Biliary Atresia
Peter W. Dillon
Thomas F. Tracy Jr.
Department of Surgery, Penn State College of Medicine, Penn State Children’s Hospital, Milton S. Hershey Medical Center, Hershey, Pennsylvania 17033.
Department of Surgery and Pediatrics, Brown Medical School, Hasbro Children’s Hospital, Provi- dence, Rhode Island 02903.
Biliary atresia is one of the most serious digestive disorders of infants and young children (1). It is a process of uncertain pathogenesis that leads to fibrosis and obliteration of the extrahepatic ductal system resulting in obstruction to bile flow and cholestasis. Progressive injury to intrahepatic ductular structures and surrounding hepatic parenchyma through a destructive inflammatory process ultimately leads to cirrhosis and hepatic failure. If left untreated, the prognosis for biliary atresia patients is extremely poor with death usually occurring within 2 years. Since the introduction of the Kasai portoenterostomy in the 1960s, the treatment for biliary atresia has been surgical. With continued improvement in hepatic transplantation, the prognosis for infants with this disorder has improved dramatically. The strategy of sequential surgical therapy initially involving a portoenterostomy procedure followed by liver transplantation if needed is widely accepted. However, both surgical therapies serve only to palliate the disease process, and there are no specific therapeutic interventions targeted to influence the pathologic endogenous inflammatory process itself. For that matter, little is known about the etiology, pathologic mechanisms, or even possible treatment strategies for this disorder.
SURGICAL HISTORY
Burns described an infant with jaundice and acholic stools in 1817; this was the first recorded case of biliary atresia in the English language (2). In 1892, John Thompson, a physician in Edinburgh, described the clinical course and autopsy results of 49 patients with congenital obliteration of the bile ducts (3). J.B. Holmes, a pediatrician and pathologist at Johns Hopkins, reported 82 autopsy cases in 1916 and predicted the feasibility of a biliary-enteric anastomosis. His work served as the basis for the subsequent development of the classification of the disease process into “correctible” and “noncorrectable” types. Reports of surgical success with the “correctable” form of biliary atresia were published by Ladd in 1928 and Gross in 1940 (4,5). However, results from institutions elsewhere in the United States and in Europe were otherwise dismal, and the only surviving patients were in the “correctable” forms of biliary atresia, where an anastomosis could be made to a biliary cyst external to the liver surface. Results were so poor with “noncorrectable” forms of atresia that few patients were operated on or even actively treated until the mid-1960s. During this time, many surgical procedures were attempted with little success. These operations included partial hepatic resection with drainage, placement of intrahepatic drains, and drainage of hepatic lymph through the thoracic duct (6,7,8). At that time, it was the opinion of most surgeons in the United States that direct reconstruction of the extrahepatic biliary tract for noncorrectable atresia was futile. If surgery was to be considered, it was believed that delaying the procedure as long as possible was desirable in the hope that spontaneous resolution of the obstructive process would occur. There was concern that surgery would increase the postoperative mortality of neonatal hepatitis or cirrhosis. Even the operative procedures were conservative in nature because all attempts were made to preserve ductal structures so they would have a chance to reopen spontaneously in the postoperative period.
In Japan, Kasai’s work with biliary atresia patients began to challenge these concepts. In 1955, he noted that when the extrahepatic biliary tract was excised to the hilum, bile flow from the porta hepatis occurred. Over the next several years, he devised and perfected a procedure in which the structures in the porta hepatis were drained via a Roux-en-Y jejunal limb. Although his operation has since undergone numerous modifications, Kasai’s lasting contribution to the surgical treatment of biliary atresia was the
realization that the occluded extrahepatic ductal system could be isolated and dissected to the porta hepatis and that a functional enteric anastomosis could potentially be constructed to drain the remnant porta hepatis.
realization that the occluded extrahepatic ductal system could be isolated and dissected to the porta hepatis and that a functional enteric anastomosis could potentially be constructed to drain the remnant porta hepatis.
EPIDEMIOLOGY
Biliary atresia has a worldwide incidence of 1 in 8,000 to 17,000 live births, and it is more common in girls than in boys (9,10). Epidemiologic studies suggest that racial and environmental factors may play a role in its pathogenesis. In the United States, the incidence of this disorder is almost two times greater in African American than in white infants, and it is more common in Chinese than Japanese infants (11,12). Although several cases have been reported in families, the disease does not have a familial incidence, and twins have been reported to be both affected and only one affected (13,14,15,16).
Maternal factors relating to the disease process have been inadequately studied, but maternal age and parity appear to have no relationship (11). Infants tend to be of low birth weight and are usually full term as the disease is rarely seen in stillborns or premature infants. However, in a more recent study from Sweden biliary atresia was found with higher incidence in infants less than or equal to 32 weeks’ gestational age or when maternal age was greater than or equal to 35 years or parity was 4 or greater (17).
Several regional studies have shown an increased incidence of biliary atresia at certain times of the year leading some to suggest an infectious etiology. However, these data are conflicting and contradictory. An increased incidence of the disease was noted in Texas from 1972 to 1980 in late summer and fall, but in Atlanta from 1968 to 1993 winter and early spring clustering was reported (11,18). However, studies from Michigan and multiple countries in Europe have failed to demonstrate significant regional variation, seasonal variation, or space–time clustering (19,20,21,22,23,24). These conflicting reports may support a growing belief that biliary atresia is not a single disease but the result of complex processes yielding a common final inflammatory pathway targeting and ultimately destroying the extrahepatic bile ducts.
PATHOGENESIS
One of the greatest handicaps in dealing with biliary atresia is the fact that the etiology of this disease remains unknown. As a result of both clinical and experimental investigations, several general mechanisms have been proposed as potential causes of biliary atresia; however, no single theory has elicited a consensus. Potential initiating events include occult viral infection, environmental toxin exposure, a defect in ductal morphogenesis, a disorder of the immunologic or inflammatory system, and a defect in the fetal hepatic circulation (25).
The possibility that a viral infection in the pre- or perinatal period is the cause of biliary atresia has been a focus of clinical research efforts. Multiple lines of evidence in animal models have been developed suggesting that viruses are involved. It has been noted that liver specimens from biliary atresia patients exhibit pathologic findings consistent with viral infection including upregulation of Fas ligand, biliary epithelial apoptosis, and mononuclear cell activation within the portal tracts (26). The related hypothesis is that destruction of the intra- and extrahepatic bile ducts subsequently results from an immunologic reaction triggered by viral-induced neoantigens on the biliary epithelium. Specific viruses proposed as potential pathogens in this scenario include reovirus 3, cytomegalovirus (CMV), rotavirus C, human papillomavirus, and retroviruses. At present, however, the data remain inconclusive.
In the 1980s, it was found that a number of infants with biliary atresia demonstrated serologic reactivity to reovirus type 3 when compared with controls, and reovirus 3 particles were detected in the extrahepatic ductal tissue of an infant with biliary atresia (27,28,29). These studies were subsequently supported by molecular investigations in which RNA specific to reovirus was demonstrated in extrahepatic biliary tissue from biliary atresia patients (30). However, subsequent studies have failed to detect the presence of reovirus 3 in the disease (31,32). Investigations of other viruses have yielded similar conflicting results. Evidence of CMV infection has been found in a number of infants with biliary atresia, but not in others (33,34,35). Reports focused on rotavirus C and human papillomavirus have yielded similar contradictory results (36,37,38,39). More recently, possible retrovirus infection has also been reported (40). No association with biliary atresia has been found for one important group of hepatic viral infections, hepatitis A, B, and C (41,42). Although many investigators believe an infectious mechanism is likely, there are no definitive data implicating a specific agent as a causative factor. It is possible that a virus could initiate or contribute to this disorder depending on the presence of specific cofactors or conditions. Finally, it must be noted that these infections are relatively common and may be incidental and unrelated.
Environmental toxin exposure during pregnancy is a potential cause of biliary atresia, although there is no current evidence implicating a specific teratogen or environmental factor. Investigators have noted several studies reporting a time–space clustering of cases, possibly consistent with toxin or environmental exposure. One report suggests that farming or pesticide exposure and maternal infectious illness during the last trimester of gestation increased the risk of developing biliary atresia (10).
Primary pathology of the immune system, either congenital or acquired, could possibly result in an “autoimmune” process in which biliary epithelium and ductal structures are targeted. Circulating autoantibodies,
including antineutrophil cytoplasmic antibodies (ANCA), have been detected in biliary atresia patients (43). Additional support for this theory is derived from serologic studies of human leukocyte antigen (HLA) expression in affected infants, as well as from pathologic similarities to the adult processes of primary sclerosing cholangitis and primary biliary cirrhosis. An increase in the frequency of the HLA-B12 allele expression was noted in infants with biliary atresia as compared with controls (44). A subsequent study was unable to confirm these observations (45). An increased frequency of HLA-B8 expression has also been reported—an observation that is important given the association of this HLA allele with other autoimmune disorders (46). It is now recognized that HLA genotypes are not alone in their association with autoimmune disease processes, and that these can be more accurately defined by determining the variation in expression of a number of genes encoding immune regulatory proteins (47). Most important among these genes are those encoding the proinflammatory cytokines tumor necrosis factor alpha (TNF-α), interleukin 1 (IL-1), and the immunoregulatory cytokine interleukin 10 (IL-10). Using DNA genotyping, no association of biliary atresia has been demonstrated for HLA class I or II gene expression or for the common polymorphisms of the genes encoding IL-1, TNF-α, and IL-10. At present, it appears that biliary atresia is not an HLA-associated disease (48).
including antineutrophil cytoplasmic antibodies (ANCA), have been detected in biliary atresia patients (43). Additional support for this theory is derived from serologic studies of human leukocyte antigen (HLA) expression in affected infants, as well as from pathologic similarities to the adult processes of primary sclerosing cholangitis and primary biliary cirrhosis. An increase in the frequency of the HLA-B12 allele expression was noted in infants with biliary atresia as compared with controls (44). A subsequent study was unable to confirm these observations (45). An increased frequency of HLA-B8 expression has also been reported—an observation that is important given the association of this HLA allele with other autoimmune disorders (46). It is now recognized that HLA genotypes are not alone in their association with autoimmune disease processes, and that these can be more accurately defined by determining the variation in expression of a number of genes encoding immune regulatory proteins (47). Most important among these genes are those encoding the proinflammatory cytokines tumor necrosis factor alpha (TNF-α), interleukin 1 (IL-1), and the immunoregulatory cytokine interleukin 10 (IL-10). Using DNA genotyping, no association of biliary atresia has been demonstrated for HLA class I or II gene expression or for the common polymorphisms of the genes encoding IL-1, TNF-α, and IL-10. At present, it appears that biliary atresia is not an HLA-associated disease (48).
When first described, biliary atresia was believed to represent a defect in the morphogenesis of the biliary tract, hence the name. As many as 30% of infants with biliary atresia have coexisting anatomic anomalies including visceral organ asymmetry, offering support to the concept of abnormal embryogenesis as an etiology. Indirect support for this cause is found in more recent investigations of the inv mouse. Mutations in the inversin gene in homozygous mutants result in situs inversus with mirror image left–right inversions of stomach, liver, and spleen (49,50). Some of these animals have polysplenia, preduodenal portal vein, and intestinal malrotation—clinical findings associated with biliary atresia. These mice become progressively jaundiced after birth with marked abnormalities of the intrahepatic bile ducts and a histologic picture that resembles biliary atresia (51). The potential involvement of this gene in patients with biliary atresia and situs anomalies is an area of current research. Abnormalities in the morphogenesis and differentiation of intrahepatic bile ducts have been described in the livers of some infants with biliary atresia (52,53,54). It has been proposed that interruption of the normal remodeling process of the primitive ductal plate may result in persistence of this structure and inadequate bile duct lumen formation. Histologic evidence of ductal plate malformation has been observed in cases of extra- and intrahepatic atresia of the bile ducts and has also been associated with abnormalities in the hepatic artery (55,56). Elucidation of the molecular regulatory factors potentially responsible for such an abnormality may come from ongoing investigations in a murine knockout model. In the mouse, it has been found that ductal plate malformations occur in the absence of the transcription factors hepatocyte nuclear factor-6 (HNF-6) and hepatocyte nuclear factor 1B (HNF-1b) (57,58). Other signaling pathways such as Jagged-1/Notch, deficient in Alagille syndrome, may also be important in bile duct morphogenesis (59).
The concept that local ischemia during fetal hepatobiliary development may contribute to the pathogenesis of biliary atresia has been the focus of renewed interest. The close relationship between the development of the hepatic vasculature and the biliary system is well recognized. During the course of normal biliary development, the epithelial ductal plates of the developing intrahepatic bile ducts induce vasculogenesis in the portal mesenchyme resulting in development of hepatic artery branches. These structures in turn influence development of the embryonic bile ducts in the portal mesenchyme (56). Arteriopathy resulting in abnormal vascular–ductal interactions is a possible mechanism in the development of biliary atresia. Thickened, hyperplastic arterioles with medial hypertrophy and concentric fibrosis have been found in resected ductal specimens (60,61). These findings may indicate the presence of ductal ischemia during development, but whether they represent a primary or secondary event in the development of biliary atresia remains to be determined.
Because a rational argument can be made for a number of the hypotheses, the current concept among most experts is that biliary atresia is the late clinical manifestation of complex and heterogeneous processes that can likely be initiated by a number of different events (62).
Regardless of initial etiology, a contributing factor to the progression of bile duct destruction may be extravasation of bile into tissues. Extravasated bile has been detected in excised bile duct specimens (63). The inflammatory response triggered by the presence of extraluminal bile may perpetuate ductal destruction and, ultimately, obstruction. Progressive cholestasis is associated with hepatic parenchymal accumulation of toxic, hydrophobic bile acids linked in turn to hepatocyte necrosis and apoptosis. The mechanisms of bile acid-induced apoptosis include oxidative stress with cellular depletion of adenosine triphosphate (ATP), mitochondrial injury, stimulation of caspase 8, and activation of caspase 9 and 3 (64). Antioxidant therapy may modify these apoptotic mechanisms and protect cellular structures (65).
EMBRYOLOGY OF THE BILIARY SYSTEM
The human liver and its accompanying biliary ducts begin visible development in the third week of gestation (66). The hepatic diverticulum develops as a bud from the ventral surface of the foregut and invades the mesenchymal
tissue of the septum transversum. The septum transversum is the mesenchymal tissue plate that separates the developing embryonic thoracic and abdominal cavities. By the seventh week of gestation, primitive portal tracts are established following the ingrowth of a sinusoidal vascular network from the vitelline vein. Interactions with surrounding mesenchyme yield mature portal venous structures. The intrahepatic ductal system forms from hepatoblasts adjacent to the mesenchyme in the portal tracts beginning in the eighth week of gestation. These hepatoblasts differentiate into biliary epithelial cells and form the ductal plate—first a single and then a double layer of cells surrounding the portal tract. Lumena develop within the two layers of the ductal plate and coalesce into tubular structures. By the eleventh week of gestation, these structures have become centrally located within the portal tract, whereas the remaining ductal plate components resorb. Subsequent maturation of the developing biliary tree takes place from the porta hepatis outward through the liver. Maturation of the entire intrahepatic biliary tree continues through birth and for several weeks thereafter.
tissue of the septum transversum. The septum transversum is the mesenchymal tissue plate that separates the developing embryonic thoracic and abdominal cavities. By the seventh week of gestation, primitive portal tracts are established following the ingrowth of a sinusoidal vascular network from the vitelline vein. Interactions with surrounding mesenchyme yield mature portal venous structures. The intrahepatic ductal system forms from hepatoblasts adjacent to the mesenchyme in the portal tracts beginning in the eighth week of gestation. These hepatoblasts differentiate into biliary epithelial cells and form the ductal plate—first a single and then a double layer of cells surrounding the portal tract. Lumena develop within the two layers of the ductal plate and coalesce into tubular structures. By the eleventh week of gestation, these structures have become centrally located within the portal tract, whereas the remaining ductal plate components resorb. Subsequent maturation of the developing biliary tree takes place from the porta hepatis outward through the liver. Maturation of the entire intrahepatic biliary tree continues through birth and for several weeks thereafter.
Development of the extrahepatic system is not as well elucidated. The gallbladder and extrahepatic ductal system arise from the caudal portion of the hepatic diverticulum, whereas the cranial portion of the diverticulum fuses with the septum transversum (67). Throughout development, the intrahepatic and extrahepatic biliary systems maintain their patency and continuity, and at no time during normal gestation has a solid phase been identified in ductal system formation (68).
A number of complex and redundant interactions occur in the genetic control of hepatic and biliary system organogenesis (69). In early gestation, the domain-specific expression of the Foxa (forkhead box a; formerly Hnf3, hepatocyte nuclear factor-3) proteins in the endoderm control the initial stages of hepatic development. The foxa proteins regulate almost all the liver-specific genes as well as genes in the lung and pancreas. A subclass of the GATA zinc-finger transcription factors has also been found to regulate liver-specific gene expression as well as endoderm-specific signals from bone morphogenic proteins (Bmps) (69,70). Bmps appear to be strongly expressed in the septum transversum mesenchyme. Other known regulatory factors include fibroblast growth factor (Fgf), hepatocyte growth factor (Hgf), vascular endothelial growth factor receptor 2 (Vegfr-2), and transcription factors such as haematopoietically expressed homeobox (Hex), prospero-related homeobox 1 (Prox1), and c-Met (Hgf receptor). A number of factors are required for tissue growth and generation of hepatic structures including Kras (Kirsten rat sarcoma oncogene), c-Jun, Sek1, and nuclear factor-6β pathway components (NF-6β).
At present, regulation of biliary tract morphogenesis is not fully understood; however, hepatic nuclear factor 6 (Hnf6) is expressed in embryonic liver tissue, hepatic ducts, and the gallbladder. Along with Hnf1b, it exerts control over biliary duct development (57). Expression of the forkhead transcription factor Foxf1 in the septum transversum mesenchyme has also been shown to regulate development of the extrahepatic ductal system including the gallbladder (71). In addition, Jagged 1 (JAG 1), a notch ligand, expression has been localized to the ductal plate tissues starting in the fourteenth week of gestation with subsequent expression on biliary epithelium in postnatal liver (72). Mutations in Jagged 1 expression have been associated with Alagille syndrome and may be associated with biliary atresia (73,74). Further studies will elucidate the roles of these factors in the development of this disease process.
Fetal studies have shown that bile formation begins at approximately 12 weeks gestational age and increases in volume until birth. However, in the full-term neonate bile formation is markedly low compared with adult levels (75). A number of factors are responsible for this age-dependent difference. Decreased bile salt uptake by the immature liver contributes to a smaller neonatal bile acid pool as compared with adults. In addition, ileal transport of bile acids is decreased in the neonatal period resulting in higher losses of bile salts in the stool and therefore decreased recirculation (76). Because bile acids are the primary stimulus for bile secretion, the smaller size of the bile salt pool and the immature enterohepatic system combine to limit the rate of bile formation (77).
Immaturity of the bile acid system is also associated with the presence of atypical bile acids in the meconium and stool of neonates. These atypical bile acids consist of multiple hydroxylated species and may be hepatotoxic (78,79). Their presence during development in a cholestatic process such as ductal obstruction may contribute to the associated inflammatory reaction.
PATHOLOGY
Characteristic pathologic findings in biliary atresia include portal tract edema, bile duct proliferation, portal and periductalar inflammation, and associated areas of hepatic cell injury (Fig. 92-1). Additional histologic findings within the liver parenchyma include bile duct plugs, giant cell proliferation, haemosiderin deposition, and increased hematopoietic activity. Longitudinal studies have shown the progressive nature of these processes even with surgical therapy (80). Macroscopic examination of the involved extrahepatic ductal system usually shows fibrous obliteration of the common and hepatic ductal structures with an absent or atretic gallbladder. The gallbladder may contain “white bile”—clear mucous—with no pigment. In severe cases, the liver is dark green in color, congested, and large and firm in texture. Nodular changes in the hepatic capsule consistent with the development of cirrhosis may be
evident. Histologic examination of livers with advanced disease shows evidence of progressive micronodular cirrhosis with bridging fibrosis between portal tracts, as well as portal destruction. Beyond 6 months of age, the ductular proliferation in the portal tracts disappears and is replaced by collagen deposition.
evident. Histologic examination of livers with advanced disease shows evidence of progressive micronodular cirrhosis with bridging fibrosis between portal tracts, as well as portal destruction. Beyond 6 months of age, the ductular proliferation in the portal tracts disappears and is replaced by collagen deposition.
Histologic studies of resected extrahepatic ductal specimens have shown that ductal involvement with the disease process is quite variable (81,82). In some cases, the common bile duct will be completely obliterated with a fibrotic reaction and very little evidence of inflammation. In other patients, there may be one or more ductlike structures patent within the fibrous tract to the porta hepatis. The lumens of these structures are variable in size, often 30 to 80 μm in diameter. The epithelial cell lining is usually destroyed in this circumstance, and inflammatory cell infiltrates are present in the surrounding connective tissue. A third group consists of specimens in which microscopic ductular structures with lumens greater than 100 μm in diameter are found. The epithelial cell lining may be partially intact, but the surrounding tissues demonstrate fibrosis and mononuclear cell infiltrates. Ductal size and structure have been reported to correlate with bile flow following surgical correction, but this has been a point of considerable controversy and questionable clinical import (83).
Extensive proliferation of the bile ductules within the portal tracts is one of the crucial pathognomonic findings for biliary atresia. However, this can also be seen in other hepatic disease processes. The origin of these newly formed bile ductules appears to be from hepatocytes positioned around the ductal plate (84). Increased and unregulated cell proliferation of the bile ducts is believed to be responsible for this observation. Abnormal biliary epithelial cell expression of Fas ligand and other markers of apoptosis cell proliferation have been noted in liver specimens from patients with biliary atresia (85,86). In addition to the proliferation of bile ductules, abnormalities of the larger intrahepatic bile ducts are found. Specifically, a paucity of interlobular ducts or ductopenia is reported (87,88,89). Cytokeratin immunostaining for CK 7 and CK 19 subtypes may help in the identification of these ductal structures (90).
Histologic differentiation of other hepatic disease processes and biliary atresia based on a liver biopsy specimen alone is difficult. Mononuclear cell infiltrate within the hepatic parenchyma, giant cell transformation, ductular proliferation, and ductopenia are also seen in neonatal hepatitis, alpha-1 antitrypsin deficiency, TPN-induced cholestasis, and the syndromic and nonsyndromic forms of intrahepatic bile duct paucity (Alagille’s syndrome).
The original classification of biliary atresia into correctable and noncorrectable forms has been refined by the Japanese Society of Pediatric Surgeons into three types (91). This morphologic classification system is based on gross findings and operative cholangiograms:
Type I—atresia of the common bile duct
Type II—atresia of the common hepatic ducts
Type III—atresia of the right and left hepatic ducts (i.e., the porta hepatis)
Two additional subclassifications are described according to the patterns of the distal extrahepatic bile ducts and ductular structures at the porta hepatis. Almost 90% of all cases were found to be type III.
IMMUNOLOGY OF BILIARY ATRESIA
The pathology of ductal destruction is not unique to biliary atresia. Bile ductules are also preferentially destroyed in liver allograft rejection, drug-induced ductopenia, and primary biliary cirrhosis (92). Biliary epithelial cells express a number of ligands in both healthy and diseased states that are important in controlling or initiating various immune and inflammatory reactions. Cell surface proteins such as the ABO blood group determinants, major histocompatibility complex (MHC) class I determinants, and integrin receptors are constitutively expressed on biliary epithelial cells. The expression of MHC class II glycoproteins and the adhesion molecules—intercellular adhesion molecule-1 (ICAM-1), LFA-3, NCAM, and CD-51—can be induced by cytokine stimulation. Thus, it appears biliary epithelial cells are active participants in the immunologic and inflammatory events associated with their destruction.
The basis for understanding the potential role of the inflammatory response in biliary atresia was established by Landing in his observations on the nature of the process he termed infantile obstructive cholangiopathy (93). He proposed that the disease resulted from an acquired
inflammatory process and offered the following observations:
inflammatory process and offered the following observations:
No laboratory data will be wholly able to differentiate neonatal hepatitis from biliary atresia.
Liver biopsy and cholangiograms are the most effective ways of differentiating the diagnosis.
No surgical mode of therapy will cure a significant fraction of infants with biliary atresia because the disease process actually destroys intrahepatic bile ducts as well. Biliary atresia is more in need of preventive or prophylactic measure than of new surgical procedures.
Because the obliterative process can resolve, even if not usually completely, surgical procedures of the Kasai type (portoenterostomy) should be considered for all infants.
Since these observations of Landing, a significant amount of information has accrued regarding immunologic events associated with biliary atresia. MHC expression on hepatic and ductal structures has been found to be quite variable in cases of biliary atresia. Class I determinants are constitutively expressed on all nucleated cells within the liver, and no differences have been detected between control and diseased livers (94). Variable expression of the class II determinant HLA-DR on biliary epithelial cells, Kuppfer cells, sinusoidal lining cells, and hepatocytes has been reported (80,94,95,96,97,98). It has been proposed that HLA-DR expression may be related to the severity and time course of the disease with strongest expression linked to progressive bile duct and hepatic parenchymal destruction (99).
Adhesion molecule expression is believed to play an important role in the immune-mediated destruction of ductal structures and surrounding hepatic parenchyma. ICAM-1 appears to be the most important adhesion molecule, and its expression has been demonstrated on intra- and extrahepatic ductal structures including proliferating bile ductules and interlobular ducts (97,98,100). Hepatocytes and sinusoidal lining cells in areas of active inflammation also express ICAM-1. Variable expression of vascular cell adhesion molecule-1 (VCAM-1) has been demonstrated on bile duct structures and sinusoidal lining cell membranes in areas of active inflammation. E-selectin expression has been detected on sinusoidal endothelium but not on any ductal structures.
In contrast, bile ducts in livers with cholestatic injury or with hepatitis fail to express ICAM-1, and no other cause of cirrhosis has been found to induce ICAM-1 expression on ductal structures. The only other hepatic disease processes known to be associated with increased ductal expression of ICAM-1 are primary biliary cirrhosis and primary sclerosing cholangitis. Thus, it appears that, in those diseases where ductal destruction is central to the pathologic process, ductal ICAM-1 expression is a unique and important finding.
The inflammatory cell infiltrates associated with biliary atresia result from specific pathways of immunologic activation. Lymphocyte infiltration of portal areas and lymphocytes within the biliary epithelial layer have been reported (101). Mononuclear cell populations with demonstrated involvement in the pathogenesis of biliary atresia include macrophages, natural killer (NK) cells, B cells, and T cells (80,97,98,102,103). In both portal and parenchymal infiltrates, all lymphocytes have been found to express lymphocyte function-associated antigen-1 (LFA-1)—the ligand for ICAM-1—and HLA-DR determinants. In terms of T-cell populations, CD4+ lymphocytes significantly outnumber CD8+ cells in areas of portal and parenchymal infiltration, and those CD8+ present in the portal region appear to have their cytotoxic activity downregulated (104). Expansion of the hepatic macrophage population has also been demonstrated and may correlate with the severity of the disease process (98,99,102). Macrophage activation with the upregulation of CD14—the lipopolysaccharide (LPS) receptor—occurs as part of the inflammatory process (102,105). These activated macrophages are a potential source of cytokine generation, a process known to stimulate the inflammation, fibrosis, and ultimately cirrhosis (106).
Proinflammatory peptides, including the chemokines RANTES, IP-10, MIP-1α, MIP-1β, and IL-8 as well as the growth factor TGF-β1, have been detected in the livers of biliary atresia patients (107,108). Increased gene expression of the proinflammatory cytokines osteopontin and interferon (IFN)-γ has also been reported (109). These data indicate a commitment to lymphocyte-mediated inflammation through a number of different pathways including TH1-like cytokines, but whether these are primary pathogenic events or secondary to a self-perpetuating inflammatory process is not known.
However, elevated serum levels of several cytokines are reported in biliary atresia patients. These may reflect the stage of the disease or response to therapy. Both IL-1B and IL-6 levels were elevated in patients with failed Kasai procedures, but normal in those with a successful operation (110). Interferon-inducible protein-10—a chemokine produced in response to lipopolysaccharide, IFN-γ, IL-1β, or TNF-α—is also elevated in the sera of biliary atresia (111). Elevated levels of IL-18—a cytokine with the capacity to stimulate IFN-γ production from activated T cells and NK cells and induce ICAM-1 expression—have also been detected (106). Although inflammatory cytokines are clearly involved, serum levels of these cytokines cannot be used as reliable indicators of disease activity.
A number of other serum moieties have been proposed as markers of disease severity. Hyaluronic acid levels have been found to be elevated in patients with biliary atresia and have a direct relationship to the severity of hepatic fibrosis (112). Normally cleared by hepatic sinusoidal endothelial cells, hyaluronic acid levels in serum are elevated
if progressive hepatic destruction sufficiently impairs uptake. However, this process is not specific to biliary atresia. In known biliary atresia patients, high serum concentrations may identify infants with significant hepatic compromise and a poor prognosis (113). Plasma endothelin-1 levels also correlate with the severity of liver cirrhosis and portal hypertension in biliary atresia patients (114). Low levels of serum insulin-like growth factor-1 (IGF-1) have been reported in patients with biliary atresia, and the lowest levels noted are in infants requiring hepatic transplantation (115). Soluble ICAM-1 and VCAM-1 are also elevated in the serum of biliary atresia infants (116,117). These may be as useful for monitoring the degree of active inflammation in biliary atresia patients as they are for other conditions (118).
if progressive hepatic destruction sufficiently impairs uptake. However, this process is not specific to biliary atresia. In known biliary atresia patients, high serum concentrations may identify infants with significant hepatic compromise and a poor prognosis (113). Plasma endothelin-1 levels also correlate with the severity of liver cirrhosis and portal hypertension in biliary atresia patients (114). Low levels of serum insulin-like growth factor-1 (IGF-1) have been reported in patients with biliary atresia, and the lowest levels noted are in infants requiring hepatic transplantation (115). Soluble ICAM-1 and VCAM-1 are also elevated in the serum of biliary atresia infants (116,117). These may be as useful for monitoring the degree of active inflammation in biliary atresia patients as they are for other conditions (118).
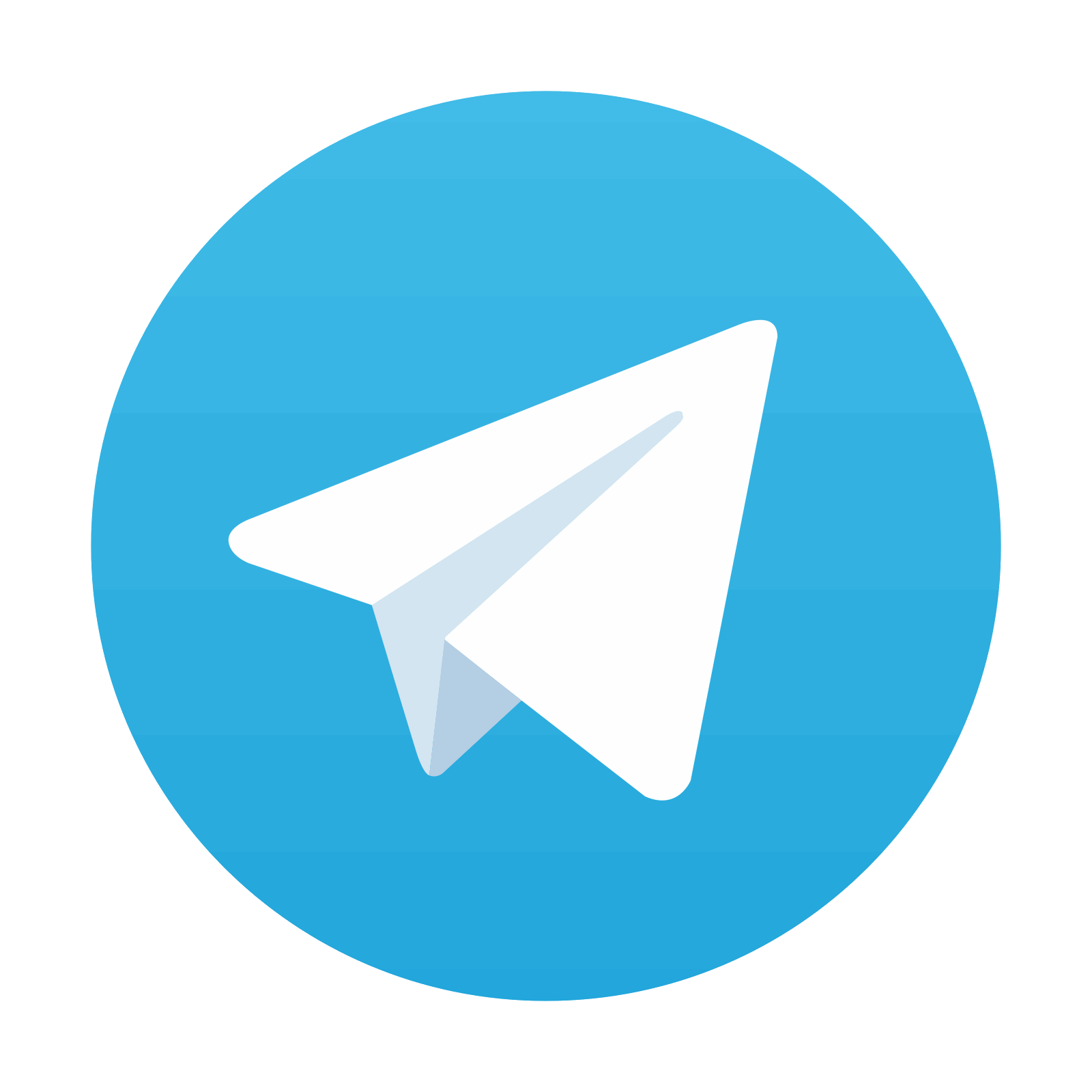
Stay updated, free articles. Join our Telegram channel

Full access? Get Clinical Tree
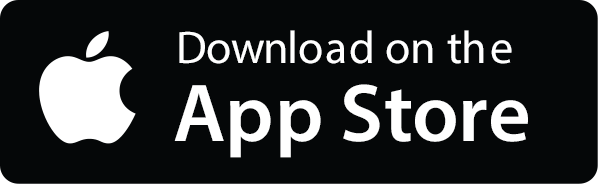
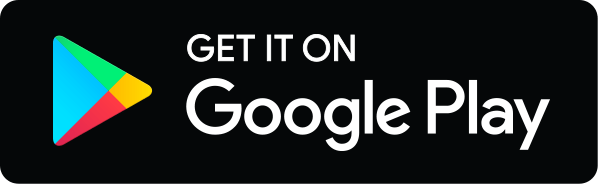