Thoracostomy and Related Procedures
Steven Baldwin
Thomas E. Terndrup
Section A: Thoracostomy Procedures
Introduction
This chapter is divided into two sections. The first section describes the issues and procedural details relevant to needle, catheter and tube thoracostomies. The second section describes open (sucking) chest wounds.
Hippocrates was the first to describe entering the pleural space with a metal tube to drain “bad humors” (1). Playfair, in 1875, and Hewitt, in 1876, described closed chest tube drainage with a tube and an underwater seal (2,3). The technique of tube thoracostomy, however, was not widely used until 1917 when it became useful in treating post influenza empyema (4). During World War II, tube thoracostomy gained widespread use for the treatment of traumatic hemopneumothorax and empyema. It is now commonly used to treat many types of pleural collections.
Various types of thoracostomy procedures have been described. These include needle thoracostomy, catheter thoracostomy (Seldinger technique or catheter over needle technique), and tube thoracostomy. In most cases, each can be performed at the bedside. Other variations exist but are not relevant to the emergency physician and will not be discussed.
Thoracostomy procedures are primarily used to treat a few specific clinical problems that involve the pleural space(s). Some of these problems are life-threatening and require immediate intervention, but most can be successfully stabilized or even definitively treated by performing a thoracostomy procedure. Other problems are less emergent and able to be managed by a thoracostomy procedure performed urgently or semi-electively. The ability to perform thoracostomy procedures emergently is a critical resuscitative skill for all physicians who provide emergency care.
Emergent thoracostomy procedures are rarely needed in children. They are most commonly needed to treat pneumothoraces complicating mechanical ventilation of preterm and term neonates. In most emergency department settings, the majority of emergent thoracostomy procedures are performed for conditions resulting from blunt thoracic trauma, but they may also be required to manage complications related to positive pressure mechanical ventilation, vascular access, or other procedures and to manage problems related to inadequacy, failure, or dislodgement of an existing thoracostomy drainage device. Thoracostomy procedures performed for other, nonsurgical indications are rare and most commonly performed for various infectious or inflammatory conditions causing fluid collections in the pleural space, for spontaneous pneumothoraces, and for other very rare reasons that are not pertinent to emergency care.
Anatomy and Physiology
Proper performance of thoracostomy procedures requires an appropriate understanding of thoracic anatomy and respiratory physiology (5,6,7,8,9). This knowledge allows for the early identification of potential thoracic pathology and the determination of the need for intervention. After a thoracostomy procedure is ordered, the operator must be able to apply additional specific anatomic and physiologic knowledge to optimally perform the procedure and to appropriately manage the patient afterward. In particular, the operator needs sufficient understanding of relevant anatomy and physiology to properly select an appropriate operative site, adapt the procedure to the patient’s condition, manage the expected clinical consequences of the procedure, and recognize significant complications related to the procedure.
The thorax consists of the thoracic wall and its associated internal contents. The thoracic wall is formed by the sternum,
the ribs and their associated cartilages, the spine, the musculature attached to the skeletal elements, and the covering skin. The ribs articulate with the thoracic vertebrae to allow the excursion necessary for breathing movements. This respiratory pump action is also partially subsumed by the action of the diaphragm.
the ribs and their associated cartilages, the spine, the musculature attached to the skeletal elements, and the covering skin. The ribs articulate with the thoracic vertebrae to allow the excursion necessary for breathing movements. This respiratory pump action is also partially subsumed by the action of the diaphragm.
The thoracic cavity is delimited by the chest wall and the diaphragm. There are several important structures contained within this area: the lower trachea, bronchi, lungs, heart and pericardium, great vessels, esophagus, phrenic nerves, thoracic duct, and several lesser structures.
A serous pleural membrane covers the inner surface of the chest wall; the outer surface of the lungs, including the lobar fissures; the thoracic surface of the portions of the diaphragm in direct contact with the lungs; and the lateral aspects of the mediastinum. This arrangement creates a pleural space in each hemithorax. Each pleural space is normally only a potential space containing a trivial amount of serous fluid. Introduction of a significant volume of air, fluid, or blood will cause the pleural space to expand and occupy a portion of the intrathoracic volume with a consequent impact on respiratory function.
In addition to understanding thoracic anatomy well enough to recognize when thoracostomy might be indicated, operators must also understand several additional anatomic relationships that are relevant to the performance of the procedure. Understanding these issues is especially important to the proper placement of thoracostomy drainage devices and to the avoidance of several serious procedure-related complications.
Bedside thoracostomy procedures invariably must traverse the soft tissues of an intercostal space to reach the pleural space. Understanding the anatomy of the intercostal space is important to reduce the risk of nerve or vascular injury. Each intercostal space is bounded by ribs superiorly and inferiorly. A neurovascular bundle resides along the inferior border of each rib. Therefore, instrumentation should occur only along the superior rib margin and dissection or procedure related trauma near the inferior border should be avoided. Inadvertent injury to the neurovascular bundle can result in the loss of intercostal nerve function or serious intercostal artery bleeding. Bleeding from an intercostal artery can be very difficult to control because there is often no easy way to apply direct pressure to the vessel. In some instances, iatrogenic injury to an intercostal artery may necessitate operative intervention to control the bleeding. The risk of neurovascular bundle injury in small babies is significantly greater than it is for larger children, adolescents, and adults because the intercostal space is much narrower.
In addition to understanding the anatomy of the intercostal space, the operator must also be able to correlate external thoracic landmarks with the position of certain internal structures (Fig. 29.1). This knowledge is important to assure proper placement of drainage devices and to avoid injury
to internal organs or structures. Perhaps the most critical issue is the location of the diaphragm, which normally extends significantly above the bottom of the rib cage. A significant portion of the upper abdomen is enclosed by the lower ribs. Hence, care must be taken during the procedure to ensure that the device is placed within the pleural space and not through the diaphragm into the abdomen. Entry to the abdomen has serious risks and a life-threatening injury to the liver, spleen, stomach, intestine, and other abdominal structures is possible. Patient position can affect the location of the diaphragm. Patient positions (e.g., fetal position) or conditions (e.g., pregnancy) that increase intra-abdominal pressure will tend to push the diaphragm higher into the thoracic cavity. Positions that place the abdomen at or above the level of the thorax (e.g., Trendelenburg position) also can displace the diaphragm cephalad due to abdominal contents pushing up against the diaphragm. Patient positions that reduce abdominal pressure and/or put the abdomen below the level of the thorax (e.g., sitting, semi-upright, or upright positions) will tend to lower the level of the diaphragm.
to internal organs or structures. Perhaps the most critical issue is the location of the diaphragm, which normally extends significantly above the bottom of the rib cage. A significant portion of the upper abdomen is enclosed by the lower ribs. Hence, care must be taken during the procedure to ensure that the device is placed within the pleural space and not through the diaphragm into the abdomen. Entry to the abdomen has serious risks and a life-threatening injury to the liver, spleen, stomach, intestine, and other abdominal structures is possible. Patient position can affect the location of the diaphragm. Patient positions (e.g., fetal position) or conditions (e.g., pregnancy) that increase intra-abdominal pressure will tend to push the diaphragm higher into the thoracic cavity. Positions that place the abdomen at or above the level of the thorax (e.g., Trendelenburg position) also can displace the diaphragm cephalad due to abdominal contents pushing up against the diaphragm. Patient positions that reduce abdominal pressure and/or put the abdomen below the level of the thorax (e.g., sitting, semi-upright, or upright positions) will tend to lower the level of the diaphragm.
The level of the diaphragm is also affected by the phases of breathing. It is usually highest at end-expiration and lowest at end-inspiration. At forced end-expiration, the diaphragm may elevate as high as the fourth interspace in some patients. If the patient is able to cooperate and control his breathing according to the operator’s commands, then he may be able to help the operator by holding his breath at end-inspiration as the operator enters the pleural space. The operator should also consider the possibility that the diaphragm may lie in an abnormal position when the patient has abdominal distention, increased abdominal pressure, paralysis of a portion of the diaphragm, severe scoliosis or other major chest wall deformities, or other suggestive findings. Rarely, diaphragmatic hernias, either congenital or traumatic, may allow unintended entry into the abdominal cavity during a thoracostomy procedure. Even worse, a diaphragmatic hernia may allow abdominal contents to enter the thorax placing them at risk for injury.
The operator must also understand the normal relative position of the mediastinum with respect to external thoracic landmarks in order to avoid entering or injuring the mediastinum. The mediastinum does not normally communicate with the pleural space of either hemithorax directly. Therefore, thoracostomy drainage devices that are unintentionally placed in the mediastinum are likely to be ineffectual for pleural drainage. Additionally, mediastinal structures, especially the heart, are put at risk for injury. The mediastinum normally occupies much of the central portion of the thoracic cavity. It extends laterally further into the left hemithorax because of the leftward projection of the heart. It contains the thymus, heart and pericardium, portions of the great vessels, trachea and esophagus. The pleurae reflect and cover both sides of the mediastinum laterally. Perforation of the lateral membrane of the mediastinum may allow communication with the pleural space on the affected side. The pleural space on each side of the chest extends close to the midline along the undersurface of the anterior chest wall and in front of the heart and pericardium. Prior to performing a thoracostomy procedure, the clinician should try to anticipate conditions that may alter the size or location of the mediastinum. In particular, history, examination, imaging, or other findings suggesting extreme cardiomegaly, abnormal situs, or other issues should be recognized. The heart wall may be nearly or even immediately adjacent to the lateral chest wall in patients with marked cardiomegaly. Unintended placement of a drainage device in a heart chamber is a catastrophic event. Rarely, patients with mediastinal tumors will require thoracostomy procedures. Such tumors can significantly increase the size of the mediastinum and distort its position. The greatest concern regarding massive mediastinal tumors is, however, their tendency to compromise the patient’s airway. Therefore, careful attention should be paid to sedation, analgesia, patient positioning, and contingency planning in the event of deterioration or complications when performing a thoracostomy procedure.
For cosmetic reasons, breast tissue should be avoided during thoracostomy procedures. It is particularly important to avoid the breast bud tissue in prepubertal females to avoid any risk of abnormal breast development during puberty. The operator should leave a significant distance between any palpable breast tissue and the operative site. If there is no palpable breast tissue, the operator should choose an operative site that is at least several centimeters away from the outer edge of the areola. Subcutaneous dissection should assiduously avoid the breast bud and areolar areas as well.
In rare cases, the clinician may need to perform a thoracostomy procedure for a patient with another medical device in place near the intended operative site. Examples of medical devices in the thoracic area that may impact the selection of an operative site include indwelling central venous catheter ports and tubing, pacemakers or implanted defibrillators and their associated leads, CSF shunt tubing, implanted infusion pumps, and vagal nerve stimulators with their associated leads. The operator may also encounter patients with postsurgical drains, transthoracic pacing wires, monitoring catheters, and other devices. The clinician performing a thoracostomy should be aware of these devices and avoid them, if possible. Coordination with consultants should be undertaken when necessary or appropriate.
Sometimes, a patient will require a thoracostomy procedure prior to another surgical procedure involving the chest. If it is anticipated or known that a patient will soon undergo placement of a medical device and/or a major surgical procedure affecting the thorax, then the clinician performing the thoracostomy procedure should avoid, whenever possible, the areas of the chest that will be used for the future surgical procedure(s). Such consideration may facilitate the upcoming surgery and reduce the risk of infection or other complications. When possible, care should be coordinated with the surgical team.
Prior surgery, infectious or inflammatory processes, neoplastic diseases, or other conditions involving the pleural
space sometimes can impact the performance of a thoracostomy procedure. This is primarily due to pleural adhesions and scars. If pleural adhesions or scars are disrupted, significant bleeding may arise from associated blood vessels. When bleeding is persistent or severe, operative intervention may be required. Breaking apart pleural adhesions may also risk lung injury or disruption of prior surgical sites with resultant bleeding, air leaks, or other consequences. Attempting to pass thoracostomy drainage devices across thick pleural adhesions, such as those that may arise at an old thoracotomy incision site, can be particularly risky. Performing a thoracostomy procedure at a site that might contain major pleural adhesions also increases the risk that the drainage device will not freely communicate with all parts of the pleural space and may not function properly. Imaging studies often help to identify a procedure site that will allow effective drainage of intrapleural air or fluid but avoids the prior surgical site.
space sometimes can impact the performance of a thoracostomy procedure. This is primarily due to pleural adhesions and scars. If pleural adhesions or scars are disrupted, significant bleeding may arise from associated blood vessels. When bleeding is persistent or severe, operative intervention may be required. Breaking apart pleural adhesions may also risk lung injury or disruption of prior surgical sites with resultant bleeding, air leaks, or other consequences. Attempting to pass thoracostomy drainage devices across thick pleural adhesions, such as those that may arise at an old thoracotomy incision site, can be particularly risky. Performing a thoracostomy procedure at a site that might contain major pleural adhesions also increases the risk that the drainage device will not freely communicate with all parts of the pleural space and may not function properly. Imaging studies often help to identify a procedure site that will allow effective drainage of intrapleural air or fluid but avoids the prior surgical site.
The ability to appropriately select a surgical site and perform the steps of a thoracostomy procedure is largely related to an understanding of the pertinent anatomy. However, the ability to determine that an individual patient actually requires a thoracostomy procedure, that a thoracostomy procedure needs to be adapted to meet some specific patient need, or that a complication has occurred is usually related to an understanding of physiology.
Thoracostomy procedures are primarily designed to ameliorate various conditions that cause physiologic derangements involving the thorax or thoracic structures. The most common conditions that can be corrected by thoracostomy procedures are those caused by abnormal accumulations of air and/or fluid in the pleural space (Fig. 29.2).
An abnormal air collection in the pleural space is termed a pneumothorax. Pneumothoraces can be caused by a traumatic injury to the lung or bronchi or from wounds that fully traverse the chest wall. Pneumothoraces can also result from positive pressure applied to the lungs during assisted ventilation, a forceful Valsalva maneuver, or scuba diving. Lung blebs can predispose the patient to the development of pneumothoraces. Iatrogenically induced pneumothoraces can complicate catheterization of subclavian or jugular vessels, and other procedures. In rare cases, a pneumomediastinum or a pneumopericardium will extend to create a concomitant pneumothorax. Air in the pleural space can also rarely result from free air tracking into the pleural space from the abdomen or from an esophageal rupture or injury.
There are several types of fluid that may collect in the pleural space. Blood in the pleural space is termed a “hemothorax” and most commonly results from blunt or penetrating thoracic trauma. Less common causes of bleeding into the pleural space include: bleeding due to iatrogenic mishaps or complications related to catheterization of a subclavian, jugular, or other vessel; bleeding related to other types of surgical procedures (including thoracostomies) in or around the chest area; bleeding caused by erosion of blood vessels related to an adjacent disease process; and bleeding associated with other rare conditions. In very rare cases, transfused blood can enter the pleural space via a malpositioned or eroded vascular catheter. Coagulopathy greatly increases the risk of a significant hemothorax.
![]() Figure 29.2 Types of intrapleural air and/or fluid collections. A. Simple pneumothorax. B. Tension pneumothorax. C. Hemothorax or hydrothorax. D. Hemopneumothorax. |
Other than blood, various fluids can also collect in the pleural space and produce a pleural effusion (hydrothorax). The Starling equation explains many of the possible pathophysiologic mechanisms that can promote the accumulation of fluid in the pleural space. (The Starling equation indicates
that transmembrane fluid flux gradients are related to the net difference between a hydrostatic pressure term which is calculated as a permeability coefficient times the net hydrostatic pressure across the membrane and an oncotic pressure term, which is calculated as a selectivity/activity coefficient times the net oncotic pressure across the membrane.) Net accumulation of pleural fluid occurs whenever the factors in the Starling equation promote pleural fluid production faster than pleural lymphatic function can remove it. Therefore, primary pleural fluid collections can arise due to changes in net hydrostatic pressure and/or net oncotic pressure favoring fluid transudation into the pleural space, changes in endothelial permeability or integrity (selectivity) favoring fluid exudation into the pleural space, or decreased lymphatic function causing impaired ability to reabsorb fluid from the pleural space or other extravascular compartments (see also Fig. 81.1).
that transmembrane fluid flux gradients are related to the net difference between a hydrostatic pressure term which is calculated as a permeability coefficient times the net hydrostatic pressure across the membrane and an oncotic pressure term, which is calculated as a selectivity/activity coefficient times the net oncotic pressure across the membrane.) Net accumulation of pleural fluid occurs whenever the factors in the Starling equation promote pleural fluid production faster than pleural lymphatic function can remove it. Therefore, primary pleural fluid collections can arise due to changes in net hydrostatic pressure and/or net oncotic pressure favoring fluid transudation into the pleural space, changes in endothelial permeability or integrity (selectivity) favoring fluid exudation into the pleural space, or decreased lymphatic function causing impaired ability to reabsorb fluid from the pleural space or other extravascular compartments (see also Fig. 81.1).
Many conditions can cause a pleural effusion via one or more of these pathophysiologic mechanisms. Examples include congestive heart failure, nephrotic syndrome and other hypoalbuminemic states, infectious diseases, inflammatory diseases, neoplasms, and others. Secondary pleural fluid collections can result from ascites, fistula drainage, or other abdominal fluids directly extending from the abdomen into the pleural space. They can also result from iatrogenic administration of fluid into the pleural space as can occur with certain CSF shunts and with mishaps involving administration of crystalloid, colloid, or intravenous alimentation fluids via faulty vascular access. Other extrathoracic circumstances are very rare causes of secondary effusions. Empyema is a collection of pus that usually results from extension of a pneumonia or lung abscess into the pleural space or from a pleural effusion that subsequently becomes directly infected. Chylothorax, due to injury or other problems related to the thoracic duct, is an uncommon type of pleural fluid collection. It is also possible to have a mixture of air and/or fluid and/or blood accumulate in the pleural space due to combinations of the various aforementioned mechanisms.
The thorax normally performs several important physiologic functions that may be adversely impacted by a pleural collection of air and/or fluid. The thorax acts like a pump to produce gas exchange via the lungs. Inspiratory excursions of the diaphragm and chest wall generate net negative intrathoracic pressures with respect to the ambient atmosphere and, thereby, achieve normal lung expansion during inspiration. The juxtaposition of the visceral pleural membrane covering the outer lung and the parietal pleural membrane covering the intrathoracic surfaces of the thoracic wall, diaphragm, and mediastinum is maintained by the viscoelastic force generated by the trivial amount of lubricating fluid normally contained in the pleural space. Without this viscoelastic effect, the lung would tend to elastically recoil, lose contact with the chest wall and diaphragm, and not inflate well during inspiration. The viscoelastic effect of the pleura also is very important for normal exhalation function. The expiratory phase of breathing is normally mostly passive due to elastic recoil forces in the chest wall and lungs. The apposition of the visceral and the parietal pleural membranes counteracts the residual elastic recoil force of the lungs once the chest wall and diaphragm have reached their end-expiratory position. This protects against further lung collapse at end-expiration. As a result, the lungs remain partially inflated and in contact with the chest wall, diaphragm, and mediastinum at end-expiration. The serous nature of the pleural membrane, along with the scant amount of lubricating pleural fluid that is normally present, also allows the visceral and parietal pleurae to slide against each other throughout the phases of breathing without significant friction or irritation.
Progressive filling of the pleural space with air, fluid, and/or blood causes a gradual loss of effectiveness of the respiratory pump function of the thorax. Abnormal increases in pleural volume directly impinge on lung volume. In addition, loss of the normal viscoelastic approximation between the visceral pleura and the parietal pleural predisposes to subtotal or even total collapse below the usual end-expiratory volume of affected areas of the lung. Once an area of lung collapses, substantial additional inspiratory force (pressure) must be supplied to restore the collapsed lung to normal end-expiratory volume. When atelectasis is present, discordant lung inflation may occur because the normal lung requires less pressure to achieve inflation and may be preferentially inflated and even hyperinflated compared to the collapsed lung. Atelectatic areas of lung also have a significantly lower pulmonary vascular resistance compared to inflated lung. This results in shunting of blood through collapsed portions of lung. Atelectasis-associated shunting can result in significant hypoxemia. Patients who have significant amounts of collapsed lung can also be more sensitive to intravascular volume deficits. Infusing fluid intravascularly will often improve hemodynamics in such cases.
The impaired respiratory pump function accompanying significant pleural air and/or fluid collections results in a compensatory increase in work of breathing to maintain satisfactory ventilation. Normally, work of breathing represents about 5 percent of total body oxygen consumption. Supranormal work of breathing requires increased perfusion of respiratory muscle to meet their increased oxygen needs. The respiratory muscles of patients with severely increased work of breathing can consume up to 8 to 10 times more oxygen than those of normally breathing individuals. Patients who are seriously ill or injured may not be able to meet or sustain the oxygen delivery needs of their respiratory muscles when intrapleural air or fluid causes their work of breathing to increase. Decreasing the work of breathing by draining an abnormal pleural air or fluid collection may be very beneficial in such cases.
In addition to deleterious effects on lung ventilation, perfusion, and mechanics, intrapleural air and/or fluid collections can significantly affect the cardiovascular system. Air and/or fluid in the pleural space not only occupy intrapleural volume, but also may increase the relative pressure inside the thorax and sometimes shift the position of the mediastinum. This affects the circulation in several ways. First, indirect compression
and/or altered transmural pressures affecting the heart results in decreased cardiac output. Second, altered transmural pressure also compresses the great veins, causing decreased venous return to the heart. Third, increases in intrathoracic pressure may significantly reduce the transthoracic pressure gradient that normally favors venous return into the thorax. These effects on venous return can seriously decrease cardiac output and are analogous to the effects produced by positive pressure ventilation. Cardiac output diminishes further if the pressure becomes great enough to shift the mediastinal position, distorting and obstructing vessels. Pressure alterations within the thorax from pleural air and/or fluid collections also can affect ECG tracings and invasive hemodynamic monitoring values and waveforms.
and/or altered transmural pressures affecting the heart results in decreased cardiac output. Second, altered transmural pressure also compresses the great veins, causing decreased venous return to the heart. Third, increases in intrathoracic pressure may significantly reduce the transthoracic pressure gradient that normally favors venous return into the thorax. These effects on venous return can seriously decrease cardiac output and are analogous to the effects produced by positive pressure ventilation. Cardiac output diminishes further if the pressure becomes great enough to shift the mediastinal position, distorting and obstructing vessels. Pressure alterations within the thorax from pleural air and/or fluid collections also can affect ECG tracings and invasive hemodynamic monitoring values and waveforms.
Simple pneumothoraces and hydrothoraces can produce near-atmospheric pressure in the affected pleural space (Fig. 29.2 A,C). Either can impair normal physiology through one or more of the aforementioned mechanisms. Patients who are otherwise healthy generally tolerate the impaired ventilation that results from partial or even total collapse of a single lung such as might occur with moderate to severe instances of either of these conditions. Cardiovascular embarrassment is generally mild to moderate and satisfactorily tolerated in otherwise healthy and euvolemic individuals. Serial physiologic assessment is very important to assure maintenance of pulmonary and cardiovascular function whenever a significant pleural air or fluid collection is present. Significant tachypnea, dyspnea, tachycardia, hypoxemia, hypotension, or changing mental status should raise concerns that pulmonary or cardiovascular compromise is not being adequately tolerated or is worsening. Maintenance of adequate intravascular volume is particularly important to compensate for the abnormal venous return when larger air or fluid collections are present. Because simple pneumothoraces and hydrothoraces are generally adequately tolerated by healthy patients, therapeutic thoracostomy can usually be performed semi-electively or urgently in such cases. Rarely, severely affected patients and those with significant comorbid conditions may require an emergency thoracostomy procedure.
The potential cardiovascular consequences of hemothoraces and tension pneumothoraces are much more significant than those associated with simple pneumothoraces and simple pleural effusions. Hemothoraces cause the same volume and pressure impacts on intrathoracic structures that occur with other intrapleural air or fluid collections but often cause additional direct and serious cardiovascular compromise due to the intravascular blood loss that they represent. Because hemothoraces result in loss of red blood cells from the intravascular compartment, there is decreased oxygen delivery to tissues. In other words, blood loss associated with hemothoraces impacts physiologic functioning in three ways; it produces hypoxemia, hypovolemia, and anemia. Restoration of intravascular volume with crystalloid solutions is usually satisfactory for small hemothoraces. Blood replacement with packed red blood cells or autotransfused blood recovered from the hemothorax may be necessary when a larger hemothorax is present (see Chapter 30). In this type of situation, hemoglobin levels must be restored to overcome anemic cardiac dysfunction and hypoxemia.
The severity of a hemothorax can be influenced by several factors. The vascular pressure head of the pulmonary circulation is usually significantly less than that of the systemic circulation. Therefore, bleeding from a site supplied by the pulmonary circulation tends to be less severe and more likely to cease spontaneously than bleeding from a site supplied by the systemic circulation. Coagulation status also influences severity. Blood contained in a hemothorax can play a role in controlling further intrathoracic bleeding. The hemothorax can help produce a covering clot or occasionally help tamponade a bleeding site. As a consequence, thoracostomy drainage of a hemothorax can occasionally cause resumption or worsening of intrathoracic bleeding by removing blood and clots that are helping to control hemorrhage. This should not prevent the performance of a thoracostomy procedure that is clearly indicated to treat a hemothorax. Thoracostomy drainage of a significant hemothorax provides the clinician with the ability to directly measure the amount and rate of blood loss into the pleural space. This can be invaluable for guiding fluid and blood replacement as well as contributing to a determination that a thoracotomy or other operative intervention is likely to be necessary. There is often no effective way to apply direct pressure to an intrathoracic bleeding site, so surgical intervention is indicated for persistent, significant bleeding. Tube thoracostomy also enables autotransfusion of blood contained in a hemothorax.
Tension pneumothoraces are the most dangerous of all pleural conditions. Tension pneumothoraces result when the pressure exerted by air contained in the pleural space becomes supra-atmospheric. They can be life-threatening even in very healthy individuals when the pleural pressure becomes high enough to seriously impede venous return into the chest, compress cardiovascular structures, or obstruct intrathoracic blood vessels (Fig. 29.2B). The cardiovascular compromise resulting from a tension pneumothorax is often the clinical finding that suggests the diagnosis. Acute changes in end-tidal CO2 concentration or capnography waveform may, however, suggest the diagnosis even before cardiovascular compromise develops because these monitors detect acute changes in ventilation and pulmonary blood flow within a few breaths of their onset. Declining oxygen saturation as determined by a pulse oximeter may also be an early sign of deterioration in cardiopulmonary function due to a pneumothorax. Death from a tension pneumothorax is usually due to the cardiovascular pathophysiology just described. Increasing intravascular volume and cardiac inotropy may help offset some of these deleterious effects. However, in all but the mildest cases, such interventions are inadequate to restore cardiovascular function. Therefore, thoracostomy drainage of tension pneumothoraces should be viewed as the initial and most important therapeutic intervention for such cases. By performing a thoracostomy procedure, the supra-atmospheric pressure can be relieved and the tension pneumothorax can be effectively converted to a
simple pneumothorax with non-life-threatening cardiovascular consequences. After satisfactory thoracostomy drainage of a tension pneumothorax is achieved, additional cardiovascular support measures can be considered and implemented as warranted.
simple pneumothorax with non-life-threatening cardiovascular consequences. After satisfactory thoracostomy drainage of a tension pneumothorax is achieved, additional cardiovascular support measures can be considered and implemented as warranted.
Many patients maintain spontaneous respiration before and after a thoracostomy procedure is performed. However, some patients require positive pressure ventilation. It is important to understand the impact of positive pressure ventilation on chest physiology in general and on thoracostomy management in particular.
During spontaneous breathing, intrathoracic pressure is subatmospheric during the inspiratory portions of the breathing cycle. This is important for promoting venous return into the thorax and the heart. When positive pressure ventilation is administered, the inspiratory phase of the breathing cycle becomes supra-atmospheric and thus impedes venous return to the heart. This effect can be particularly important in patients requiring high mean airway pressures to achieve adequate ventilation, in patients with intravascular volume deficits (e.g., hypovolemic shock) and in patients with conditions that cause inappropriate vasodilatation or distribution of perfusion (e.g., septic shock, neurogenic shock, anaphylactic shock). The cardiovascular consequences of positive pressure ventilation are further aggravated whenever a significant air and/or fluid collection are present in the pleural space. The clinician should, therefore, anticipate a sudden worsening of the patient’s hemodynamic status at the initiation of positive pressure ventilation in such cases. Intravascular fluid infusion to augment intravascular volume and/or cardiotonic medication to improve cardiac performance and/or vasoactive medication to favorably improve vascular tone may be required. Emergent thoracostomy drainage of a significant pleural air and/or fluid collection may provide substantial additional cardiovascular benefit. Apart from the usual effects of positive pressure ventilation on venous return, induction of a tension pneumothorax also should be considered when a patient undergoes cardiovascular deterioration after the initiation of positive pressure ventilation.
Another important physiologic consideration arises whenever a patient with a pneumothorax will experience significant changes in ambient atmospheric pressure over a relatively short period of time as can occur during transportation via aircraft, travel across widely changing elevations, or administration of hyperbaric therapy. Pneumothoraces that do not readily communicate with the ambient atmosphere are unable to equilibrate with changing atmospheric pressure except very slowly by diffusion of gas across the pleural membrane. Because gas does not flow rapidly into nor out of a noncommunicating pneumothorax, it approximates a closed system and the usual gas laws of physics apply (e.g., P1V1 = P2V2). Therefore, if ambient atmospheric pressure becomes significantly less than the pressure in the pneumothorax, the pressure in the pneumothorax acutely becomes relatively supra-atmospheric, creating in effect a tension pneumothorax. Conversely, if the ambient atmospheric pressure acutely becomes significantly greater than the pressure in a noncommunicating pneumothorax, then the pressure in the pneumothorax will become relatively subatmospheric and the pneumothorax will decrease in size acutely. If extrathoracic air pressure remains relatively higher than the pressure in the pneumothorax over a period of time, then the air in the pleural space and the ambient atmosphere will begin to approach equilibrium. When, after significant equilibration occurs, the patient acutely transitions to a lower atmospheric pressure, the intrapleural air pressure acutely will become relatively supra-atmospheric and behave as a tension pneumothorax. Almost all pneumothoraces can act this way because they are caused by a relatively small hole in the lung parenchyma or a bronchus that does not allow quick equilibration of pleural space pressure. Performing a thoracostomy protects against the effects of rapid changes in atmospheric pressure because the communication created through the chest wall allows constant rapid and free airflow to maintain pressure equilibrium between the pleural space and the atmosphere. The pneumothorax thus is converted to an open system that will not develop supra-atmospheric pressure. Instead, when ambient atmospheric pressure changes acutely the necessary amount of air flows immediately across the thoracostomy drainage device until the pressure in the pleural space equals the new barometric pressure. (Typically, a one-way valve is interposed in the drainage system between the pleural space and the atmosphere. This arrangement allows air to escape the pleural space whenever intrapleural pressure becomes supra-atmospheric and prevents air from entering the pleural space whenever the intrapleural pressure becomes subatmospheric. Although not a totally free communication, such an arrangement does effectively prevent the unwanted development of a tension pneumothorax.)
An analogous situation can occur when the partial pressure of a gas that is being breathed changes significantly with respect to the air contained within a pneumothorax. This is an important consideration when an inhaled anesthetic agent is administered to a patient with a pneumothorax. Administered anesthetic gases will accumulate in the pneumothorax as long as the partial pressure of the anesthetic gas in the lung (and, hence, the circulating blood) is higher than in the pneumothorax. After anesthetic gas administration is discontinued, residual anesthetic gas in the circulating blood is cleared by the lungs and exhaled. However, the pneumothorax will also contain residual anesthetic gases that are not so readily mobilized. Anesthetic gas in the pneumothorax may take some time to diffuse back into the blood. As anesthetic gas diffuses out of the pneumothorax and into the circulation, systemic anesthetic effects may persist or recur. These same principles are used to the patient’s benefit when a clinician administers air with a higher than normal concentration of oxygen (and correspondingly lower than normal concentration of nitrogen) to hasten the resolution of a pneumothorax. This generates a partial pressure gradient favoring diffusion of nitrogen out of the pneumothorax. Oxygen contained in the pneumothorax is reabsorbed readily because oxygen that diffuses into nearby tissues is used for aerobic metabolism
and because the high affinity of hemoglobin and low partial pressure of oxygen in nearby capillaries favors reabsorption of oxygen from the pneumothorax. Placing a thoracostomy drainage device essentially eliminates issues related to partial pressure differentials across the pleural membrane.
and because the high affinity of hemoglobin and low partial pressure of oxygen in nearby capillaries favors reabsorption of oxygen from the pneumothorax. Placing a thoracostomy drainage device essentially eliminates issues related to partial pressure differentials across the pleural membrane.
Some patients develop pulmonary edema upon re-expansion of compressed or atelectatic portions of a lung following drainage of a pleural air or fluid collection. The physiologic mechanism for this is not clear. The development of dyspnea or hypoxemia, auscultatory findings, and radiographic evidence usually establish the diagnosis. Therapy is supportive until spontaneous resolution occurs.
Extrathoracic structures may be indirectly but severely impacted by the effects of intrathoracic volume or pressure changes. For example, intracranial pressure may rise if increased intrathoracic pressure interferes with venous blood flow from the head into the thorax. In the setting of head trauma or other conditions in which intracranial pressure is already elevated, a further rise in intracranial pressure might be devastating. Pleural air or fluid accumulations can also affect intracranial pressure if they interfere with ventilation and thereby cause an increase in pCO2 or a decrease in pH. Likewise, serious and even fatal exacerbations of other conditions that are critically related to acid-base status (e.g., pulmonary hypertension, certain poisonings, metabolic disorders, respiratory failure, vasopressor therapy, shock) may occur whenever pleural space problems decrease pulmonary ventilation with a resultant increased pCO2 or decreased pH.
Occasionally, the physiologic impact of a pneumothorax, hydrothorax, or hemothorax can be ameliorated by changing the position of the patient. In general, gravity causes the dependent lung to receive relatively more blood flow and to ventilate less effectively than the other lung. Positioning the patient on one side versus the other, or even using the prone position may take advantage of gravity to produce a more favorable situation. The effect of such maneuvers is usually small but can be significant.
Indications
Thoracostomy procedures are most commonly indicated to drain air, blood, or other fluid from the pleural space. The patient’s history or exam will often provide the initial clue to the presence of one of these conditions. For example, the patient may have a history of trauma, recent surgery, pain, dyspnea, or disease involving the chest. Typical examination findings may include: abnormalities or changes in vital signs measurements reflecting respiratory function, cardiac function, or both; signs of trauma; abnormal auscultatory findings, abnormal percussion findings; signs of prior surgery; the presence of certain medical devices; and signs of disease states affecting the chest. Imaging studies or other interventions may occasionally serve to initially identify pleural space problems and are often used to confirm the presence of abnormal contents in the pleural space (10,11,12,13,14,15,16,17,18,19,20,21,22,23,24,25).
Once it is suspected that air and/or fluid is present in the pleural space, the clinician can perform additional physical examination. Careful percussion and auscultation of the chest are especially useful to help confirm these suspicions and to clarify the severity of the situation. Imaging studies, such as frontal and lateral view plain chest radiographs, may further help elucidate the nature, location, and degree of the problem(s). More specialized imaging studies, such as decubitus view chest radiographs or computerized tomography imaging of the chest, can provide additional useful information in selected cases. In particular, special imaging can help the operator determine whether or not intrapleural fluid is free flowing and drainable with a thoracostomy catheter or tube.
Progressive filling of the pleural space with air, fluid, and/or blood usually causes progressive physiologic deterioration and compensatory physiologic responses are often the earliest clinically recognizable warning signs of these conditions. As the disorder progresses, more dire warning signs of organ dysfunction appear. An individual patient may present at any point along this spectrum of worsening physiologic impact from essentially asymptomatic to extremis depending on his/her individual circumstances.
The pulmonary and/or the cardiovascular systems are most commonly and most significantly impacted by serious pleural space problems. Therefore, findings of abnormal pulmonary function (ventilation, oxygenation, work of breathing, or pulmonary mechanics) or abnormal cardiovascular function (heart rate, rhythm, blood pressure, perfusion, or oxygenation) are the most important warning signs of significant intrapleural air and/or fluid collections. Local pain, findings associated with significant blood loss, the presence of blunt or penetrating chest trauma, or additional manifestations of disease processes affecting the chest are other clues to the presence of air and/or fluid in the pleural space.
Once a pleural air and/or fluid collection is confirmed, the clinician must determine its physiologic importance. By understanding the physiologic impact and the anticipated course of a pleural air and/or fluid collection, the need for and relative timing of a thoracostomy procedure can be properly determined. An appreciation of the potential impact of other coexisting conditions or other planned interventions will sometimes affect these decisions.
Pneumothoraces, hydrothoraces, and/or hemothoraces that are very small, well-tolerated, and not expected to worsen, can often be monitored without intervention unless worsening or complications occur. Aspiration of fluid solely for diagnostic purposes may or may not be desirable in some of these cases. Therapeutic thoracostomy becomes clearly indicated if significant physiologic embarrassment is present, awaiting spontaneous resolution poses unacceptable risk, or serious worsening is anticipated.
Pulmonary and/or cardiovascular compromise is the primary indication for a thoracostomy procedure. Rarely, other indications arise. Perhaps the most significant of these is the problem of achieving good antibiotic concentrations in pleural fluid. Infected pleural fluid collections are akin to abscesses
elsewhere. Because there are no vascular beds within the pleural fluid collection itself, drug concentrations in pleural effusions depend on diffusion of the drug from nearby vascular beds, resulting in lower antimicrobial concentrations. Therefore, better and faster resolution of some types of infections may result when infected fluid or blood is removed. Often, knowledge of the properties of the infecting organism(s) and the specific antimicrobial agent(s) will help determine when drainage of an infected pleural effusion is indicated. This issue can become more complicated if one or more loculated intrapleural fluid collections are present. In such cases, the risk of a poorly responding or persistent infection may increase. The difficulty associated with achieving good thoracostomy drainage also increases. Failure to drain infected pleural fluid when indicated can result in significant complications. However, these are more often a concern for the physician managing the patient in the hospital than for the emergency physician.
elsewhere. Because there are no vascular beds within the pleural fluid collection itself, drug concentrations in pleural effusions depend on diffusion of the drug from nearby vascular beds, resulting in lower antimicrobial concentrations. Therefore, better and faster resolution of some types of infections may result when infected fluid or blood is removed. Often, knowledge of the properties of the infecting organism(s) and the specific antimicrobial agent(s) will help determine when drainage of an infected pleural effusion is indicated. This issue can become more complicated if one or more loculated intrapleural fluid collections are present. In such cases, the risk of a poorly responding or persistent infection may increase. The difficulty associated with achieving good thoracostomy drainage also increases. Failure to drain infected pleural fluid when indicated can result in significant complications. However, these are more often a concern for the physician managing the patient in the hospital than for the emergency physician.
Pleural air or fluid collections related to certain conditions are associated with long resolution times, greater complication risks or less favorable outcomes if allowed to spontaneously resolve. In particular, drainage is beneficial, even in the absence of physiological embarrassment for pleural effusions caused by certain infectious diseases, inflammatory processes, or other types of conditions that tend to produce scarring or adhesions involving intrathoracic structures. Analysis of drained pleural fluid using culture, serology, chemical analysis, or other diagnostic tests can often facilitate diagnosis of many of these conditions. Usually thoracentesis is preferred when pleural fluid is to be removed for diagnostic purposes only (see Chapter 81).
Patients with pneumothoraces are at significant risk for worsening whenever positive pressure ventilation is administered. As a rule, a therapeutic intrapleural drainage device should be placed in all such cases to prevent the development of a devastating tension pneumothorax. Likewise, certain circumstances, such as a need for interhospital aeromedical transportation, a requirement for major surgery, a lack of an available appropriate inpatient bed, or other situations that will sufficiently impair or preclude the ability to monitor a patient effectively and/or perform a thoracostomy if it becomes needed, should be construed as strong general indications to perform an antecedent therapeutic thoracostomy.
Occasionally, patients who have significant amounts of air, blood, or fluid in their pleural space will have pre-existing or concomitant conditions that additionally impact their pulmonary or cardiovascular physiology beyond that due to their pleural space pathology. Pre-existing conditions that can be of additive physiologic significance may include congenital or acquired heart disease, asthma or chronic lung diseases, and anemia. Patients with trauma-related pleural air or blood collections frequently have associated lung injuries and occasionally have cardiac or great vessel injuries. They also may have significant derangements due to blood losses in the chest or elsewhere. Patients with pleural effusions due to infectious or inflammatory disorders may also have co-existing direct involvement of their lungs or heart due to their primary condition or indirect involvement of these organs due to circulating mediators or toxins. Additionally, pre-existing or concomitant conditions or therapeutic interventions may interfere with the patient’s ability to compensate for the physiologic effects of pleural air or fluid collections. The patient therefore should be assessed for conditions and medications that might impact his/her ability to compensate for physiologic compromise.
Thoracostomy procedures are occasionally needed to facilitate other interventional procedures or to manage their complications. For example, a thoracostomy procedure may be needed to manage a pneumothorax complicating subclavian vein catheterization. When a patient already has a thoracostomy tube in place, procedures such as subclavian vein catheterization, which may induce a pneumothorax or hemothorax, should be performed on the same side as the pre-existing chest tube in order to avoid further complications.
Rarely, a pneumothorax can arise from a large rupture or tear of the trachea or a major bronchus. The resistance to airflow through a tracheobronchial hole lessens as the hole becomes larger. So, air will preferentially flow into the pleural space rather than the lung through a large defect. This will produce serious hypoventilation and resultant hypoxemia. The presence of a large tracheobronchial disruption is suggested by significant airflow out of the thoracostomy tube with each inspiration. Positive pressure ventilation using a rapid rate, low inspired and mean airway pressures, and a high concentration of inspired oxygen will improve lung ventilation and oxygenation. A functioning thoracostomy drainage device will prevent development of a tension pneumothorax. In this circumstance, suction should not be applied to the drainage device in order to minimize the pressure gradient favoring airflow across the tracheobronchial rent.
Different sizes of thoracostomy catheters and tubes are available to accommodate the spectrum of patient sizes from preterm neonate to obese adult. The criteria for selecting an appropriately sized catheter or tube for an individual patient are described in the following section. Beyond choosing a catheter or tube that is appropriate for the patient’s size, it is also necessary to consider the type and volume of material that must be drained from the pleural space. Generally, air and freely flowing serous fluid can be very effectively drained with a modestly sized catheter. However, blood, pus, or other viscid fluid should be drained with a large bore chest tube. If autotransfusion of blood from a hemothorax is contemplated, then an autotransfusion apparatus is needed (Chapter 30).
When formulating a risk-benefit analysis regarding the performance of a thoracostomy procedure, the operator should consider not only the direct risks of the thoracostomy procedure itself but also the associated risks of periprocedural sedation and of coexisting conditions. (See the Complications section below.)
Procedures
Other than issues related to the sizing of equipment, essentially no significant modifications are required when performing thoracostomy procedures in children versus adults. However, the practitioner must choose the most appropriate management strategy for each clinical situation. These management strategies and their attendant advantages and disadvantages are described below (8,13,14,15,17,23,26,27,28,29,30,31,32).
Conservative Management
In some cases, small, unilateral pneumothoraces can be managed conservatively. This option can be considered for stable older children and adolescents who have no evidence of respiratory distress, who will not undergo surgery, be placed in a hyperbaric chamber, or be transported by aircraft. However, there is some debate as to what constitutes a “small” pneumothorax. Many have suggested that pneumothoraces as large as 20 percent of the pleural space might be managed conservatively. But, there are few methods by which the clinician can accurately estimate the size of a pneumothorax using the results of imaging studies. Perhaps the most simple and accurate of these methods was developed by Rhea (18) who developed an (adult-based) nomogram based upon the average intrapleural distance (Fig. 29.3).
Needle Thoracostomy
Indications
Although a needle thoracostomy can temporarily relieve most pneumothoraces, the primary indication for this procedure is the emergent palliative decompression of a tension pneumothorax pending the placement of a larger thoracostomy tube. Needle thoracostomy can also assist in the diagnosis of tension pneumothorax. Needle thoracostomy can occasionally serve as the definitive procedure for neonates and small children but even in these cases there is often ongoing air leak into the pleural space which requires treatment with a thoracostomy catheter.
Equipment
Angiocath (catheter over needle device) or butterfly type needle (neonates)
Sterile prep solution
Sterile gloves
Procedure
The operator should select an insertion site on a nondependent area of the chest wall on the affected side(s). If the patient is supine then the 2nd or 3rd intercostal space in the midclavicular line is typically chosen (Fig. 29.4). However, other interspaces may be used if potential complications make the infraclavicular site undesirable. If the patient is not supine, then an antidependent area of an intercostal space can be used. The proposed site for the procedure should be chosen specifically to avoid entering the heart, traversing the diaphragm into the abdomen, and to minimize other risks. The insertion site should be sterilely prepped with antibacterial solution. A syringe is then partially filled with a small amount of sterile saline and attached to the angiocath device. Using sterile technique, the angiocath device is inserted perpendicularly just through the skin at the intended site on the chest wall (Fig. 29.5). The syringe should be left in place during insertion. The angiocath is then advanced through the intercostal muscles and the pleura. Some operators prefer to grasp the shaft of the angiocath firmly between the thumb and fingers of their free hand at a distance above the skin about equal to the anticipated thickness of the patient’s chest wall so that their fingers will act as a stop during advancement of the angiocath device. This helps to avoid unintentional, overly deep penetration of the angiocath into the thorax. The angiocath should be advanced immediately over the superior aspect of the rib that forms the inferior margin of the intercostal space. This avoids injury to the neurovascular bundle that courses along the inferior border of each rib. Some operators prefer to intentionally touch the outer surface of the rib with the angiocath needle, ‘walk’ the needle over the superior aspect of that rib, and then advance the needle into the pleural space in an attempt to further minimize the risk of injury to the neurovascular bundle. Upon entering the pleural space, the operator should perceive a modest decrease in resistance to further advancement of the angiocath. Once the pleural space is entered, the angiocath should not be advanced further. The operator should assess for air return from the pleural space. This helps determine the presence or absence of tension or simple pneumothorax.
![]() Figure 29.4 Site for needle thoracostomy evacuation of a tension pneumothorax: 2nd interspace, midclavicular line. |

Needle Thoracostomy
Assemble all equipment and assign duties to personnel.
Explain the procedure and obtain consent (as indicated).
Position the patient, and prepare and anesthetize (as time allows) the skin and subcutaneous structures.
Advance the over-the-needle catheter device over superior aspect of rib while maintaining negative pressure in syringe.
When air returns, remove the needle while leaving catheter in place.
Evacuate the pneumothorax.
Obtain a chest radiograph.
![]() Figure 29.5 Needle thoracostomy. An angiocath attached to a syringe partially filled with saline is advanced over the rib, and air is aspirated when the needle enters the pleural space. |
If a tension pneumothorax is present then immediately upon entering the pleural space, air bubbles will often be noted in the fluid-filled syringe. Depending on the initial volume of the tension pneumothorax and the ongoing rate of air leakage, further aspiration may be required to fully relieve the tension physiology. When larger air leaks are present, then removal of the syringe from the angiocath will facilitate more rapid drainage of intrapleural air. Rarely, an additional needle thoracostomy may be needed to achieve satisfactory decompression of a tension pneumothorax while preparations for a more definitive procedure are undertaken. Relief of the tension associated with a pneumothorax will relieve cardiopulmonary compromise. Persistent, severe cardiopulmonary compromise following a needle thoracostomy suggests that either the tension pneumothorax has not been adequately decompressed or the patient has another coexisting condition.
If a simple pneumothorax is present, then air return into the syringe will not occur spontaneously but will occur with aspiration. If neither a tension pneumothorax nor a simple pneumothorax is present, then there will be no significant return of air into the syringe either spontaneously or with aspiration. Occasionally, the angiocath will enter the surface of the lung and a tiny amount of air will be aspirated with each breathing cycle.
Some operators prefer not to attach a syringe partially filled with saline to the angiocath when advancing the device into the pleural space. This option may be less desirable because spontaneously breathing patients may suck air into their pleural space during inspiration via the uncovered angiocath hub thus creating a pneumothorax. If a procedure-related pneumothorax occurs, it may cause confusion because the clinician may have difficulty determining whether the pneumothorax is due to the procedure or to another cause. Patients who are receiving positive pressure ventilation and are not spontaneously breathing do not have this problem because intrathoracic pressure never becomes subatmospheric. When a needle thoracostomy is performed without a syringe attached to the angiocath, the presence or absence of a tension pneumothorax can generally be inferred by the following criteria: (i) tension pneumothorax is present when a return of air occurs via the angiocath needle as the pleural space is entered; (ii) air flowing out of the hub of the angiocath can be felt against an area of the operator’s skin or, often heard as a hissing or whistling type of sound. (If the patient is spontaneously breathing there might be some sucking of air through the angiocath with inspiration. This should not be mistaken for air egressing from the angiocath); (iii) tension pneumothorax is not present if there is no return of air as the angiocath enters the pleural space. (iv) Relief of the tension pneumothorax should alleviate cardiopulmonary compromise. When performing a needle thoracostomy on a preterm or term neonate some operators prefer to use a butterfly type of needle with the manufacturer’s tubing left attached. The hub of the tubing is immersed in a 4 ounce baby bottle of sterile water. A piece of tape along the rim of the bottle is used to hold the tubing in a position that keeps the hub under the surface of the water. The bottle is then placed alongside the baby and the butterfly needle is used to enter the pleural space. Air bubbles emerging from the hub of the tubing are indicative of a tension pneumothorax.
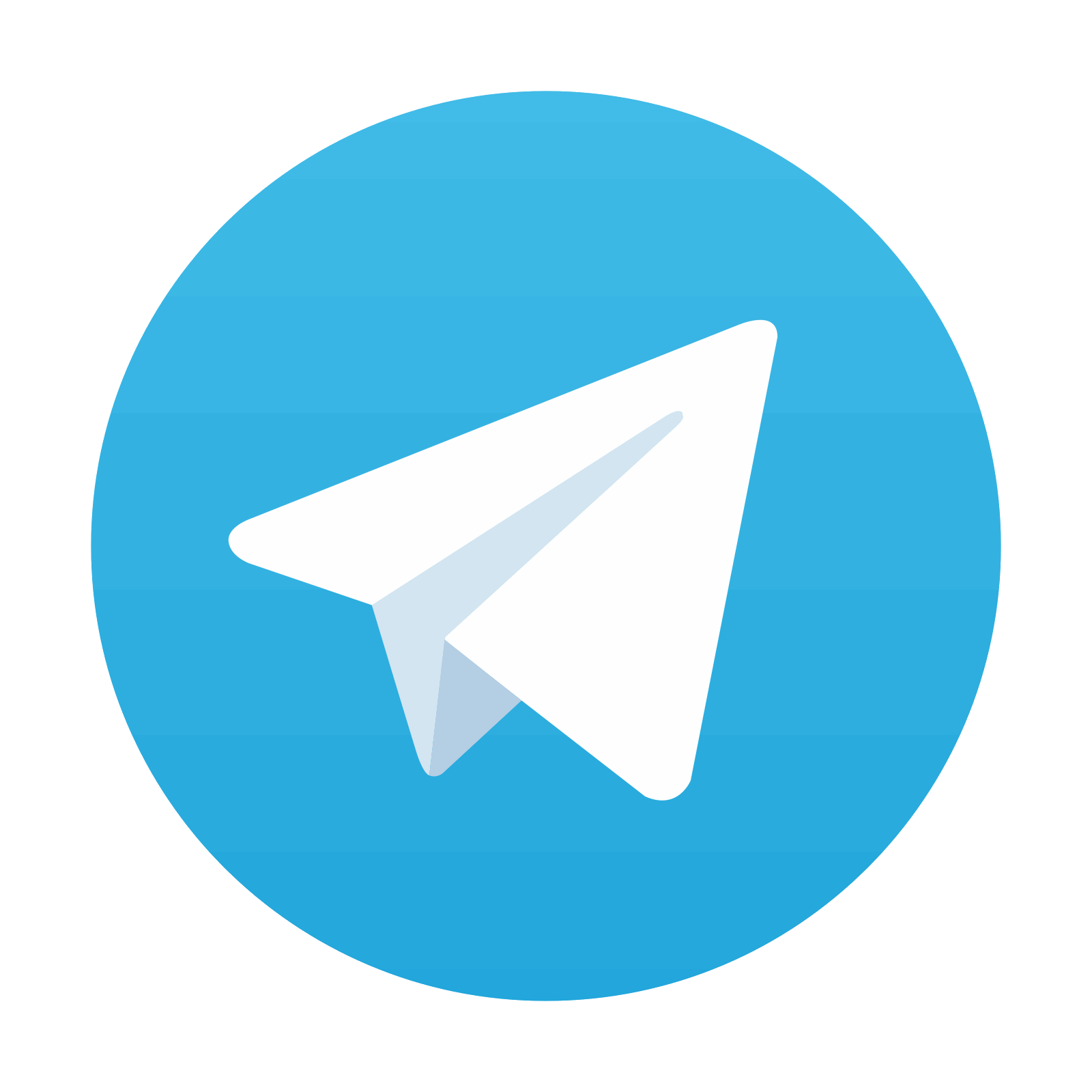
Stay updated, free articles. Join our Telegram channel

Full access? Get Clinical Tree
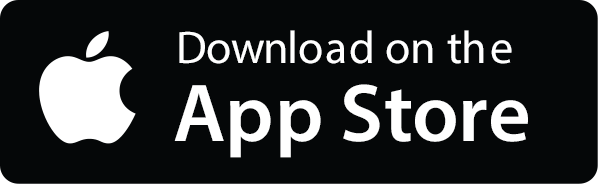
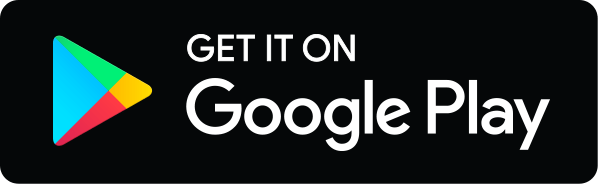
