Bone Marrow Transplantation
Allen R. Chen
Jeff C. Hoehner
Oncology Center, Johns Hopkins Hospital, Baltimore, Maryland 21287.
Division of Pediatric Surgery, Department of Surgery, Johns Hopkins University School of Medicine, Johns Hopkins Hospital, Baltimore, Maryland 21287.
Bone marrow is the most frequently transplanted organ, and bone marrow transplantation (BMT) differs from solid organ transplantation in many important respects (Table 50-1). In contrast to solid organ transplantation, in BMT, immunologic reactions occur not only in the direction of rejection (host versus graft), but also in the opposite, graft versus host, direction. Graft versus host disease (GVHD) occurs after BMT because the immune system is composed of marrow-derived cells, and the marrow graft contains an enormous number of functional immune cells. In fact, after BMT, GVHD is a much more significant clinical problem than graft rejection. Nevertheless, after successful BMT, full immunologic tolerance generally ensues such that all immune suppression can generally be withdrawn several months after transplantation, and full donor marrow engraftment persists, without GVHD.
In addition, whereas the usual indication for solid organ transplantation is failure of the organ to be transplanted, the indications for BMT are diverse. BMT is performed far more frequently for poor-prognosis malignancies than for marrow failure syndromes.
The marrow graft itself is also unusual in that it consists of stem cells and progenitor cells of such high proliferative capacity that the donor suffers no loss of organ function as a result of marrow donation. Therefore, cadaveric marrow is not used, and registries have been established internationally that have facilitated thousands of BMTs from a pool of millions of unrelated volunteer donors. Moreover, the graft can readily be cryopreserved. Therefore, autologous marrow or peripheral blood stem cells (PBSCs) can be stored for subsequent transplantation. This is a useful strategy for treating certain poor-prognosis malignancies.
IMMUNOLOGY
If the preparative regimen does not fully destroy the patient’s immune system, immunologic rejection of the graft may ensue. If the graft contains functional immune cells, there is the potential for the graft to reject the patient. What determines whether these reactions occur?
By definition, an alloantigen is any molecular structure that, when transplanted between different individuals of the same species, can elicit an immune response. Normally, the immune system is tolerant to self, and in principle, any polymorphic molecule may be recognized as nonself, and thus may serve as an alloantigen. Which alloantigens are most potent? The importance of the human leukocyte antigens (HLAs) for BMT outcomes has been recognized for decades, and is now understandable at the level of molecular and cellular physiology. The function of the HLA system is to present antigen to immune cells. Antigen-presenting cells (APCs) process antigens in their lysosomes, producing peptides that nestle in the peptide-binding groove of the HLAs. These are then transported to the cell surface for presentation to T lymphocytes together with costimulatory signals. Therefore, the density of HLAs on antigen-presenting cells is orders of magnitude higher than the density of any processed antigenic epitope. Also, each HLA is presented in the context of a myriad of peptides, whereas each peptide is presented in the context of, at most, a few HLAs. It follows that the frequency of cytotoxic T lymphocyte (CTL) precursors that can react against an HLA is orders of magnitude higher than the frequency of CTL precursors that can react against other alloantigens. For these reasons, the HLAs are the major histocompatibility antigens.
The HLAs are extremely polymorphic, with hundreds of different alleles identified at each locus. If these antigens were inherited randomly as unlinked loci, the chance of finding a fully matched donor for any patient would be vanishingly small. However, because they are closely linked (within less than 2 cM) on chromosome 6 (1), the HLAs
are inherited as “haplotypes,” and by Mendelian genetics each full sibling has a one in four chance of inheriting the same pair of haplotypes from both parents, and thus being genotypically HLA identical. Because of linkage dysequilibrium, some HLA haplotypes are reasonably common, and with ∼6 million volunteer donors enrolled in computerized registries, there is about a one in three chance of finding an unrelated donor who matches a patient phenotypically at both copies of the HLA-A, B, and DRB1 alleles, a so-called 6/6 antigen match. With the recognition that matching at HLA-C and DQB1 also affect unrelated donor BMT outcomes (2,3), phenotypic matching for 10 antigens is increasingly being carried out for patients who lack genotypically matched donors.
are inherited as “haplotypes,” and by Mendelian genetics each full sibling has a one in four chance of inheriting the same pair of haplotypes from both parents, and thus being genotypically HLA identical. Because of linkage dysequilibrium, some HLA haplotypes are reasonably common, and with ∼6 million volunteer donors enrolled in computerized registries, there is about a one in three chance of finding an unrelated donor who matches a patient phenotypically at both copies of the HLA-A, B, and DRB1 alleles, a so-called 6/6 antigen match. With the recognition that matching at HLA-C and DQB1 also affect unrelated donor BMT outcomes (2,3), phenotypic matching for 10 antigens is increasingly being carried out for patients who lack genotypically matched donors.
TABLE 50-1 Comparison of Solid Organ and Bone Marrow Transplantation. | |||||||||||||||||||||||||||
---|---|---|---|---|---|---|---|---|---|---|---|---|---|---|---|---|---|---|---|---|---|---|---|---|---|---|---|
|
The most important determinant of rejection and of GVHD is the extent of matching of the major histocompatibility antigens. However, there are many other factors. A randomized study was performed in an attempt to reduce the relapse rate of acute myeloid leukemia (AML) by increasing the dose of radiation in patients undergoing matched-sibling BMT. Patients who received the higher dose of radiation were less likely to relapse, but were more likely to die of GVHD (4). Conversely, delaying the infusion of donor lymphocytes by a few weeks after completion of the preparative regimen decreases their potency to cause GVHD (5). These observations led to extensive work in animal transplantation models that support the current theory that tissue injury leads to translocation of endotoxin and release of cytokines that activate recipient APCs to stimulate T cells present in the graft, increasing the likelihood of GVHD (6,7).
A unique feature of BMT, in contrast to solid organ transplantation, is that temporary immune suppression is sufficient. Generally, by 6 months after BMT, patients are weaned from immune suppression, and most do not develop GVHD. This tolerance develops because naive immune cells that mature within the recipient’s thymus undergo positive and negative selection on recipient antigens (8,9,10), and thus recapitulate normal immune ontogeny. In the absence of a functional thymus, immune reconstitution depends on the proliferation of postthymic cells present in the graft that have previously undergone selection in the donor’s thymus (11), and therefore may not provide fully potent immunity and may include lymphocytes that are alloreactive against the recipient. As a result, adults, whose thymuses are largely involuted, are at greater risk for GVHD than younger patients. Moreover, depletion of T lymphocytes from the graft can prevent GVHD (12,13), by restricting immune reconstitution to naive cells that must undergo thymic selection in the recipient’s thymus. However, a disadvantage of T-depleted BMT is that immune reconstitution is very slow in patients with poor thymic function.
INDICATIONS
The first successful BMTs were performed for patients with immune deficiencies (14,15). For certain forms of severe combined immune deficiency, a preparative regimen may not be necessary for the engraftment of T lymphocytes sufficient to ameliorate the condition (16). For less complete immune deficiency syndromes, a preparative regimen is administered, but BMT is often feasible even with partially HLA-mismatched donors.
For children with severe aplastic anemia, matched-sibling BMT after a cyclophosphamide-based preparative regimen is the treatment of choice (17). Not only is the survival superior to that of patients conventionally treated with cyclosporine, anti-thymocyte globulin (ATG), and prednisone, but also the secondary malignancy rate is less after BMT than the rate of clonal hematopoiesis after immune suppressive therapy. Moreover, approximately one-half of those who respond to immune suppressive therapy develop recurrent aplastic anemia, whereas recurrence after successful BMT is rare.
Any disorder of cells derived from the marrow is potentially curable by BMT. In Italy, because of the prevalence of thalassemia, blood donors are in short supply, and matched-sibling BMT is done early in the course of the
disease (18). Similarly, sickle cell anemia can be cured by matched-sibling BMT (19), and the use of unrelated donors is beginning to be explored. Neutrophil disorders are also curable with BMT. However, in contrast to T-lymphocyte disorders, these patients generally have an intact immunologic barrier to engraftment so the standard approach is a fully ablative preparative regimen for a fully HLA-matched donor.
disease (18). Similarly, sickle cell anemia can be cured by matched-sibling BMT (19), and the use of unrelated donors is beginning to be explored. Neutrophil disorders are also curable with BMT. However, in contrast to T-lymphocyte disorders, these patients generally have an intact immunologic barrier to engraftment so the standard approach is a fully ablative preparative regimen for a fully HLA-matched donor.
Many inherited metabolic diseases involve enzymes expressed in leukocytes. For these conditions, BMT may provide stem cells that provide a source of normal enzyme (20,21,22). The Kupffer cells of the liver and the microglial cells of the brain are derived from circulating monocytes, and can thus deliver the marrow donor’s normal enzyme to the critical organs involved in storage diseases (23). Surprisingly, osteopetrosis can be cured by BMT because the osteoclasts are also derived from circulating monocytes (24).
BMT is the treatment of choice for poor prognosis malignancies. The dose-limiting toxicity of many effective therapies, such as alkylating agents and radiation is marrow suppression. For these agents, a severalfold dose increase is possible if patients are rescued from the marrow toxicity by the infusion of a marrow graft. The ideal preparative regimen, then, involves the use of highly active agents with severe marrow toxicity, mild toxicity to other organs, and a steep dose response for log cell kill with no plateau (25). In addition, BMT constitutes a form of immunotherapy, in that the donor’s immune system can include alloreactive cells that can eliminate minimal residual disease, by mechanisms not cross-resistant with chemotherapy or radiation. The importance of this response is demonstrated by the survival advantage of BMTs from matched siblings versus identical twins (26), by the increased relapse rate after T-cell depletion of the marrow graft (27), and by the ability to induce remissions in patients who relapse after BMT by the simple infusion of lymphocytes from their marrow donor (28).
For acute leukemia, chronic myeloid leukemia, lymphoma, and neuroblastoma, BMT is not only the most effective therapy, but also the most toxic therapy. The decision of whether or when to use BMT to treat these malignancies then depends on balancing the risk of relapse using conventional therapy versus using BMT against the risk of transplant-related complications. The degree of HLA matching of the donor enters into this calculus because the use of partially mismatched donors increases the risk of graft rejection and GVHD, but simultaneously increases the graft versus leukemia effect and is therefore appropriate for patients with higher risk of relapse.
For children with AML, consolidation with BMT from matched related donors in first remission improves event-free survival, compared with the results of standard chemotherapy consolidation (29). Primary refractory AML carries a poor prognosis, but is occasionally curable by BMT. AML in second remission and beyond is an indication for alternative-donor BMT.
Very high-risk acute lymphoblastic leukemia (ALL), defined by such factors as presence of the Philadelphia chromosomal (9; 22) translocation (30,31) or infant ALL with the characteristic t(4; 11) translocation that rearranges the mixed-lineage leukemia (MLL) locus (32,33,34), are indications for matched-sibling BMT. Retrospective analyses of ALL treated in second complete remission with chemotherapy or matched-sibling donor BMT indicate that BMT approximately doubles EFS (35). Alternative donor BMT is an appropriate consideration for ALL in second remission after short first remission, or in third remission or beyond (36).
Allogeneic BMT remains the therapy of choice for chronic myeloid leukemia in children (37). The advent of Gleevec offers a less toxic alternative therapy (38), but at present it is not clear whether it offers acceptable long-term cure rates, and resistant clones that emerge during Gleevec therapy have additional mutations (39) that may reduce the cure rate of subsequent allogeneic BMT. Similarly, allogeneic BMT is appropriate initial therapy for myelodysplastic syndromes in children (40).
The conventional multimodal therapy for all types of lymphoma in children is sufficiently effective that BMT is reserved for patients who relapse. Although there is an allogeneic graft versus lymphoma effect (41), this benefit is generally offset by the increased risk of complications of allogeneic BMT as compared with autologous BMT. Thus, for most patients with chemotherapy-sensitive recurrent lymphoma, if the marrow is uninvolved, autologous transplantation is performed.
Neuroblastoma is the most common solid tumor in children. There are biologically distinct subtypes of neuroblastoma with low-risk disease typically presenting in infants and aggressive disease presenting in older children. The latter, high-risk neuroblastoma, is usually disseminated at initial presentation and is often associated with unfavorable biological characteristics such as N-myc amplification, high mitotic-karyorrhexis index, DNA index of 1, and loss of heterozygosity of the short arm of chromosome 1. Autologous BMT has been applied as consolidation for patients with high-risk neuroblastoma because these patients nearly always have initially chemosensitive disease, but the majority progress within 2 years of diagnosis when treated with conventional therapy. Multiple retrospective analyses (42) and a large randomized prospective study (43) have demonstrated the benefit of autologous BMT for high-risk neuroblastoma. Similar strategies are considered for other pediatric solid tumors that are similarly chemotherapy sensitive, but have poor ultimate prognoses.
Future directions include even more diverse potential applications for BMT. There is active experimental interest in the potential use of BMT to cure severe autoimmune diseases. Autologous BMT may be sufficient to abolish an autoreactive lymphocyte clone. If the trigger for autoreactivity is rare, as suggested by low concordance in identical twin studies (44), the autoimmune disease may not
recur. Also, because successful BMT results in tolerance to donor cells, it is theoretically possible that BMT may be used to tolerize a patient to a solid organ graft from the same donor. Another area of current investigation is the concept of stem cell plasticity (45), with the proposition that hematopoietic stem cells may give rise to cells that can contribute to the repair of nonhematopoietic tissues.
recur. Also, because successful BMT results in tolerance to donor cells, it is theoretically possible that BMT may be used to tolerize a patient to a solid organ graft from the same donor. Another area of current investigation is the concept of stem cell plasticity (45), with the proposition that hematopoietic stem cells may give rise to cells that can contribute to the repair of nonhematopoietic tissues.
PROCEDURES
Preparative Regimens
Before a marrow graft is transplanted, the patient first receives a preparative regimen that may consist of chemotherapy alone or in combination with total body irradiation (TBI). The preparative regimen is required to (1) overcome immunologic barriers to engraftment, (2) provide a competitive advantage for graft stem cells, and (3) eliminate abnormal or malignant host cells.
Traditional preparative regimens destroy the patient’s marrow cells and immune system, and are therefore referred to as ablative preparative regimens. In contrast, nonablative preparative regimens are less intensive; because the patient’s marrow is not destroyed, the graft must compete actively with the patient’s marrow cells, and improved posttransplant immune suppression is used to prevent rejection (46). The advantage of ablative preparative regimens is that they minimize the risk of graft rejection or graft failure, and for chemosensitive disease, maximize the cytoreduction of the tumor. Patients with poor organ function or active uncontrolled infection are unlikely to tolerate the toxicity of an ablative preparative regimen, and nonablative approaches may offer them an acceptable alternative. Reduced toxicities are desirable for patients with nonmalignant disorders, for whom maximum cytoreduction is not relevant. Also, for patients whose malignancies are chemotherapy resistant, the benefit of BMT depends on the allogeneic immune response to the tumor, so nonablative preparative regimens are appropriate.
The most common standard ablative preparative regimens for patients with hematologic malignancies undergoing allogeneic BMT are busulfan with cyclophosphamide (47) and cyclophosphamide with TBI (48). These regimens have demonstrated efficacy against the broad range of hematologic malignancies, and are sufficiently immune suppressive for HLA-matched allogeneic transplantation when the patient is not specifically alloimmunized against donor antigens. There are many variations of these regimens, including intensification by the addition of cytarabine or etoposide, or substitution of melphalan for cyclophosphamide. The degree of immune suppression can be increased by the addition of antithymocyte globulin or monoclonal antibodies directed at lymphocytes, such as Campath-1H.
When autologous transplants are performed to treat malignancy, the preparative regimen need not be highly immune suppressive or immune ablative. Instead, the focus is on antitumor efficacy with agents that are not cross-resistant with the standard therapy with which the patient has previously been treated. For lymphomas, standard regimens include carmustine, cyclophosphamide, and etoposide (49), and carmustine, etoposide, cytarabine, and melphalan (50). For neuroblastoma, standard regimens include cyclophosphamide, carboplatin, and etoposide (51); thiotepa and cyclophosphamide (52); thiotepa, topotecan, and carboplatin (53); and melphalan, etoposide, cyclophosphamide, and total body irradiation (TBI) (43,54).
GRAFT
The graft is harvested from the donor, either by repeated marrow aspiration from the hips or by mobilizing stem cells [using a cytokine such as granulocyte colony-stimulating factor (G-CSF)] from the marrow to the blood for collection by leukapheresis. Placental blood (also known as cord blood) is rich in hematopoietic stem cells and can also used for BMT. The advantage of PBSCs as the source of the graft is a 10-fold greater number of progenitor cells, and therefore significantly faster hematopoietic recovery (55). PBSC grafts also contain one to two orders of magnitude more T cells, which in the autologous setting is associated with faster immunologic reconstitution (56), but in the allogeneic setting is associated with a greater risk of chronic GVHD (57,58). Advantages of cord blood include the lack of risk to the donor, a much reduced risk of infectious exposure to the recipient, and a reduced risk of GVHD for any degree of match because of the immaturity of the donor’s immune system (59). The disadvantages include a limited cell dose, slower hematopoietic recovery, and unavailability of the unrelated cord blood donor for manipulations such as donor lymphocyte infusions.
Before the marrow harvest, the donor must be cleared for donation. It is essential to know whether the donor has any disease that can be transmitted in blood or marrow, and whether the donor has any condition that would increase his or her risk of donation. Standard evaluations include the American Association of Blood Banks donor screening history, serologic testing for HIV, cytomegalovirus (CMV), Epstein-Barr virus (EBV), syphilis, and hepatitis A, B, and C, a type and screen, and both a red blood cell cross-match and a lymphocytotoxic cross-match with the recipient. A screening history is obtained, and a physical exam is performed. Young children who are prospective donors for a sibling may be assigned an advocate who is not involved in the care of the recipient to consider the risks and benefits of the procedure from the standpoint of the donor.
For marrow harvesting, the donor is given either general anesthesia or regional anesthesia with sedation. The donor is positioned prone, with rolls placed under the hips and trunk to stabilize the torso with the hips slightly flexed to facilitate the palpation of bony landmarks. A wide area over the posterior iliac crests is prepared and draped. For patients receiving general anesthesia, instillation of additional local anesthesia over the periosteum can reduce postoperative pain.
With the trocar in place, the marrow aspirate needle is advanced past the area of cortical resistance so the tip is firmly lodged in the medullary space. The trocar is then removed and a 60-cc syringe is placed on the needle. The operator then vigorously pulls back the plunger of the syringe to generate maximal suction. After a few milliliters of marrow have been drawn into the syringe, the needle is rotated 180 degrees and advanced a few millimeters to access the deeper medullary space, and vigorous suction is again applied to the syringe. About 5 to 10 mL of marrow can be obtained from each marrow aspirate site before replacing the trocar and repositioning the needle. If larger volumes of marrow are aspirated without repositioning the needle, the graft will be diluted with more peripheral blood, and will contain fewer stem cells and progenitor cells, and more lymphocytes. Improved marrow harvest needles have been designed that have multiple side ports that access a greater portion of the surrounding medullary space.
The marrow is expelled gently from the syringe into a collection system that is anticoagulated, typically with ACD-A, or sufficient preservative-free heparin to produce a final concentration of 10 to 20 U per mL. Generally, for each kilogram of recipient weight, 10 to 15 mL of marrow will provide the required marrow dose of at least 2 to 3 × 108 nucleated cells per kilogram. Although this amounts to approximately 1L of marrow for a 70-kg recipient, and requires 100 to 200 marrow aspirates, the harvest is usually done through two or four skin sites (one on each iliac crest), repositioning the needle at various angles, taking care to avoid sciatic nerve injury. Occasionally, when a smaller donor is being harvested for a larger recipient, it may not be possible to obtain a harvest of optimal size from the posterior iliac crests. In this situation, it may be necessary to turn the patient supine and harvest the anterior iliac crests as well. In this case, it is important for the operator to avoid entering the joint capsule. When the marrow harvest is complete, the marrow is passed through screens or filters to prevent the infusion of bone fragments and clumps of cells that would embolize to the lungs.
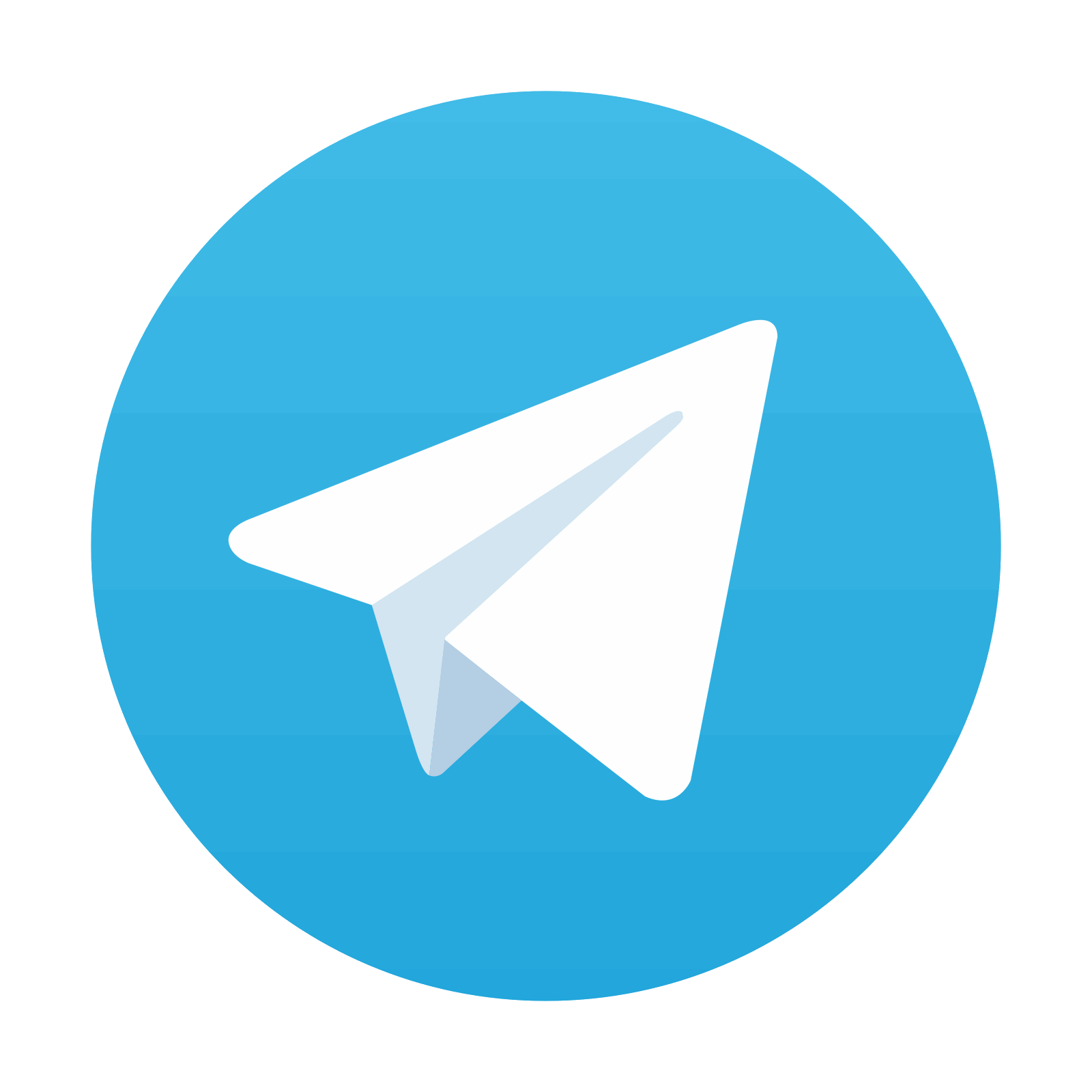
Stay updated, free articles. Join our Telegram channel

Full access? Get Clinical Tree
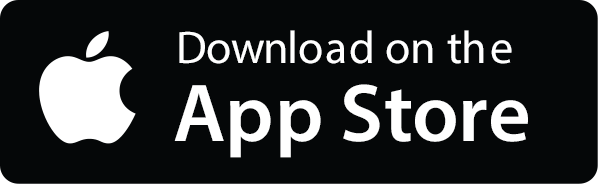
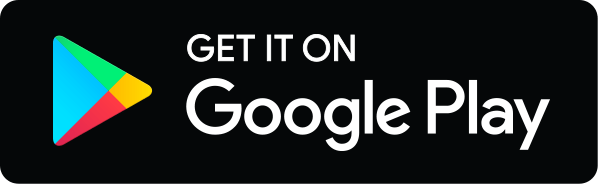