Angiogenesis and Vascular Anomalies
Arin K. Greene
Steven J. Fishman
Division of Plastic Surgery, Harvard Medical School, Brigham and Women’s Hospital, Boston, Massachusetts 02115.
Department of Surgery and Vascular Anoma- lies Center, Children’s Hospital Boston, Harvard Medical School, Boston, Massachusetts 02115.
ANGIOGENESIS
Angiogenesis is the process by which new blood vessels are formed from preexisting vasculature, leading to neovascularization. Almost every cell in the body lives within the oxygen diffusion distance of 100 to 200 μm from a capillary. Endothelial cells are normally quiescent, with less than 0.01% of cells proliferating and a turnover time of more than 1,000 days. In contrast, the turnover time for bone marrow is 5 days (1).
During angiogenesis, endothelial cells exit their resting state and proliferate as fast as bone marrow cells. Angiogenesis is broadly divided into physiologic angiogenesis and pathologic angiogenesis (Table 34-1). Physiologic angiogenesis, such as occurs in wound healing, the development of adipose mass (2), and liver regeneration (3), is tightly controlled with a finite end point. Pathologic angiogenesis, in contrast, is dysregulated and persistent.
Pathologic angiogenesis can be subdivided into neoplastic and nonneoplastic diseases. Uncontrolled angiogenesis supports the growth of tumors (one endothelial cell can support 5 to 100 tumor cells), provides an entry for inflammatory cells into sites of chronic inflammation (Crohn’s disease), causes blindness, leads to growth and hemorrhage of atherosclerotic plaques, and is responsible for the growth of hemangiomas (1).
The field of angiogenesis developed by studying neoplastic tissue. In 1971, Folkman proposed that the growth of tumors was dependent on the vasculature (4). He showed that tumor growth was retarded in the absence of tumor vascularization (5,6). In addition, he proposed that antiangiogenic therapy could potentially prohibit tumor growth (7). The field of angiogenesis then progressed with the ability to grow capillary endothelial cells in vitro (8), as well as after the discovery of the first angiogenesis inhibitor (9) and with the later purification of the first proangiogenic protein (10).
The Angiogenic Switch
Most neoplastic tissue begins as in situ, microscopic lesions between 0.2 to 2 mm in diameter (1). Carcinomas that have activated the angiogenic switch become clinically detectable, cause local bleeding, and metastasize. Several processes turn on angiogenesis by shifting the balance between angiogenesis stimulators and inhibitors (11,12). For example, overexpression of the ras oncogene increases the production of vascular endothelial growth factor (VEGF), whereas deletion of the p53 tumor suppressor decreases the production of the angiogenesis inhibitor, thrombospondin. Hypoxia increases the production of VEGF, thus promoting the growth of neoplastic tissue as the center of a tumor becomes compressed and hypoxic (13).
Angiogenesis Stimulators
A family of proteins has been discovered that regulates the process of angiogenesis. Of the angiogenesis stimulators, basic fibroblast growth factor (bFGF) and VEGF are the most commonly identified proteins involved in angiogenesis (10,14). Fibroblast growth factor (FGF) is stored in the extracellular matrix, and promotes endothelial cell mitosis and migration. It is mobilized from the extracellular matrix by proteinases and heparanases. FGF can stimulate several targets, including fibroblasts, smooth muscle cells, and neurons, but is selective for endothelial cells. Elevated levels of bFGF are found in the urine and serum of patients with cancer (15).
VEGF is a specific endothelial cell mitogen that exists as five isoforms of 121, 145, 165, 189, and 206 amino acids. VEGF165 is the most common isoform found in neoplastic tissues. VEGF binds to the tyrosine kinase (Flt-1) and the kinase insert domain-containing receptor on endothelial cells. Homologues of VEGF, including VEGF-B, -C, -D, and -E have been recently discovered. VEGF-C stimulates the growth of lymphatic endothelium by binding to Flt-4 (1). Angiopoietin-1 is a peptide that stabilizes blood vessels by binding to the tyrosine kinase receptor Tie-2 on endothelial cells, stimulating the recruitment of pericytes and smooth muscle cells into the vessel wall. Angiopoietin-2 inhibits the Tie-2 receptor and destabilizes the vasculature. Along with VEGF, angiopoietin-2 increases angiogenesis.
TABLE 34-1 Examples of Angiogenesis-mediated Processes. | ||||
---|---|---|---|---|
|
Angiogenesis Inhibitors
A tumor that produces proangiogenic proteins to support its own growth can also secrete antiangiogenic proteins to inhibit the growth of its metastases. This observation led to the discovery of angiostatin, a 38-kDa cleavage product of plasminogen, which inhibits tumor growth when administered exogenously (12). Endostatin, a 20-kDa cleavage product of collagen XVIII was generated by murine hemangioendothelioma (16). Human non-small cell lung carcinoma produces a 53-kDa cleavage product of antithrombin III (antiangiogenic ATIII) (17). These proteins block tumor growth by specifically inhibiting endothelial cells. Numerous other endogenous inhibitors of endothelial cell proliferation have been described (9,12).
Angiogenesis in Nonneoplastic Diseases
Many nonneoplastic diseases are now known to be angiogenesis dependent (1). For example, pathological angiogenesis is the most common cause of blindness. Macular degeneration is the leading cause of blindness in people older than 64 years of age. In macular degeneration, angiogenesis develops in the choroids and microhemorrhages of these new vessels cause loss of vision (18). Diabetic retinopathy is the most common cause of blindness in people between 25 and 64 years of age, and is characterized by development of pathologic angiogenesis in the retina to induce vessel growth into the vitreous.
VASCULAR ANOMALIES
Vascular anomalies are disorders of the endothelium that usually present during childhood. These lesions affect all parts of the vasculature: capillaries, veins, arteries, or lymphatics. Although nearly always benign, vascular anomalies may involve any location and are often locally destructive. In addition to severe disfigurement, local complications include obstruction, bleeding, bone loss, and pain. Systemic sequelae can include thrombocytopenia, pulmonary embolism, congestive heart failure, and even death.
The field of vascular anomalies requires interdisciplinary cooperation between medical and surgical specialties. This topic is confusing because many practitioners still refer to vascular anomalies by their descriptive, rather than histologic terms. For example, hemangioma, a tumor, has been referred to as a “strawberry hemangioma,” whereas a venous malformation, a congenital anomaly, is often incorrectly called a “cavernous hemangioma.” Consequently, communication, diagnosis, and treatment of patients afflicted with these lesions remains difficult.
A biologic classification of vascular anomalies based on physical findings, natural history, and cellular characteristics has clarified the differences between the different types of vascular anomalies (19). In particular, vascular anomalies are broadly divided into two groups: tumors and malformations (Table 34-2). Vascular tumors are characterized by endothelial cell proliferation. Vascular malformations, in turn, arise from dysmorphogenesis and have normal endothelial cell turnover.
The list of vascular tumors includes hemangioma, tufted angioma, and kaposiform hemangioendothelioma. Malformations, which may involve any part of the vasculature, are further subdivided into slow-flow lesions [capillary malformations (CMs), venous malformations (VMs), lymphatic malformations (LMs)] or fast-flow lesions [arterial malformations such as aneurysm, ectasia, stenosis, fistulas, or arteriovenous malformation (AVM)]. Finally, combined vascular anomalies exist that
carry eponyms based on the physician responsible for the initial description. One example of a combined vascular anomaly is Klippel-Trenaunay syndrome (KTS), a capillary-lymphatico-venous malformation associated with soft tissue or skeletal hypertrophy.
carry eponyms based on the physician responsible for the initial description. One example of a combined vascular anomaly is Klippel-Trenaunay syndrome (KTS), a capillary-lymphatico-venous malformation associated with soft tissue or skeletal hypertrophy.
TABLE 34-2 Biological Classification of Vascular Anomalies | ||||||||||||||
---|---|---|---|---|---|---|---|---|---|---|---|---|---|---|
|
Great progress in understanding and treating patients with vascular anomalies has been made over the past quarter of a century. For example, radiographic imaging is now the standard for diagnostic confirmation, rather than surgical biopsy. Antiangiogenic drug treatment is available for problematic hemangiomas, sclerotherapy has replaced operative resection in many instances, and techniques for excision have been improved. Several multidisciplinary Vascular Anomalies Centers now serve as regional, national, or international referral centers for patients with these lesions.
Nosologic Features
Vascular anomalies most commonly involve the skin, and thus are usually noted at birth. They often look similar, as either flat or raised lesions that may be blue or red. Historically, these lesions were referred to by what food they resembled (“cherry,” “strawberry,” “port-wine stain”) (20). After the development of histopathology in the nineteenth century, vascular anomalies were often referred to as angiomas. Virchow, the father of pathology, was the first to attempt to categorize vascular anomalies based on histologic characteristics. He divided vascular anomalies into angioma simplex (superficial “capillary” or “strawberry” hemangioma”), angioma cavernosum (subcutaneous hemangioma or venous malformation), or angioma racemosum (arteriovenous malformation) (21). His student, Wegener, attempted to classify lymphatic malformation as “lymphangioma” or “cystic hygroma” (22).
The modern classification of vascular anomalies is based on cellular traits, correlated with physical findings and natural history (19). The suffix –oma describes a lesion that formed by upregulated cellular growth. Thus, this suffix is used for vascular tumors (hemangioma, tufted angioma, kaposiform hemangioendothelialoma). Terms such as cystic hygroma (macrocystic lymphatic malformation), lymphangioma (microcystic lymphatic malformation), or cavernous hemangioma (venous malformation), which describe nonproliferating malformations, have been abandoned. Although tumors and malformations may be diagnosed by history and exam in 90% of cases, radiologic and histologic studies may confirm the diagnosis (23).
Vascular Tumors
Hemangioma and Angiogenesis
Hemangioma, a benign tumor of the endothelium, is the most common neoplasm of infancy. Capillary or strawberry hemangioma has previously been used to describe hemangioma involving the dermis, which appeared red. Hemangiomas deep to the dermis may appear bluish and have been referred to as cavernous hemangioma. The terms capillary and cavernous also have been used to describe CM and VM, respectively. The terms capillary, strawberry, and cavernous confuse diagnosis and should not be used. Insight into the pathophysiology of hemangioma has paralleled the development of the field of angiogenesis. Ninety percent of hemangiomas may be diagnosed by history and physical exam. These lesions have unique growth stages, a proliferating phase until 1 year of age, an involuting phase from 1 to 7 years of age, and finally an involuted phase after 7 years of age.
The stages of hemangioma growth are dependent on pro- or antiangiogenic stimuli on the endothelial cell. During the proliferating phase, when the hemangioma is rapidly enlarging, microvessel density increases as demonstrated by the increased expression of markers of mature endothelium (CD31, von Willebrand factor, Ulex europaeus lectin I, VE-cadherin, E-selectin) (24,25,26). The potent angiogenic peptides, bFGF, and VEGF are upregulated during hemangioma proliferation (24). During proliferation, the epidermis overlying the hemangioma expresses increased proangiogenic FGF and decreased antiangiogenic interferon beta (IFN-β), promoting a proangiogenic environment (27). In addition, matrix metalloproteinases are upregulated to assist in the breakdown of the extracellular matrix during angiogenesis (24).
During the involuting phase, when the hemangioma decreases in size, endothelial turnover is downregulated. The angiogenesis inhibitor, tissue inhibitor of metalloproteinase, is expressed (24) and endothelial cells undergo apoptosis, which begins prior to 1 year of age and is maximal at 2 years (28). In addition, the balance of angiogenic proteins shifts from a proangiogenic environment to an antiangiogenic milieu. Specifically, bFGF levels in the overlying epidermis decrease, whereas expression of the angiogenesis inhibitor, IFN-β, increases (27).
Clinical Characteristics
Hemangioma occurs in 4% to 10% of white infants and is rare in dark-skinned individuals (29). It is more common in premature infants (23% in premature infants less than 1,200 g) (30) and females (3:1 to 5:1) (31). Most are single (80%), but multiple hemangiomas are more likely to involve extracutaneous sites. Hemangiomas most commonly involve the head and neck (60%), followed by the trunk (25%), and extremities (15%) (22). Between 30% and 50% of lesions are visible at birth as either a small pale spot, telengiectatic stain, or ecchymotic area. However, the median age of appearance is 2 weeks after birth (31). Although Mendelian inheritance does not play a role in transmission (32), a potential locus on 5q has been located in three families with autosomal-dominant transmission (33).
Hemangiomas exhibit a unique growth pattern. During the 6- to 8-month proliferating phase, the lesion grows rapidly, faster than the growth of the child. If the tumor involves the superficial dermis it appears raised, firm, and reddish (Fig. 34-1). If the lesion occupies the deep dermis, the overlying skin may be minimally raised and bluish. By 10 to 12 months of age, hemangioma growth plateaus and increases in size at the same rate of growth as the child.
The involuting phase begins at approximately 1 year of age and is characterized by shrinking of the hemangioma. The bright color fades, the skin pales at the center of the lesion, and the tumor becomes less tense. Involution is completed in 50% of children by 5 years of age and 70% of children by age 7 (34). Improvement continues until 12 years of age. Size, site, depth and gender do not affect the rate of regression (23). The final involuted phase occurs after involution is complete. Fifty percent of patients have residually damaged skin, often with telangiectasias, yellowish discoloration, scarring, redundant skin, or skin laxity (31).
Occasionally, children have multiple cutaneous hemangiomas, termed disseminated hemangiomatosis. The lesions are usually less than 5 mm in diameter. Individuals with five or more cutaneous hemangiomas should be screened with ultrasonography or magnetic resonance imaging (MRI) to rule out lesions in internal organs (31). The liver is most commonly affected followed by the brain, gut, and lung.
Although hemangiomas usually affect the skin, isolated visceral lesions also occur. The liver is the most common site of extracutaneous hemangioma. Although most are small and discovered incidentally, children may present during the proliferating phase with congestive heart failure, hepatomegaly, and anemia (35). Differential diagnosis includes AVM and malignancy. Because both hemangioma and AVM are fast flow, they may be confused radiographically. However, 90% of fast-flow lesions of the liver are hemangioma (36). Hepatoblastoma does not illustrate the arteriovenous shunting characteristic of hemangioma.
Rarely, hemangiomas are associated with other anomalies. For example, cervicothoracic hemangiomas have been associated with sternal nonunion or supraumbilical raphe (37,38). Lumbosacral hemangiomas may signal an underlying spinal dysraphism. Consequently, children with hemangioma in this location should undergo ultrasonography for occult spinal dysraphism (39,40).
Differential Diagnosis
Deep hemangiomas may appear similar to a VM or LM, as a mass with overlying skin discoloration. However, the natural history of hemangioma usually differentiates it from these malformations. Rare variants of hemangioma include a macular lesion that can resemble CM and hemangiomas with persistent shunting like AVM (41).
Congenital hemangioma is completely developed at birth and does not illustrate the usual rapid postnatal growth (35). These small, well-localized lesions have a characteristic red-violet color and telengiectases, with central pallor. These tumors often regress rapidly after birth. Because these lesions are obvious at birth, they may be mistaken for a vascular malformation.
Pyogenic granuloma has been called lobular capillary hemangioma.These lesions usually affect the face, although they may occur anywhere on the skin. Unlike hemangioma, which begins proliferating at 2 weeks of age, the mean age of onset for pyogenic granuloma is 6.7 years of age (42). These lesions may arise from a CM and grow rapidly, forming a stalk. They are small, with an average diameter of 6.5 mm. They are complicated by crusting and bleeding (42). Treatment includes curettage, shave excision, laser therapy, or full-thickness excision (43).
Other congenital tumors that may be confused with hemangioma include tufted angioma (44), hemangiopericytoma (45), and fibrosarcoma (46). Although the natural history of hemangioma often differentiates it from other tumors and malformations, radiologic imaging is indicated if the diagnosis is in doubt. Biopsy is indicated if malignancy is suspected. An erythrocyte-type glucose transporter (GLUT 1) is specifically expressed in proliferating hemangiomas (47). Immunostaining against GLUT 1 can thus differentiate hemangioma from other vascular tumors such as pyogenic granuloma, hemangioendotheliomas, or angiosarcomas in cases when diagnosis cannot be made by history and physical exam.
Radiologic Characteristics
Radiographic studies can aid in the diagnosis in the 10% of patients whose diagnosis is still in question after the
history and physical exam. Ultrasonography is an easy and cost-effective way of differentiating hemangioma from slow-flow vascular anomalies. Hemangioma appears sonographically as a soft tissue mass with decreased arterial resistance and increased venous flow (48). Unlike VM or LM, a hemangioma will have increased blood flow. However, it is often difficult to sonographically differentiate a hemangioma from an AVM because both are fast-flow lesions. Radionucide scanning with technetium Tc 99m-tagged red blood cells may be used to locate multiple hemangiomas in the central nervous system or gastrointestinal tract (49).
history and physical exam. Ultrasonography is an easy and cost-effective way of differentiating hemangioma from slow-flow vascular anomalies. Hemangioma appears sonographically as a soft tissue mass with decreased arterial resistance and increased venous flow (48). Unlike VM or LM, a hemangioma will have increased blood flow. However, it is often difficult to sonographically differentiate a hemangioma from an AVM because both are fast-flow lesions. Radionucide scanning with technetium Tc 99m-tagged red blood cells may be used to locate multiple hemangiomas in the central nervous system or gastrointestinal tract (49).
MRI is the radiologic modality of choice for differentiating vascular anomalies. Children younger than 6 years of age generally require sedation for MRI (50). During the proliferating phase, hemangioma shows dilated feeding and draining vessels, appears solid and intermediately intense on T1-spin-echo images, and hyperintense on T2-weighted images. Fast flow and arteriovenous shunting are illustrated by flow voids (51). MRI of the involuting hemangioma demonstrates decreased blood flow and fewer blood vessels, with increased lobularity and fatty tissue. Involuted hemangioma appears as an avascular fatty mass on MRI (31).
Treatment
Observation
Observation is the mainstay of management because 90% of hemangiomas are small, localized, and do not involve aesthetically or functionally vital structures (31). Parents should be reassured by showing them photographs of the tumor in its proliferative, involuting, and involuted phases. Patients are followed closely through the proliferative phase of growth.
Treatment for Ulceration
Local wound treatment is indicated for the 5% of cutaneous hemangiomas that cause skin ulceration (52). Ulceration is more common on the lips, anogenital region, and parotid region (53). Local treatment includes application of hydrated petrolatum and viscous lidocaine, topical antibiotic, or hydrocolloid dressing. Eschars should be debrided and treated with wet-to-dry dressing changes.
Pharmacologic treatment can accelerate healing and may be considered in large ulcers or for small ulcers in sensitive locations. Flashlamp pulsed-dye laser may aid healing when two applications are given 4 to 6 weeks apart (54,55). Resection of the ulcerated tumor may be performed when the defect can be closed primarily, and the resulting scar would not be worse than if the lesion were removed during involution. Excision is most commonly performed for small, well-localized lesions on the scalp, chest, or extremity, but not for facial lesions (31).
Treatment for Endangering Complications
Ten percent of hemangiomas cause significant deformity or severe complications (56). Most complications occur with hemangiomas located in the head and neck. Sublottic hemangioma may obstruct the airway, whereas ulcerated lesions may destroy the eyelid, ear, nose, or lip. Periorbital hemangioma can block the visual axis or distort the cornea causing amblyopia. Gastrointestinal hemangioma may cause bleeding that requires transfusions.
Large hemangiomas, most commonly involving the liver, can cause high-output congestive heart failure (36) or hypothyroidism (57). Hypothyroidism results from the expression of a deiodinase by the hemangioma that cleaves iodine from thyroid hormone. Consequently, thyroid function should be evaluated in patients with a large hemangioma. Massive intravenous thyroid replacement may be necessary to avoid mental retardation until the hemangioma regresses.
Intralesional Corticosteroid
Corticosteroid is the first-line treatment of problematic hemangiomas. Corticosteroids can accelerate involution of hemangioma by their antiangiogenic mechanism. Corticosteroids inhibit macrophage production of the proangiogenic proteins VEGF and bFGF. In addition, corticosteroids inhibit phospholipase A2, the enzyme that releases arachidonic acid, the precursor to the proangiogenic prostaglandins (58). Finally, corticosteroids inhibit metalloproteinases, which facilitate angiogenesis by breaking down the extracellular matrix.
Intralesional corticosteroid is indicated for problematic lesions that are small, well-localized, and involve the nasal tip, cheek, lip, or eyelid. The response rate is 80% and is similar to the response from systemic corticosteroid therapy, and can minimize deformity and accelerate healing (59). Triamcinolone (25 mg per mL) is administered 3 to 5 mg per kg per injection. Injections are administered at 6- to 8-week intervals and may require up to five injections. Two cases of blindness have been reported with injection of periorbital hemangioma that may have been due to embolic occlusion of the retinal artery (60).
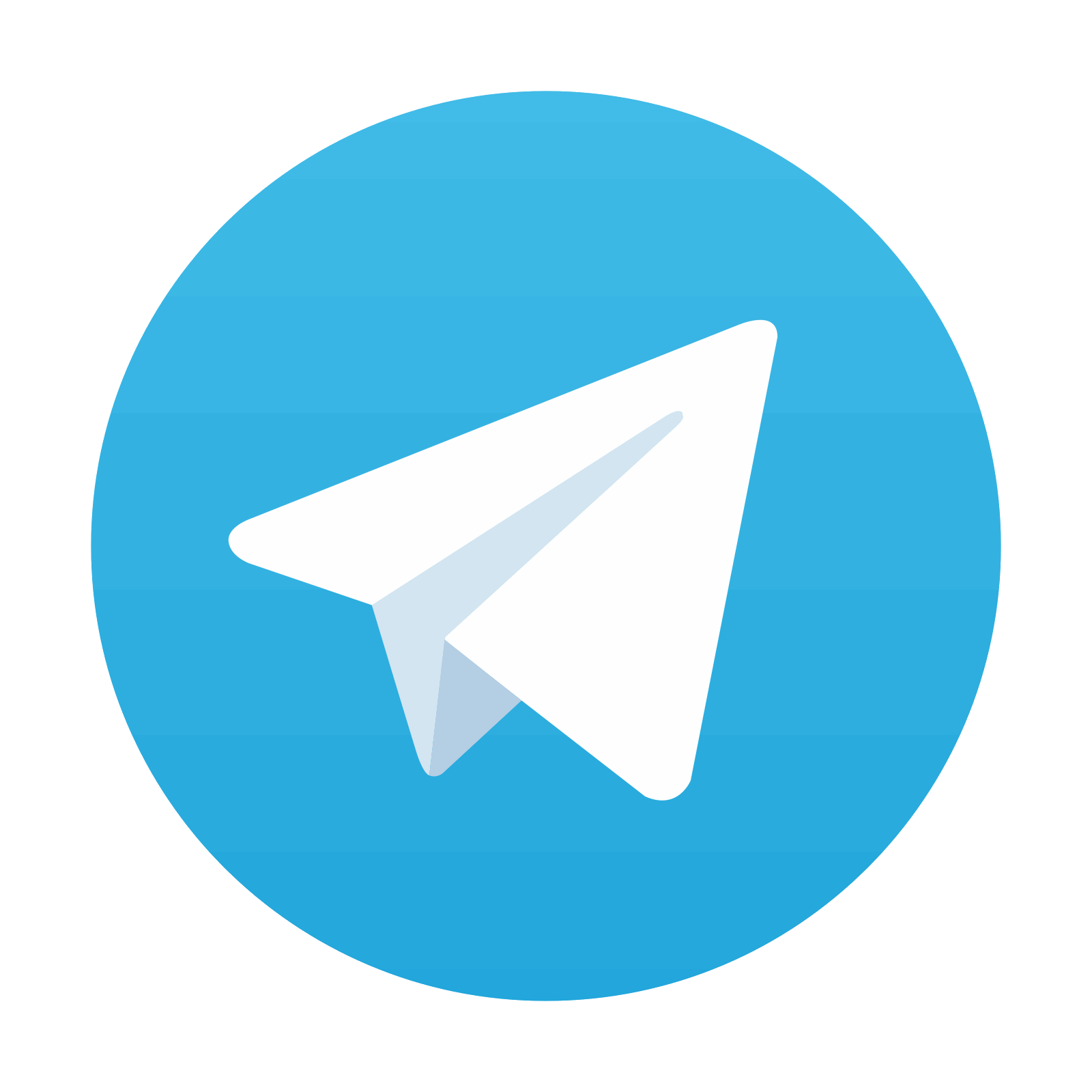
Stay updated, free articles. Join our Telegram channel

Full access? Get Clinical Tree
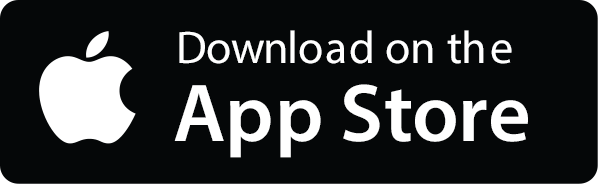
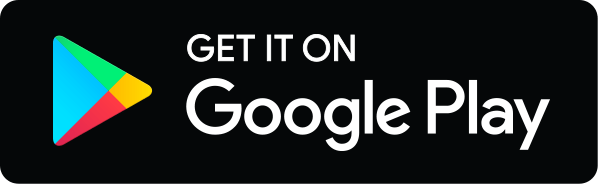
