Abstract
For persons who wish to have children but are unable to produce their gametes, assisted reproductive technology (ART) involving donated gametes suggests a means of becoming gestational and social parents (Figure 15.1). Conceiving with a donor gamete ultimately yields a child who lacks genetic relations with one or both of the parents. In order to compensate for this genetic lack, some fertility clinics match the ethnicity of gamete donors and recipient parents, to increase the likelihood that the resulting child will have phenotypic characteristics of the receiving parent despite the absence of a direct genetic link. This matching allows the family to keep secrecy about the use of a donor by ensuring that the child could look as a genetic child.
For persons who wish to have children but are unable to produce their gametes, assisted reproductive technology (ART) involving donated gametes suggests a means of becoming gestational and social parents (Figure 15.1). Conceiving with a donor gamete ultimately yields a child who lacks genetic relations with one or both of the parents. In order to compensate for this genetic lack, some fertility clinics match the ethnicity of gamete donors and recipient parents, to increase the likelihood that the resulting child will have phenotypic characteristics of the receiving parent despite the absence of a direct genetic link. This matching allows the family to keep secrecy about the use of a donor by ensuring that the child could look as a genetic child. Nonetheless, given that such “ethnic” matching also involves the classification and matching of people based on characteristics that are typically associated with “race,” such as skin color and hair color, it raises ethical concerns regarding racism [1].
Figure 15.1 Percentage of ART general cycles resulting in live birth and miscarriage by age of women.
The ready availability of sperm donors and the ease of artificial insemination have long enabled couples to treat male infertility. The development of in vitro fertilization (IVF) and related techniques has made oocyte and embryo donation another option for infertile couples. Gamete donation is an ethically and legally accepted procedure in most countries. Donation is also associated with the highest pregnancy and live birth rates after IVF (Figures 15.2 and 15.3) [2].
Figure 15.3 Egg donation impact on IVF success rates.
The family and medical history of the donor is established to exclude the presence of major Mendelian disorders, chromosome rearrangements, and multifactorial disorders that have a significant genetic component [3]. In most assisted reproduction clinics, donors of sperm, eggs, or embryos undergo some genetic screening procedures in order to maximize the health of the donor-conceived offspring. Genetic tests may include karyotyping and genetic screening for the carrier status of specific conditions such as cystic fibrosis, spinal muscular atrophy, thalassemia, Tay–Sachs disease, and fragile X syndrome. Recent advances in genetic testing technologies now enable more extensive genetic testing of donors than ever before. To date, most attention is directed towards expanded carrier screening for autosomal recessive disorders [4]. New genetic testing technologies could potentially be used to screen for undiagnosed autosomal dominant disorders and even for susceptibility to some multifactorial diseases [5]. Donors and recipients alike acknowledge the importance of genetic information. Recipients are concerned that expanded genetic screening of donors might lead to additional financial costs, but still recognize the potential benefits of performing more extensive genetic screening of donors [6]. Both recipients and donors are apprehensive about extended genomic technologies, with concerns relating to how this information can be used and the ethics of genetic selectivity.
Usually, female and male donors differ in their social status. Over half of the female donors have children of their own when they make a donation. In contrast, male donors are more likely to be single at the time of donation.
The communication and interaction between gamete donors and fertility clinic staff remain to be improved, as donors often feel disrespected and treated as a commodity by clinic staff [7].
Sperm Donation
Sperm donation has been used in the treatment of male infertility for more than 100 years. It is known that pregnancy rates using frozen semen are lower than those with fresh semen [8]. Due to the risk of acquired viral infections, as hepatitis (HBV, HCV), human immunodeficiency (HIV), or cytomegalovirus, frozen sperm for clinical use are quarantined for 6 months until the donor has been retested and found to be seronegative.
Sperm donors.
Complete medical, genetic, social, sexual, and family histories must be taken at the time of the initial interview and donors must undergo a physical examination. Usually, men younger than 40 years old are eligible donors. The drawback of using sperm from older men is the increased risk of neurological and chromosomal abnormalities in offspring (autism, schizophrenia, achondroplasia). Before cryopreservation and post-thaw cryo-sensitivity testing, two semen analyses must be performed using criteria established by the semen bank.
The fertility and health of the donor must be immutable, and there should not be a family history of genetic disease. Screening for thalassemia in Mediterranean races, Tay–Sachs heterozygosity in donors of Jewish origin, and sickle cell disease in donors of African origin should be executed. Individuals with a history of herpes, chronic hepatitis, or venereal warts should be excluded. There is no simple method of thoroughly ensuring that infectious agents will not be transmitted by donor sperm, but proper screening should make this a remote possibility. Tests should be performed when the donor is first seen and at repeat donations.
Genetic screening is recommended for all potential donors. Moreover, pairs need to be aware of the fact that in 4–5% of pregnancies using donated sperm, the child could be born with a congenital anomaly. These anomaly rates are comparable to those seen with spontaneous pregnancies [9].
In a sense, most women want the donor to resemble their partner as much as possible. The resemblance is generally limited to hair color and eye color, ethnic origin, height, and blood type.
Quite a debate continues on the type of gamete donors to be recruited and payment of fees or expenses. Traditionally, sperm donors have received a nominal fee, including reimbursement for their direct and indirect expenses. Most sperm donors are young single students and soldiers who are motivated predominantly by financial incentives. It has been recommended that donors should be paid discomfort fees similar to payment made to healthy volunteers who take part in drug and treatment trials [10].
Positive communication and interaction between donors and fertility clinic staff are of critical importance. Donors conveyed frustration after not receiving information on the expenses they could claim. Donors also negatively commented on aftercare, location and condition of the donation room [11], including the location of the waiting room, and visibility of the donation room to fertility patients, and clinic staff. Hygiene of the donation room is also critical.
Limiting donors.
There is concern regarding the risk of exposure among the offspring of the same donor. This is a problem in small towns in which a limited supply of donors is available. It has been recommended that in a population of 800 000, limiting a single donor with no more than 25 pregnancies will avoid inadvertent consanguineous conception [12]. However, only 20–30% of the pregnancies produced through commercial donations are ever reported to the sperm banks [13].
Record management.
The nearly universal practice is to maintain the confidentiality of both donors and recipients. In the United Kingdom, the Human Fertilisation and Embryology Authority (HFEA) policy dictates that those who donated sperm, eggs, or embryos after April 1, 2005 are, by law, identifiable. Donor-conceived individuals conceived after April 1, 2005 can apply to the HFEA after the age of 16 years, to receive non-identifying donor information; after reaching the age of 18 years, they can apply to the HFEA to find the information their donor provided, including identifying information.
Records, including those about donor suitability, quality assurance, sample collection, processing, storage, and medical and laboratory data, must be booked for at least 10 years after insemination [14]. This allows donors and recipients to be tracked in the event of a medical problem in the donor or donor’s family.
Oocyte Donation
Oocyte donation was first described in the early 1980s. It was thought to be a logical method of achieving pregnancy in women with premature ovarian failure as well as in those who wished to avoid passing on to their children a heritable disease. Since cryopreservation was in its infancy, when a more significant number of oocytes were obtained, one or more could reasonably be donated to a potential recipient [15]. The technique used induced no prohibitive immune reaction. Also, it prepared the recipient’s uterus for implantation using exogenous estradiol and progesterone (P4). Donation appeared to be a viable option for women who needed a donated egg to conceive. Early oocyte donation methods relied on natural ovulation and fertilization in the donor. The developing embryo was then flushed from the uterus of the donor before implantation and then transferred to the uterus of the recipient [16], whose ovulation was monitored and thus known to be synchronous with the ovulation of the donor.
Transvaginal ultrasound-guided follicular aspiration revolutionized oocyte donation. Egg donors no longer needed to undergo insemination with sperm or risk a subsequent retained pregnancy. Controlled ovarian stimulation and standard IVF procedure could be used, thus increasing the yield and efficiency of the process. Since oocyte quality could now be controlled, the effect of the age of the uterus on pregnancy success could be estimated. No decrease in pregnancy rates was observed with increasing recipient age, even beyond the age of 40 years [17]. Obstetrical risks are higher for older women, but pregnancy rates with eggs donated by young women remain high, regardless of the age of the recipient.
In oocyte donation the procedure requires the donor to undergo superovulation, as with conventional IVF. Oocyte retrieval is performed and the donor oocytes are fertilized in vitro with the sperm of the partner of the recipient. The fertilized oocyte (embryo) is transferred to the hormonally synchronized recipient or cryopreserved for transfer at a later date. Although the number of potential recipients is increasing continuously, the shortage of donors is one of the significant difficulties in establishing a donor oocyte program. Anonymous donors were recommended to be under 35 years of age. The disadvantage of using oocytes from older women is the increased risk of chromosomal abnormalities. Another possible problem is that older donors produce fewer oocytes than younger women.
In the early 2000s, techniques of oocyte cryopreservation advanced to the point where reliable results could be obtained with elective oocyte cryopreservation and subsequent thawing. In the context of oocyte donation, oocyte cryopreservation made possible the establishment of oocyte banks, which provided frozen donor oocytes to recipients in a manner analogous to that of frozen sperm in sperm banks.
Ovum sharing raises several ethical and medical concerns. A “shared” ovum donation program is limited by the number of ova available for donation and oocyte quality. Ethical problems might arise in cases where the recipient woman conceives and gives birth to a child while the donor herself does not conceive.
Attempts to reverse the age-related drop in oocyte quality through micro-manipulation of the nucleus and cytoplasm have produced disappointing results and ethical concerns have been expressed. In contrast, the use of oocytes from younger donors is associated with very high rates of conception in menopausal women. The increased obstetrical risks in this population, which bear a higher rate of underlying medical comorbidities, must be considered prior to attempt assisted conception, often in consultation with a multidisciplinary team of physicians.
Oocyte donors.
Oocyte donors should have attained their legal majority and preferably be between the ages of 21 and 34 years. Proven fertility is desirable but not required. All prospective oocyte donors should be screened for normal karyotype, and genetic and infectious diseases in order to minimize transmission to the recipient or her offspring. The antral follicular count should be more than 10. The history and physical evaluation should rule out inherited disorders, and the possibility of reproductive dysfunction.
A number of controlled ovarian hyperstimulation protocols have been developed for donors, including the administration of gonadotropins in conjunction with gonadotropin-releasing hormone agonists. When follicles, according to size and number, are ready for aspiration, ultrasound-guided transvaginal oocyte recovery is performed. After a preincubation interval of several hours, the donor oocyte is fertilized with the recipient’s partner’s sperm.
A side effect of the fertility drugs, used to stimulate the ovaries to release oocytes, is an ovarian hyperstimulation syndrome (OHSS) with symptoms including abdominal swelling, nausea, dehydration, and breathing difficulty. Oocyte donors reported to have OHSS often sensed that clinic staff were not concerned about their physical or emotional well-being but instead focused disproportionately on extracting the oocytes [11].
Recipients.
Initially, the primary indication for oocyte donation was a premature ovarian failure, defined as hypergonadotropic hypogonadism occurring before the age of 40 years. Later, oocyte donation became widespread throughout the world to treat a variety of reproductive disorders, some of which are age-related.
Both the oocyte recipient and her partner should be healthy, and there should be no physical contraindication to pregnancy. Screening for infectious diseases in the patient and her partner generally includes tests for HIV, hepatitis, chlamydia and syphilis. Hysterosalpingography should be performed to evaluate any uterine abnormalities.
Many young women are electing to delay childbirth for personal, economic, or professional reasons. However, fertility potential decreases with advancing maternal age and it is expected that older women between the ages of 35 and 45 will be unsuccessful in their effort to reproduce. The introduction of oocyte donation to establish pregnancy in patients with age-related infertility has allowed many older women a new opportunity to conceive. Maximal age of recipients for egg donation in most European countries is 45 years, in England is 50 years, in Israel is 55 years, and in the USA, it is only suggested before the age of 50 years.
Though implantation and clinical pregnancy rates are similar between vitrified donor oocytes and fresh donor oocytes [18], pregnancy presents a high medical risk for the elderly mother and her fetus [19]. It has been shown that most of the complications in pregnancy associated with older age are caused by age-related confounders such as diabetes mellitus, hypertension, and multiparity [20]. Postmenopausal women are considered at a particularly increased risk of vascular complications during pregnancy. There are increased rates of obstetrical and maternal complications with increasing maternal age, including maternal death, fetal and neonatal death, preterm delivery, and low birth weight and efficient delivery [21–25]. However, these risks are already increased in women over the age of 40; nonetheless, treatment is offered to women between the ages of 40 and 55 without significant debate.
Embryo Donation
Embryo donation is a new procedure and is a well-established and successful form of ART when both partners are infertile. The vast majority of embryo donors are couples who completed IVF procedures and who had the extra embryos cryopreserved. The embryo donors must sign an informed consent document stating their consent to donate their embryos, renouncing all rights to the embryo and any child that may result from the transfer of these embryos. They should be screened for genetic and infectious diseases to prevent transmission to the recipient or the offspring.
Recipient couples should be made aware of the limitations of genetic and infectious disease screening and informed that there is no guarantee that the resulting child will be born free of birth defects or illnesses. Couples who receive embryo donation should be thoroughly evaluated, including medical history, physical examination, and psychological counseling. A pelvic ultrasound evaluation should be performed to assess uterine size and endometrial thickness and to rule out pelvic pathologies such as endometrial polyps, myomas, or ovarian cysts.
Ooplasma Donation (Mitochondria Donation)
At the end of the 1990s, an alternative to oocyte donation termed “cytoplasmic donation” was developed [26–27]. In 1997, Jacques Cohen and coworkers reported on the first human pregnancy following the transfer of cytoplasm from donor oocytes to oocytes of a patient with a history of poor embryo development [26]. In this technique, a fraction of ooplasm from a donor oocyte is co-injected into a recipient oocyte with sperm during intracytoplasmic sperm injection (ICSI). This ooplasm contains mitochondria and other organelles, proteins, and mRNAs. The presence of extraneous cytoplasm appears to increase the quality of the recipient oocyte to the level where the embryo produced is viable. To note, significant improvement in embryo quality does not always occur after cytoplasmic transfer. The donation of ooplasm appeared to result in a reduction in the level of embryo fragmentation and an increase in the number of blastomeres present in the embryo [28]. The mixed (heterologous) ooplasmic transfer has also been reported to lead to successful pregnancies [29]. Donor mitochondrial DNA was identified in the offspring as “three genetic parents.” The sufficient benefit of this technology was never fully proved; its safety has been questioned by many specialists and it has raised both ethical and genetic questions. The US Food and Drug Administration banned the procedure in 2001 until a clinical study could be conducted to determine its safety. Although no such study was performed, this technology is still commercially available in IVF clinics in numerous countries worldwide.
Mitochondria are a key component of ooplasm. Mitochondria constitute the powerhouse of cells, as they synthesize ATP by oxidative phosphorylation. They possess their own small genome in the form of mitochondrial DNA (mtDNA). Mitochondrial replication starts in the primordial germ cells (PGCs) and continues during early oogenesis; however, a sharp increase in mitochondria counts is observed during later stages of oogenesis [30]. Thus, whereas PGCs only contain a few hundred copies of mtDNA, mature oocytes contain up to several hundred thousand copies [31]. Since mitochondria in oocytes are ancestral to all somatic mitochondria of the next generation and to all cells of future generations, oocytes must prepare for the high energetic demands of maturation, fertilization, and embryogenesis. Unlike the nuclear genome, which is derived from both the egg and sperm at fertilization, the mtDNA in the embryo is derived almost exclusively from the egg, i.e., it is of maternal origin. Mutations in mtDNA occur at a ≥10-fold rate than in nuclear DNA, possibly due to the high concentration of free oxygen radicals, lack of histones, and limited mtDNA repair mechanisms in the mitochondria [32]. Pathogenic maternally inherited mtDNA mutations are a frequent cause of severe human disease and are found in 0.5% of the population [33], with disease affecting at least 1 in 5000 individuals [34]. Interest in mtDNA mutations has grown due to the increasing number of associated diseases and because they can affect patients throughout life. Also, mtDNA mutations are increasingly implicated in high-energy requirement body systems such as the brain, skeletal muscle, heart, and liver, and are associated with Alzheimer’s, Parkinson’s, Huntington’s diseases and Leigh syndrome [35–37]. ART could eliminate the transmission of mitochondrial diseases in affected families by performing cytoplasmic, germinal vesicle (GV), and pronuclear transfer.
Typically a cell contains only one type of mtDNA (homoplasmy). Mixtures of mtDNA types (heteroplasmy) allow lethal mutations to persist and, most importantly, to pass to the next generation. Many mtDNA diseases are heteroplasmic. Currently, there are no therapies for mitochondrial disorders and available treatments only alleviate symptoms and slow disease progression. Therefore, there is a significant necessity to consider new therapeutic approaches that could prevent the transmission of mtDNA mutations from mother to child.
Another possibility of treatment is transferring nuclear DNA from a mother with mtDNA disease to a cytoplast (or spindle-free oocyte) containing normal mtDNA obtained from a healthy egg donor. The procedure comprises removal of nuclear DNA from an unfertilized oocyte of a patient carrying abnormal mitochondria, followed by its transfer to an enucleated donor oocyte containing assumed healthy mitochondria. ICSI subsequently fertilizes this constructed oocyte. However, such mitochondrial manipulations have come under criticism worldwide [38] as these techniques raise the risks of heteroplasmy, linked to the use of mitochondria or ooplasm from a donor. A pronuclear transfer is essentially the same procedure, except that the nuclear material, both the male and female pronuclei (pN), is removed after fertilization. Spindle–chromosomal apparatus transfer is free of mitochondria [39].
Injection of purified mitochondria instead of ooplasm presents a potential alternative means of delivering more massive amounts of mitochondria into the oocyte [40]. For such procedures, the mitochondria should be obtained from the patient’s cells, which, optimally, should be of ovarian or oocyte origin [41–42], and the mtDNA from these cells should be of high quality, without deletions or mutations [43].
Transfer of mitochondria from putative oogonial stem cells (OSCs) into oocytes of low responders [44] led to limited improvement in oocyte functions. In the context of ICSI, the mitochondria were injected into oocytes to augment oocyte performance; healthy live births or ongoing pregnancies have been reported [45]. It was established that the oocyte mtDNA copy numbers per cell and accumulation of mtDNA mutations were not found to be different between older patients with a low ovarian reserve and younger patients [46], so a number of mitochondria per cell should not be a point reason in the reduction of functionality. Furthermore, if OSCs are homologous with PGCs or oogonia, they are expected to have only a few thousand copies of mtDNA compared with the 160 000 copies found in mature oocytes. It remains unclear how mitochondria transfer restores oocyte competence if the aneuploidy is preexisting at metaphase II (MII) in oocytes from aging patients. It is unlikely that mitochondria transfer can boost the embryonic developmental potential of low-quality oocytes obtained from subfertile patients.
So, which of the existing genome replacement approaches available offers the best means of completely eliminating affected mtDNA? While the three options (GV, MII, and pN) are technically similar, they differ significantly from a cell cycle point of view. Mitochondria undergo redistribution along with meiotic progression, a process that has been documented in human oocytes [47]. In GV-stage oocytes, mitochondria are concentrated in the perinuclear space, often associated with lipid vesicles [48]. Thus, there is tremendous potential for transferring a significant amount of patient mtDNA into the donor oocyte following GV transplantation. During meiotic progression, mitochondria scatter in the cytoplasm, where they tend to concentrate in the inner cytoplasm. At the pN stage, mitochondria are again concentrated in a perinuclear position, particularly during pronuclear opposition [49]. Hence, it is likely that there will be less carryover of patient mtDNA in MII karyoplasts compared with pN transfer. The proportion of mtDNA copies carried over has been estimated to be 1% in MII [50], versus 8% [51] in pN transfer, which aligns with the mitochondria distribution dynamics.
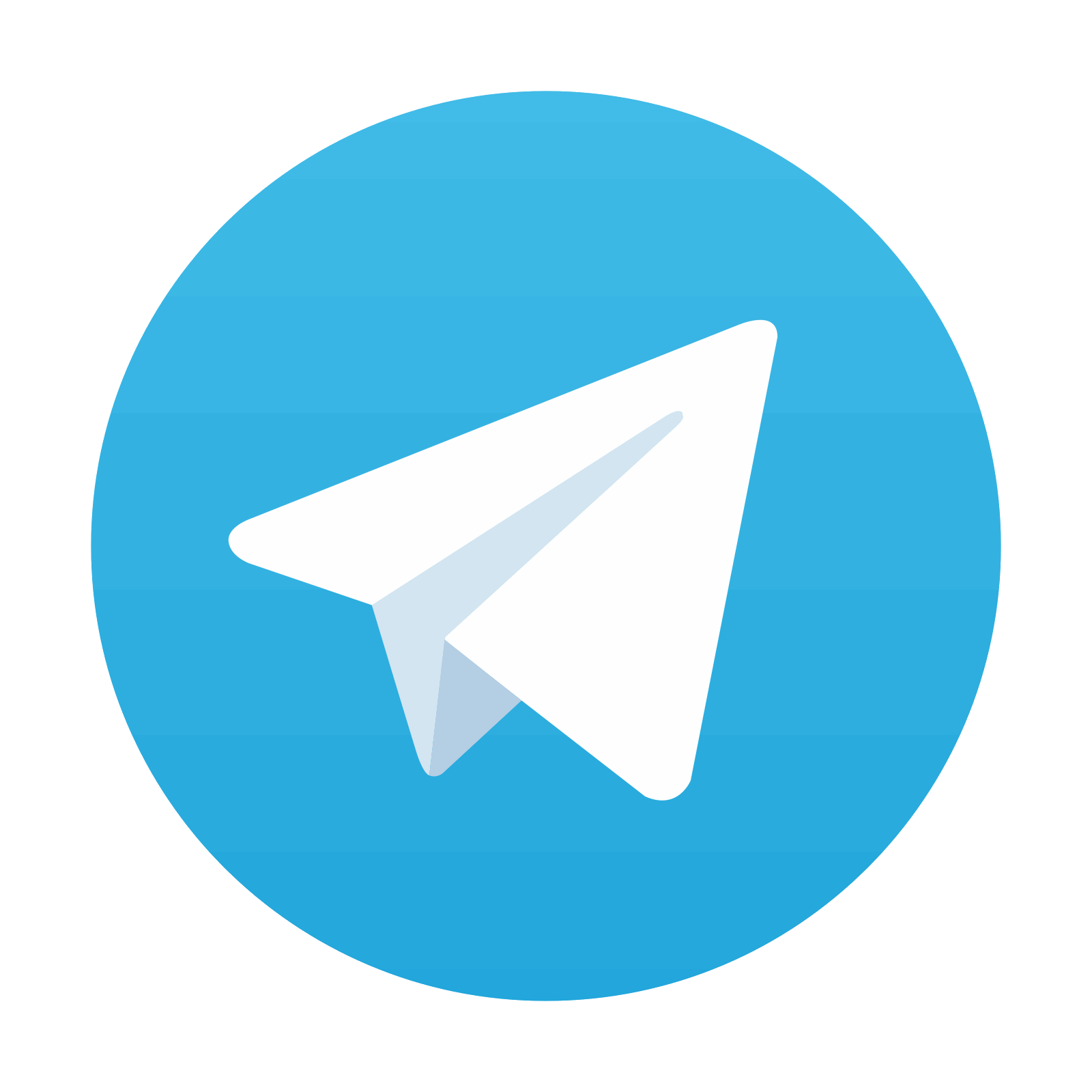
Stay updated, free articles. Join our Telegram channel

Full access? Get Clinical Tree
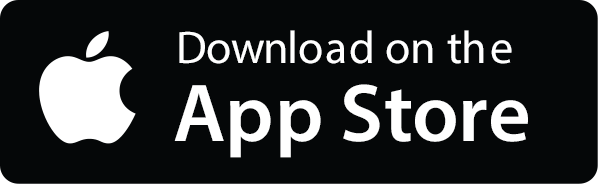
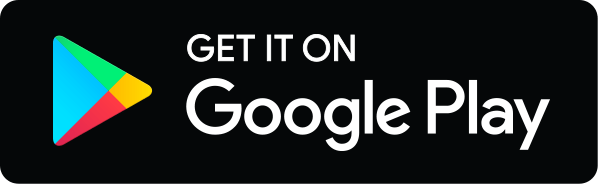