Use of Metered Dose Inhalers, Spacers, Dry Powder Inhalers, and Nebulizers
Morton E. Salomon
Frank A. Illuzzi
Introduction
Respiratory diseases such as asthma, croup, and bronchopulmonary dysplasia (BPD) are among the most common childhood diseases and account for 10% to 15% of visits to the emergency department (ED) each year. Medications delivered by inhalation are being used with increasing frequency to treat respiratory disease, both acutely and on a maintenance basis. Aerosol therapy permits the delivery of small quantities of medication directly to the site of action (1,2,3).
Studies comparing the effectiveness of medications delivered via inhalation with that of oral or parenteral delivery have demonstrated that the inhalation route provides more rapid onset, equal duration of activity, and comparable improvement in pulmonary function testing, with fewer side effects (2,3,4,5). Because inhalation therapy can be administered without painful or invasive procedures, it also has the added benefit of eliciting less fear and resistance when a child visits the ED. Inhalation treatment should be viewed as a significant therapeutic advance and is now the mainstay of our treatment for many respiratory illnesses, particularly asthma.
Anatomy and Physiology
Lung Receptor Pathophysiology
The human respiratory system is under the control of the nervous system through autonomic nerve fibers originating in cranial nerve X. It is also controlled by multiple receptor sites with or without accompanying nerve fibers.
Autonomic influences on the lung are contributed by parasympathetic (cholinergic) forces and by sympathetic (adrenergic) receptors. The adrenergic system contains both α- and β-receptors.
Parasympathetic innervation is provided by fibers of the vagus nerve. Stimulation of the parasympathetic efferent fibers causes bronchoconstriction of the large and midsize airways, increased mucous gland secretion, and vascular dilatation. Postganglionic parasympathetic fibers terminate in bronchial smooth muscle, mucus-secreting goblet cells, and mast cells. These parasympathetic nerve fibers are present from the trachea to the bronchioles but are found in greatest density in midsize airways 3 to 8 mm in diameter. These same parasympathetic effects also can be induced by direct administration of cholinergic agents such as acetylcholine and methacholine (6).
The parasympathetic nervous system plays a role in airway response to irritants through what is referred to as the vagally mediated “cholinergic reflex.” When specific irritants activate receptors in the airways, these receptors initiate impulses via afferent fibers of the vagal system. The afferent vagal stimuli trigger reflexive responses via efferent fibers that release acetylcholine at multiple neuroeffector sites, leading to bronchoconstriction, mucus secretion, and mediator release from mast cells and cell membranes. This cholinergic reflex can be triggered by immunologic or nonimmunologic stimuli. The role of this parasympathetic reflex in chronic hyperreactive airway diseases, such as bronchitis and asthma, is not well defined and is likely to vary from one patient to another. Moreover, the cholinergic reflex is not the only mechanism by which irritants exert influence on the airways (6).
Sympathetic nerve fibers have not been found in the tracheobronchial tree of the human lung. Adrenergic influences on the lung are exerted at α- and β-receptor sites that receive no neuronal innervation. These adrenergic receptors are found in bronchial smooth muscle, blood vessels, and goblet cells and can be stimulated by either sympathomimetic endogenous agents (e.g., epinephrine) or exogenous agonists (e.g., terbutaline).
In contrast to parasympathetic receptors, adrenergic receptors are mostly concentrated in the smaller peripheral airways. Stimulation of β-receptors produces smooth muscle relaxation and subsequent bronchodilatation. β-agonists also are potent inhibitors of mast cell release and thus exert a strong anti-inflammatory effect. This anti-inflammatory control is most effective in the earliest phases of inflammation. Prolonged inflammation, with late phase reaction, is not as responsive to β-agonist stimulation. Glucocorticoids, in fact, exert some of their anti-inflammatory effect by restoring the availability and binding affinity of β-receptors (6).
At the intracellular level, cholinergic and adrenergic receptor stimulation is mediated by alterations in cyclic AMP and cyclic GMP. Increases in cyclic AMP (c-AMP) relax bronchial smooth muscle and inhibit mast cell degranulation. Increases in cyclic GMP (c-GMP) exert the opposite effect, contracting smooth muscle and stimulating mast cell release. Stimulation of parasympathetic effectors produces increases in intracellular c-GMP. Conversely, β-adrenergic stimulation increases c-AMP, whereas α-receptor stimulation decreases c-AMP (6). Thus, β-sympathomimetic activity produces bronchial relaxation, and both parasympathetic activity and α-sympathetic activity produce bronchoconstriction. Excessive parasympathetic tone, excessive α-sympathetic stimulation, or β-sympathetic blockade may be the predominant cause of hyper-reactive airways in different patients.
Two common categories of medication available by aerosol and used to treat common pulmonary illnesses are β-adrenergic agonists and parasympathetic antagonists. β2-agonists relax bronchial smooth muscle, producing bronchodilatation; inhibit inflammatory mediator release from mast cells; and stimulate mucociliary clearance. These effects are produced by activating adenyl cyclase with subsequent increased intracellular c-AMP.
Parasympatholytic agents include atropine and its derivatives. They are competitive inhibitors of acetylcholine at the neuroeffector junction and block the cholinergic reflex and parasympathetic receptor stimulation, thereby preventing a rise in intracellular c-GMP. The net effect, therefore, is to inhibit bronchoconstriction. Anticholinergic agents currently available for aerosolized treatment include atropine sulfate, which may lead to significant side effects when used in therapeutic respiratory doses, and ipratropium, a quaternary derivative of atropine that has a much wider therapeutic margin. Theoretically, α-adrenergic blocking agents should have a beneficial effect in the treatment of bronchoconstrictive respiratory diseases. By blocking α-stimulation and thereby inhibiting decreases in c-AMP, α-blockers should enhance bronchodilation. To date, however, α-receptor blockers have not been shown to have a convincing role in treating asthma or bronchitis. Their application is usually reserved for the patient with severe disease who is already being treated maximally with other agents (6).
Inflammation, not bronchospasm, is the basic pathologic condition encountered in chronic asthma. Airway inflammation results from injury to the lung possibly caused by trauma, inhalation of noxious or toxic or allergenic substances, respiratory infection, or even systemic infection. The most common chronic inflammatory disease of the lung is asthma.
Once triggered by injurious stimuli, the inflammatory process starts with the release of several chemical mediators from mast cells, leukocytes, and endothelial cell phospholipids. Chemical mediators in turn activate specific receptor sites on the cell wall surface of the lung. These receptors then decrease c-AMP concentration or increase calcium ion concentration inside the cell. The net result includes smooth muscle contraction, chemotaxis of inflammatory cells, microvascular leaks, increased mucus secretion, and parasympathetic neuronal reflex stimulation. The immediate response to fast-acting inflammatory mediators, such as histamine, is primarily bronchoconstriction. This reaction often responds to bronchodilators. If the inflammatory response continues and the slower-acting metabolites of arachidonic acid accumulate, mucosal swelling, mucus secretion, and desquamation of cells occur. This produces a late-phase inflammatory response that is less sensitive to bronchodilator therapy (6).
Corticosteroids exert an anti-inflammatory response principally by stabilizing mast cells. They also restore the availability and sensitivity of β-agonist receptors. The glucocorticoids currently used for aerosol therapy are synthetic analogs of hydrocortisone. When compared with cortisol or dexamethasone, they have the distinct advantage of having less systemic absorption, rapid inactivation when reaching the central circulation, high local potency in the lung, and less systemic potency. The aerosolized glucocorticoids currently in use in the United States via metered dose inhalers (MDIs) include beclomethasone, triamcinolone, and flunisolide. The glucocorticoids commonly available via dry powder inhalers (DPIs) include fluticasone and budesonide.
Aerosols
An aerosol is a suspension of either solid particles or liquid droplets in a stream of air. By incorporating medication in these droplets, aerosols can deliver therapy directly to the lung. Large droplets are often trapped and filtered by the upper airway. To be effective in treating respiratory illnesses, the droplets must reach their receptor sites in the smaller airways. Drug delivery to the peripheral lung depends on three factors: aerosol droplet size, inspiratory flow, and disease state in the lung (1,2).
Droplets created by jet nebulizers and MDIs vary in size. Droplets greater than 8 μm are always deposited in the mouth and nasopharynx by inertial impaction. β-Agonists are not well absorbed by this mucosa and therefore will not exert any demonstrable effects (1).
To reach the distal airways, droplets must be less than 5 μm in size. Once they reach the bronchiole, they settle out by gravitational sedimentation. However, very small droplets (less than 1 μm) generally remain suspended in the airstream and are exhaled without deposition on the tissue surface (1).
Regardless of droplet size, particles are unlikely to get beyond the oropharynx if significant turbulence occurs in the airstream. Turbulence can be created by rapid inspiratory flow rates, partial mechanical obstructions, or sudden changes in airstream direction. Inspiratory flow rates through the patient’s airways greater than 1.0 L/sec will create turbulent airflow. Under these conditions, inertial forces keep droplets from remaining suspended in the mainstream, and droplets of any size will deposit on the nasal and oropharyngeal airways. At slower inspiratory rates—generally less than 0.5 L/sec—laminar airflow is evident, and the droplets may reach the peripheral lung. Slow flow rates of less than 0.5 L/sec are best achieved by inhalations of 6-second duration. The airstream slows even further on reaching the smaller airways, allowing the droplets to deposit by gravitational sedimentation. Sedimentation at peripheral sites can be further enhanced by breath holding at the end of inspiration, which postpones the reversal of airflow (1,2).
Airflow through diseased airways, narrowed by spasm, edema, or mucus, is much more turbulent than through healthy airways. More drug is deposited before reaching the targeted airways. This may lead to the patient with acute respiratory illness requiring larger doses of aerosolized medication to obtain the same therapeutic effect.
Indications
Aerosol therapy is most commonly applied in pediatric emergency settings to the treatment of asthma, bronchiolitis, bronchopulmonary dysplasia (BPD), and laryngotracheobronchitis (LTB).
Asthma is the most common chronic disease of childhood, affecting up to 15% of the pediatric population in the United States. It is characterized by hyper-responsive airways that are prone to develop edema, mucus hypersecretion, and muscular constriction in response to offending stimuli. Triggers most commonly associated with airway inflammation in asthmatics include inhaled irritants such as cigarette smoke, changes in weather, exposure to cold air, exercise, environmental allergens, and minor respiratory illnesses such as sinusitis and otitis. Patients with asthma generally manifest their airway hyperactivity with symptoms such as cough and wheezing. In more severe cases, patients also will have shortness of breath, tachypnea, retractions, diminished peak expiratory flow rates, and hypoxia. Aerosolized medications are currently the mainstay of bronchodilator therapy for asthma. Corticosteroids and sodium cromolyn are anti-inflammatory agents commonly used in children to reduce the inflammatory aspects of exacerbations. Inhaled β-agonists, such as albuterol, are used as both prophylactic therapy and acute therapy. The role of inhaled anticholinergic agents in the treatment of acute childhood asthma is not as well substantiated, although these agents are known to have measurable bronchodilator effect. Atropine sulfate is available only as a nebulizer solution. Ipratropium bromide, an anticholinergic agent with much fewer side effects than atropine, is available in the United States in a metered dose inhaler and as a nebulizer solution (7). Inhaled ipratropium added to albuterol demonstrates added benefit in children with acute severe asthma and leads to fewer admissions (8).
Bronchiolitis is a pulmonary infection generally occurring in late fall and winter that mostly affects young infants. Its peak incidence occurs between the age of 2 and 8 months. Bronchiolitis is characterized by coryza and cough progressing to wheezing, prolonged expiration, and respiratory distress. Most cases of bronchiolitis are caused by respiratory syncytial virus (RSV), but other viruses such as parainfluenza and influenza can be isolated from patients with this illness. Mild bronchiolitis has been treated with nebulized bronchodilators, such as albuterol, and improved symptoms and signs are found in subgroups of patients. Corticosteroids are not clearly beneficial but are under investigation. In some children, however, it is difficult to differentiate acute asthma from bronchiolitis, because the symptoms are so similar. Aerosolized ribavirin can be effective in ameliorating the course of severe bronchiolitis caused by RSV when administered continuously over a 3- to 5-day period in high-risk patients (9,10). This antiviral agent is not commonly useful and is generally reserved for patients with severe respiratory compromise or an underlying illness such as congenital heart disease or BPD (7,10).
Bronchopulmonary dysplasia (BPD) is a chronic pulmonary disorder of infancy that results from treatment by mechanical ventilation of hyaline membrane disease or other congenital lung disorders. It generally occurs in newborns undergoing prolonged ventilation during the first several weeks of life. Approximately 15% of premature infants develop BPD. Its etiology has been ascribed to many factors, including high concentrations of oxygen and barotrauma from positive pressure ventilation. Infants with BPD often have chronic tachypnea, wheezing, and asymmetric breath sounds. In more severe cases, arterial blood gases show chronic hypercarbia and hypoxia. Chest radiographs of BPD patients usually demonstrate hyperinflation and interstitial nodularity or multiple cystic areas. Home management of patients with BPD and treatment of acute exacerbations of respiratory difficulties can be similar to the treatment of asthma. These patients are frequently improved with daily nebulized β-agonists and occasionally by aerosolized corticosteroids. Acute exacerbations are treated by intensified β-agonist treatment, systemic steroids, and other therapies as necessary (7).
Laryngotracheobronchitis (LTB), or croup, is a viral infection of the upper airways affecting the larynx, trachea, and bronchi. Sixty percent of cases appear to be caused by parainfluenza virus, mostly in early fall. Influenza virus, adenovirus, and RSV also have been recovered from croup patients. LTB is characterized by mild to moderate fever, barking cough, and inspiratory stridor. Croup is the most common cause of stridor in childhood. Milder cases can be treated simply with normal saline mist. Moderate to severe bouts of LTB receive treatment with either racemic or L-epinephrine in a nebulized format. Studies have demonstrated a role for nebulized or systemic corticosteroids as well (11).
TABLE 78.1 Available Formats | ||||||||||||||||||||||||||||||||||||||||
---|---|---|---|---|---|---|---|---|---|---|---|---|---|---|---|---|---|---|---|---|---|---|---|---|---|---|---|---|---|---|---|---|---|---|---|---|---|---|---|---|
|
In patients with cystic fibrosis, aminoglycosides can be aerosolized to suppress Pseudomonas aeruginosa and other organisms. Aerosolized pentamidine is indicated for prophylaxis against pneumocystis carinii in patients over the age of 5 years who have AIDS and are unable to tolerate trimethoprim-sulfa (7).
Table 78.1 summarizes the medications available in the MDI format, in nebulized solution, or in both. A large variety of adrenergic medications are available by MDI, including nonselective β1– and β2-agonists such as epinephrine, isoproterenol, and metaproterenol and β2-selective agents such as terbutaline, pirbuterol, and albuterol. Corticosteroids are currently only available in MDIs or DPIs for inhalation therapy. These preparations have been difficult to aerosolize when incorporated into nebulizer solutions.
All adrenergic drugs available in MDIs are also available for nebulization. Ipratropium and sodium cromolyn are similarly available in both MDI and nebulizer formats. Drugs only available in nebulizer format include atropine, ribavirin (which requires a special particle generator), pentamidine, racemic (L- and R-) epinephrine or L-epinephrine, and nystatin suspension (for laryngeal candidiasis) (1,2,3). Cromolyn powder also can be delivered using a spinhaler.
Spacers are small canisters that attach to MDIs to allow patients who cannot easily hold their breath to inhale their medication more effectively. Spacers are indicated for use with MDIs in patients who are likely to have difficulty correctly coordinating the MDI inhalation, such as younger patients (generally less than 10 years of age), neurologically impaired patients, patients with arthritis of the hands, and adults who are unable to master the MDI technique. Spacers also are helpful for patients of all ages with significant acute distress, who are more likely to have difficulty taking slow deep breaths and holding it at the end of inspiration. This may include anyone with acute respiratory distress. Indications for nebulizer use are similar to those for spacer need. Nebulizers are indicated for younger patients, patients with coordination problems, and patients with moderate to severe asthma or distress. Patients who are maintained chronically on β-agonist therapy by MDI or nebulizer at home often require higher and more frequent doses during acute exacerbations of bronchospasm. Home use should not exceed a frequency of less than every 3 hours without physician contact. Failure to seek emergent care in a timely manner in patients on bronchodilators without anti-inflammatory therapy is an important contributor to asthma mortality. In the monitored setting, such as the hospital, MDIs can be used with significantly more intensity. One hospital regimen that has been suggested is four puffs over 2 minutes followed by one puff every minute until dyspnea is relieved or the patient develops a significant tremor (1). It is key to augment β-agonists with anti-inflammatory therapy early in the treatment course. Continuous nebulization therapy (CNT) should be applied only to patients with severe asthma, and its use restricted to the ED or a monitored inpatient setting. Indications for CNT might include patients whose chests are too tight to perform peak flow testing, patients with PaCO2 greater than 40, and patients with asthma scores in the severe range. CNT is also indicated for hospitalized patients who repeatedly deteriorate clinically between β-agonist treatments performed every 1 to 2 hours (12).
Equipment: Technical Considerations
Metered Dose Inhalers
A metered dose inhaler is an inhalation device that contains medication dissolved or suspended in a pressurized propellant solution. The MDI has two components—the drug canister and the inhalation device (Fig. 78.1). Within the canister, the therapeutic medication is mixed with the propellant at high pressure to maintain a liquid phase. The inhalation device consists of a hollow canister holder, an actuator, a valve, and a mouthpiece with cover. The actuator, when depressed, opens the valve. The valve is designed to release a precise, premeasured amount of aerosol with each actuation.
Sudden decompression of the liquid in the canister produces an aerosol of propellant plus medication. As the liquid exits the mouthpiece, heterodisperse (various size) particles average 35 μm in diameter. These large droplets travel away from the mouthpiece at a high velocity, and the propellant evaporates within a few centimeters, leaving smaller droplets consisting primarily of medication.
When the drug is released from the MDI, approximately 5% to 10% is deposited on the inhaler device or escapes into the air, and another 80% is deposited in the oropharynx and eventually swallowed. This means that only 10% to 15% of the drug released actually reaches the lung, even under ideal conditions. It is likely that only 3% of the total dose reaches the bronchioles and alveoli. The proportion of drug reaching the lung is greatly influenced by the patient’s technique. Factors such as the position of the inhaler at the mouth, slow inspiratory flow rate, adequacy of breath holding, adequate interval between puffs of at least 1 minute, and volume of air inhaled can all influence the delivery of medication to the peripheral lung sites (2).
Most package inserts instruct the patient to close the lips around the mouthpiece of the inhaler. However, the open mouth technique can deliver up to twice as much medication to the lower lung. By holding the inhaler 1 to 2 inches (two adult fingerbreadths) away from the mouth, less medication is deposited on the oropharynx. Studies have demonstrated that the open mouth technique produces less oropharyngeal deposition, more peripheral lung delivery, and better pulmonary function response (2). A variation on the open mouth technique has the patient hold the MDI between opened lips, which reduces the problems of improper aiming and provides the same pharmacologic benefits (2).
As mentioned, slow inspiratory flow rates create more laminar flow and deliver more drug to the distal airways. Ideally the patient should inhale the released medication for a minimum of 6 seconds (2). Breath holding at the end of inhalation allows more time for the inhaled medication to sediment on the lung surface. Breath holding for up to 10 seconds has been shown to incrementally increase drug absorption. Beyond 10 seconds, breath holding does not add benefit (2). If patients are unable to hold their breath for 10 seconds, they should do so as long as is comfortably possible. Larger, deeper breaths bring a larger tidal volume into the lung and more medication with it. Optimal dosing can be achieved by beginning with a full exhalation to functional residual capacity, then inhaling. Such maneuvers are unnecessary, however, are likely to cause additional turbulence or coughing, and are difficult for acutely ill patients (1).
Theoretically, waiting several minutes between puffs of bronchodilator aerosol should enhance delivery of drug. The minimum time recommended is 1 minute. Allowing time for the first puff to exert some therapeutic effect should open the airway to deeper penetration by subsequent bursts. Moreover, if the patient is to receive both bronchodilator therapy and anti-inflammatory therapy, it would be logical to suppose that bronchodilator therapy should precede anti-inflammatory therapy. This would allow the anti-inflammatory to penetrate deeper after the bronchodilator exerts its benefit.
Spacer Devices
Spacer devices are holding systems or reservoirs that collect the aerosol from an MDI, momentarily, before inhalation. The spacer holding chamber varies in volume but can be as large as 750 mL. The spacer has an inlet for the inhaler at one end and a mouthpiece at the other. Within the mouthpiece, a one-way valve allows aerosol out of the chamber but blocks return of exhaled breath. A whistle or alarm sounds when the patient inhales too rapidly (usually greater than 0.3 L/sec). Each brand of spacer has some combination of these features but not all. Moreover, spacers are not interchangeable on all commercially available inhalers.
Once the medication is discharged from the MDI into the chamber, the patient takes one or two slow breaths or several normal tidal breaths through the mouthpiece. A spacer device offers several advantages over the MDI without a spacer attached. The chamber slows down the propellant, giving the droplets time to evaporate. These smaller, slower droplets are less likely to deposit on the oropharyngeal mucosa (2). The spacer removes the need to coordinate inhaler activation with inspiration. This is especially useful in children, patients with neurologic impairment or arthritis, and patients having difficulty with correct MDI technique (1,2,3,13).
Spacer devices have allowed children as young as 2 or 3 years of age with stable asthma to use MDIs effectively (13). When fitted with a face mask attached to the mouthpiece, spacers can even be used with infants as young as 4 months (12,14). However, the mask deposits aerosol on the face and increases nasal filtration, thereby reducing lung delivery to some extent.
Because they slow the propellant down, evaporate the particles, and reduce oropharyngeal deposition of the medication, it is expected that spacers increase the dose of medication reaching the lung. However, it has not been proven that the amount of medication reaching the alveoli is increased. In terms of clinical benefits, studies have produced mixed results when comparing the bronchodilating effects of MDIs with spacers against MDIs used alone. Currently, it appears that spacer devices do not add any measurable clinical benefit when the MDI without spacer is used properly (1). Even results in children are inconclusive. However, it is safe to say that in both children and adults there is no drawback to the use of spacers, and they are likely to produce equivalent or better lung function with generally greater convenience (13).
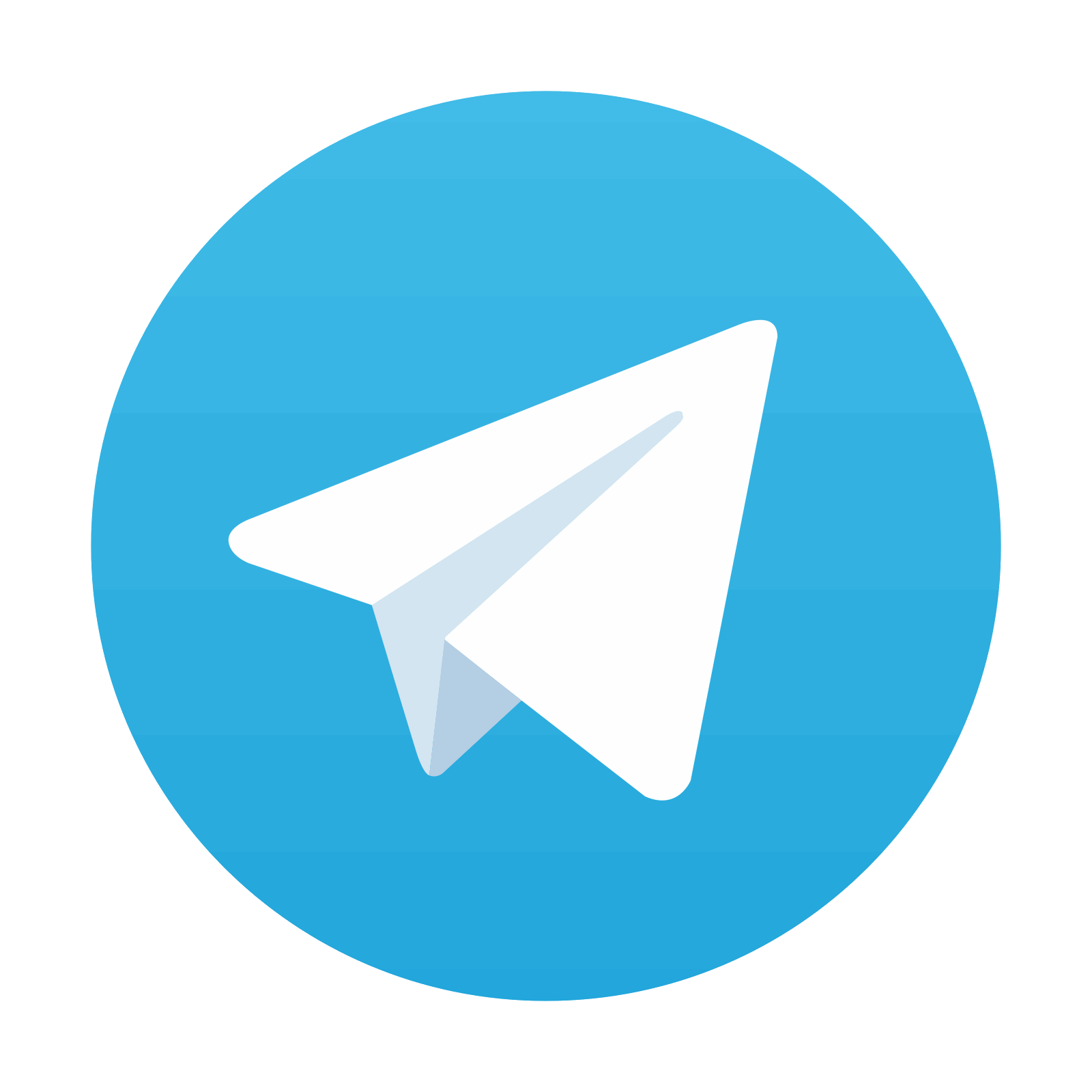
Stay updated, free articles. Join our Telegram channel

Full access? Get Clinical Tree
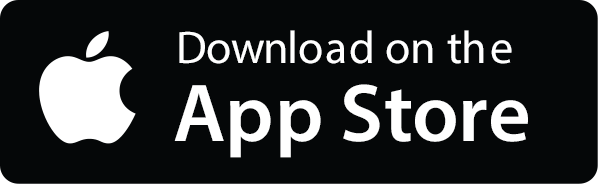
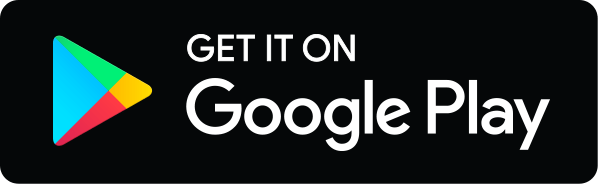