a possible monogenic mutation in a single cell biopsied from a preimplanted embryo. The tested mutation has an autosomal dominant, autosomal recessive, or X-linked transmission pattern. The justification for conducting IVF with PGT-M (usually for fertile couples) is determined according to the risk for affected offspring, the severity of the disease, the therapeutic options to treat it, the penetration and variable expression, and the time of onset.
In general, PGT-M can diagnose any severe genetic disorder that has already been identified in the family/carrier patient and can also be diagnosed in prenatal samples such as amniocentesis and CVS.
PGT-M, in contrast to PGT-A and PGT-SR, is a tailor-made diagnostic system based on the amplification and characterization of the familial mutation parallel to several polymorphic markers flanking the tested mutation. A qualified molecular laboratory can test as many as several dozen DNA loci in a single biopsied blastomere. This flexibility enables the diagnosis of several genetic conditions simultaneously if needed. For example, to select an immunologically suitable bone marrow donor for an affected sibling, by analyzing the human leukocyte antigen (HLA) typing loci with or without concurrent testing for an additional monogenic disorder [37].
When PGT-M was first introduced, two molecular diagnostic approaches were suggested in order to analyze mutation in a single cell biopsied from a cleavage-stage embryo: WGA and single-cell multiplex nested PCR. In WGA, the DNA of a single cell is randomly amplified (as described later), followed by a second amplification of selected DNA loci, including the mutation of interest and flanking polymorphic markers, custom-designed for each family. For single-cell multiplex nested PCR, only desired DNA loci, including the familial mutation and flanking markers, in contrast to the random amplification in the WGA method, are amplified from DNA originating from the single cell [38]. The second amplification in this method is the nested PCR, in which each of the genetic loci is separately re-amplified, using the same primers of the first reaction or nested primers. The WGA procedure is considered to be less accurate than multiplex PCR and is characterized by higher rates of ADO and total amplification failures. ADO is a phenomenon in which only one of the alleles, maternal or paternal, is amplified, while the other allele is dropped out, likely in the very first PCR cycles. Also, for WGA, a TE biopsy is required, followed by the pre-diagnosed biopsied embryos being frozen separately. The main advantage of WGA is a circumvention of the need for meticulous protocol calibration for single-cell PCR amplification. Nevertheless, most molecular PGT laboratories opt for PGT-M in the single-cell multiplex PCR procedure, which is performed on a single blastomere biopsied from cleavage-stage embryos. Using this highly flexible “home-made” protocol, specific adjustments for each family can be made, enabling the detection of several genetic conditions, all in the DNA from a single blastomere [39–40].
Analysis of a specific DNA locus from a single cell, which contains a single maternal and a single paternal DNA copy, is usually accompanied by amplification failure and relatively high rates of ADO. ADO is a common phenomenon and is the main obstacle in single-cell PCRs and the major cause of misdiagnosis in PGT-M. Such events are especially significant when dominant mutations or recessive disorders, caused by two different mutations, are analyzed. In these cases, ADO of the mutated allele, leading to the demonstration of the normal allele only, may lead to the not desirable transfer of an affected embryo. The main problem with this method is that due to the extremely high sensitivity of the PCR required to amplify a single DNA copy, the yield of the amplicons does not reflect the original amount of DNA in the biopsied blastomere and, therefore, the existence of a single or double normal allele cannot be extrapolated. When ADO of the mutated allele occurs, there is no way to differentiate between normal cells and affected cells carrying the mutated allele that was accidently dropped out [41–42]. When amplifying and analyzing the mutation sequence only, the mutated allele may be dropped out, leading to misdiagnosis since biopsied cells from the affected embryo may be diagnosed as the cells from a normal embryo. In order to prevent such devastating results, monogenic disorders are usually tested by simultaneous amplification of the specific familial mutation, together with several informative polymorphic markers usually represented by short tandem repeats (STRs). The polymorphic markers with STRs are usually long repeats of the C and A nucleotides dispersed throughout the genome. Due to their repetitive nature, cellular DNA polymerase fails to amplify them correctly and deletion or insertion of repeats is frequently observed. Therefore, within generations, variability of these markers increases until it is possible to distinguish both one person from another and also between maternal and paternal alleles in each individual. The diagnosis of a familial mutation by analysis of STR markers flanking the tested mutation decreases the risk for misdiagnosis almost entirely. This immense variability is of value not only for PGT-M but also for other genetic applications, including paternity/maternity determination tests, forensic biology, and others [43–45].
The collection of polymorphic markers closely flanking the mutation of interest (no more than 1.5 million bases upstream and downstream of the mutation) constitutes a stable genomic cluster that, due to the minimal distances between them, is not subject to crossing-over events and is called a “haplotype.” It is technically feasible to differentiate between the maternal and paternal haplotypes and, consequently, between mutated and healthy alleles. Informativity of haplotypes depends on the differences in the numbers of CA repeats between the maternal and paternal alleles and the distances between informative markers and the tested mutation. The chances that a calibrated diagnostic system will drop out an entire haplotype are close to zero. The careful design of each molecular PGT-M diagnostic system for each family ensures a negligible risk for misdiagnosis, alongside high reliability and accuracy.
In cases when the causative mutation is localized on the X chromosome and the disease is expressed in a gender-dependent manner, the gender of the embryo is also determined. For this purpose, the sex chromosomes are analyzed using at least three informative STRs, localized on X and Y chromosomes [39].
Following tailoring the diagnostic system for each family, accuracy and reliability should be validated before the clinical PGT-M cycle. For this purpose, single leukocytes are isolated from the peripheral blood of healthy and affected family members in order to simulate the single-blastomere analysis. Only when all single tested leukocytes are correctly diagnosed, with amplification rates above 95% and an ADO rate for each locus below 10%, is the analytic system considered accurate and reliable. After that, hormonal induction for the IVF treatment cycle for PGT is initiated.
In brief, for the PGT-M, single blastomeres are removed from -80˚C in a clean room dedicated to the first PCR amplification (multiplex PCR for PGT-M or WGA for PGT-A). The most critical steps of a single-gene molecular analysis are the first ones and faults, errors, or contaminations in these initial stages are frequently fatal and irreversible. The first step of PGT-M analysis is the exposure of DNA, which is naturally condensed within the protecting nucleus and covered with histones, to the PCR reaction components. The DNA is accessed by treating the cell with an alkaline lysis buffer before the PCR amplification [12]. For the first multiplex PCR amplification, all primers, designed to amplify the mutation, flanking polymorphic markers, and markers located on sex chromosomes (when an X-linked disease is analyzed), are mixed in the tube containing the single blastomere. The tube also contains other ingredients required for single-cell PCR, e.g., the extremely robust DNA polymerase, special additives that stabilize the DNA, suitable buffer, and other reagents. Meticulous calibration of the PCR program, starting with a prolonged denaturation stage and careful designation and purification of relatively long primers, allows for efficient and accurate amplification of many DNA loci from an extremely limited amount of DNA [39]. At the end of the first PCR amplification, the PCR tube content containing a significant but still insufficient amount of DNA of each of the desired loci is subjected to a second PCR reaction, in which each locus is further amplified [46]. To this end, aliquots of each analyzed blastomere (representing each of the analyzed embryos) are prepared. In each tube, each locus amplified in the first PCR reaction is amplified separately. At the end of the second PCR, the amount of DNA is highly amplified and subjected to fragment analysis for haplotype determination and mutation testing, or incubated with specific restriction enzymes which discriminate between normal and mutated alleles. Collecting, editing, and analyzing all data of direct mutation testing, together with haplotype results for each sample, enable accurate genotyping of each of the biopsied embryos. The final results are then shared with the IVF laboratory; after which, the healthy and transferrable embryos are selected.
PGT-SR
PGT-SR refers to the analysis of an unbalanced chromosomal constitution arising from a pathogenic aberration already identified in the family of the patient. Most PGT-SR tests analyze the chromosomal constitution of embryos derived from a couple in which one of them is a carrier of a balanced translocation. Due to meiotic chromosomal segregations, those couples have about 60% risk for unbalanced offspring. In individuals with an unbalanced chromosomal constitution, partial chromosomal deletion and/or addition is observed, and viability and severity of the syndrome will depend on the numbers and functions of genes localized on DNA regions involved in the aberration.
At the very beginning, only fluorescence in situ hybridization (FISH) was available. However, in the past decade, advanced molecular methods, such as array-based comparative genomic hybridization (aCGH), single nucleotide polymorphism (SNP) array, and NGS were introduced and serve as the gold standard for PGT-SR as well as for PGT-A [47]. Although these advanced technologies enable detection of all expected unbalanced forms of the chromosomal rearrangement, they do not distinguish between embryos with a normal karyotype and embryos with an abnormal but balanced aberration.
Fluorescence In Situ Hybridization (FISH)
When PGT-SR was first introduced, FISH was the standard diagnostic method applied to detect numerical and structural chromosomal aberrations. FISH-based PGT was mainly applied for detection of inherited chromosomal rearrangements but also for embryo sexing, and for the analysis of X-linked diseases, such as Duchenne muscular dystrophy. FISH enables the labeling of specific chromosomal regions that are involved in structural rearrangements or indicative of sex chromosomes. By using specific DNA fragments designed to hybridize with known chromosomal regions and labeled with fluorescent tags in different colors, the number of chromosomal signals can be counted. In the analysis of autosomal chromosomes, two signals represent a balanced embryo and other numerical combinations hint to an unbalanced chromosomal constitution. Fluorochrome probes are specifically designed to hybridize the centromere region, the telomere region, or the entire chromosome (chromosome painting). The specific probes and fluorescent tags are carefully selected according to the chromosomal aberration in question and are usually ordered from a commercial supplier.
For the FISH analysis, two blastomeres are usually aspirated from a cleavage-stage embryo and carefully lysed with a spreading buffer placed over a positively charged glass slide [48], and left until the nucleus is separated from other cell components and is clearly observed. The area on the slide where each nucleus is localized should be immediately bordered with a diamond pencil (mark the opposite side of the slide) and the serial number of the biopsied embryo should be indicated [48]. The slides embedded with embryonic interphase nuclei are treated with proteinase, to remove cytoplasmic remnants and to achieve better exposure of DNA and enhance its accessibility to the probes. Nuclear and probe DNA are simultaneously denatured and generally incubated with three probes that can differentiate between the balanced and unbalanced chromosomal constitution of the tested aberration. After overnight incubation, excess probes are rinsed and signals are observed using a fluorescence microscope [49–51].
To analyze reciprocal translocations, a combination of three informative probes with three different fluorescent colors (one color per probe), targeting both chromosomes involved in the translocation, are used. In order to detect all unbalanced segregation products of a reciprocal translocation and to reliably discriminate between normal/balanced and unbalanced chromosomal constitution, the three probes should be designed in correlation to the translocation breakpoints, with either two distal and one proximal, or two proximal and one distal. For Robertsonian translocations and inversions, two fluorescent probes are sufficient. For deletions and duplications, locus-specific probes for the deleted or duplicated region should be used, and a control probe (usually on the same chromosome) should be included.
In contrast to conventional cytogenetic analysis, FISH does not require cells at the metaphase stage and can analyze interphase nuclei as well. Compared with other advanced molecular methods, FISH is relatively cheap, rapid, and simple, and does not require dedicated or costly equipment. Also, the biopsy for FISH can be performed on day 3, so diagnosed unaffected embryos can be transferred in fresh cycles. However, this early stage of embryo development is characterized by high mosaic rates. Biopsy of a blastocyst for a FISH-based diagnosis is not recommended because both separation of different nuclei from the same biopsied TE cells and avoidance of overlapping of the nuclei and their signals are difficult. While biopsy of more cells usually increases reliability in this case, it increases the uncertainty of results suffering anyhow from relatively low accuracy and reliability, compared with PCR and CMA/NGS techniques.
Drawbacks of FISH analysis include the delicateness required to spread blastomeres on a slide, requiring high proficiency, and often met with obstacles. Also, the diagnosis is based on a visual and subjective inspection of fluorescent signals, which are often misinterpreted due to overlapping signals, where two different signals may be considered as one and two chromosomes are interpreted as only one. Similarly, when the signal is split, one signal may be considered as two different signals and one chromosome is interpreted as two. These two types of misinterpretations account for the relatively high rates of misdiagnosis. Another significant disadvantage is that, in comparison to other molecular cytogenetic methods, which provide comprehensive details of the chromosomal constitution, FISH results are limited to the loci targeted by the specifically selected probes. The FISH analysis is also limited by the small variety of available fluorescently colored (wavelength) probes. A maximum of three to nine chromosomes from each embryo biopsy specimen (when two separated hybridizations are performed with striping of probes between the first set of DNA probes and the second set) can be analyzed in a single cell. Therefore, FISH-based PGT is currently only used for chromosomal rearrangements involving small fragments or subtelomeric regions of chromosomes that are difficult or impossible to detect using other advanced molecular diagnostic methods, e.g., NGS and DNA array. For sex determination, the use of multiplex single-cell PCR of specific CA repeats localized on X and Y chromosomes is much more accurate, reliable, and cost-effective than FISH analysis, with only one probe for each of the sex chromosomes [32].
Chromosomal Microarray (CMA) and Next-Generation Sequencing (NGS)
Over the last decade, molecular genome-wide technologies, such as aCGH and NGS, have proven to provide a comprehensive means of chromosomal screening, and have gradually replaced the FISH method. In general, for PGT-SR (and for PGT-A as well), advanced molecular methods and significantly higher amounts of DNA are needed to perform both aCGH and NGS. Therefore, TE biopsy should be performed at day 5 or 6 and then subjected to WGA. During this initial and most critical stage of the analysis, the embryonic DNA is randomly and uniformly amplified using random primers. The WGA product can be further analyzed by CMA or NGS techniques, to obtain the complete constitution of all 23 chromosome pairs.
Whole-Genome Amplification (WGA)
When DNA quantity in a tested sample is limited and not sufficient to allow diagnosis by molecular and cytogenetic analysis, WGA can be used to amplify a DNA template of interest. This approach is used when preimplantation embryos are biopsied and comprehensive chromosomal analysis is required. WGA can amplify the original amount of DNA from a small number of single TE cells, enabling further analyses. Technically, it is possible to use the same method for single blastomere amplification. However, in a single cell, the amplified product is often of poor quality, with significant variances in the representation of different genomic regions. While several WGA protocols, using different types of primers or different DNA polymerization enzymes, are available, they are all based on the same principle of random amplification, using complete or partially random primers. Three basic strategies were developed and are still commonly used for WGA. In the primer extension preamplification (PEP) method, the primers utilized for the amplification are entirely random and complementarity between primers and the DNA template is very low. To achieve amplification under these conditions, a relatively low temperature is used during PCR cycles to allow the random primers to anneal to the DNA template. In the degenerate oligonucleotide polymerization (DOP) protocol, only the six nucleotides in the center of each primer are random, while the rest of the nucleotides are constant in all used primers. This structure allows the use of a higher annealing temperature, which increases productivity and uniformity compared with the PEP method. DOP-PCR is the method of choice for the detection of copy number variations (CNV). The third and commonly used method is the multiple displacement amplification (MDA) method. This approach is based on the annealing of random short primers (hexamers) to the DNA template, followed by strand displacement polymerization, which results in many amplification branches on the same strand. This amplification is performed under isothermal conditions instead of the regular PCR program, which is based on repetitive cycles of temperature changes for DNA denaturation, annealing, and elongation. The branching and continuity of amplified amplicons yield PCR products that are much longer than following PEP or DOP amplification. This unique amplification method is enabled by a special DNA polymerase – Phi29, which exhibits very high enzymatic activity compared with the commonly used Taq polymerase. The amplicons in MDA can reach to thousands of base pairs in length, while the average fragment sizes achieved in PEP and DOP are 400–500 bp. It has been claimed that this method of amplification increases fidelity, and prevents slippage, stoppage, and dissociation of the polymerase from the template, resulting in higher uniform amplification of the genome. Commercial kits, based on the PEP, DOP, or MDA technologies or a combination of these methods, are available and frequently compared. Some differences and advantages of the range of commercial products have been described, mainly concerning fidelity, as tested by exome sequencing. Before choosing the method of preference, all the WGA methods should be evaluated with regards to uniformity of genomic coverage, high fidelity of the sequence, reliability of CNV quantification, and technical ADO-rooted errors. For CMA, it seems that the differences between strategies and commercial kits are insignificant [52–53]. A suboptimal WGA product, with regards to the relative and absolute DNA amounts and most importantly, genomic amplification uniformity, will negatively influence test reliability and accuracy. Poor WGA quality may lead to total amplification failure and even to misdiagnosis.
For the WGA procedure, the biopsied TE sample is handled as in PGT-M and is performed precisely according to the manufacturer’s protocol. Usually, the WGA protocol includes cell lysis, preamplification preparations, such as fragmentation or ligation, and final amplification. In some of the commercial kits, the quantity and quality of DNA amplification can be estimated by agarose gel separation, before advanced genetic analyses.
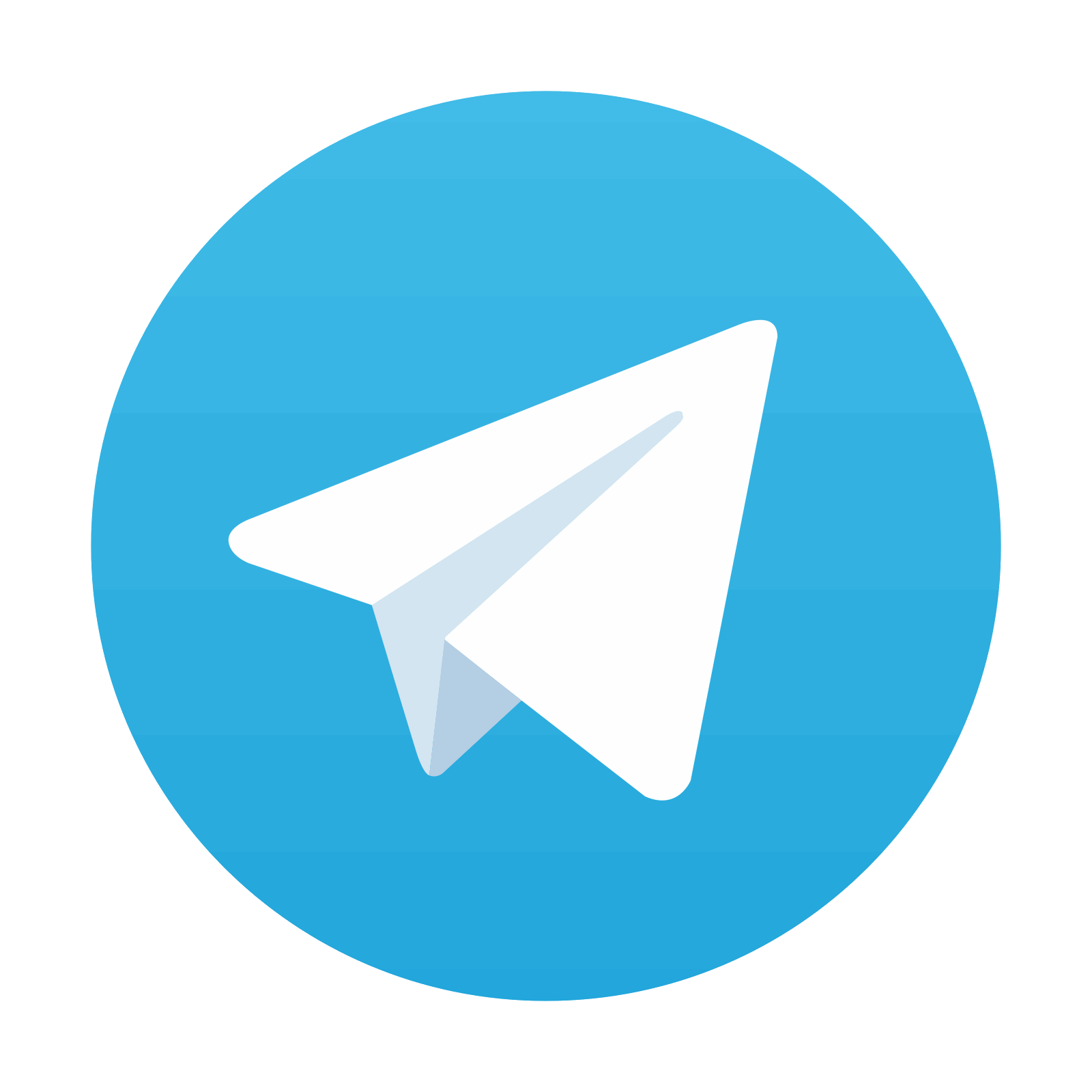
Stay updated, free articles. Join our Telegram channel

Full access? Get Clinical Tree
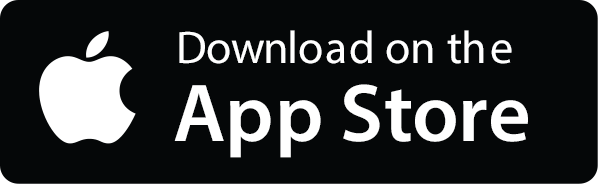
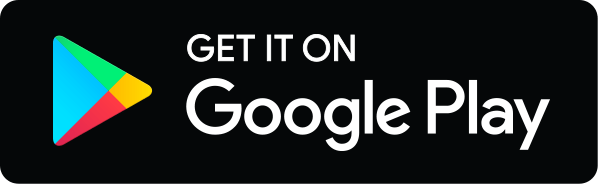