Therapy for Cardiorespiratory Failure in the Neonate
Assisted ventilation and oxygen therapy remain the standard of care for neonatal respiratory failure. A few term and preterm infants with a wide range of diagnoses develop intractable cardiorespiratory failure despite maximal ventilatory support. In these patients, severe pulmonary hypertension often contributes to persistent hypoxemia.
Two unique therapies for such patients are presented in this section: extracorporeal membrane oxygenation (ECMO), which is now well established as a rescue therapy for intractable respiratory failure in term and late-preterm neonates, and inhaled nitric oxide (iNO), a noninvasive inhalational therapy that can elicit selective pulmonary vasodilation.
Extracorporeal Membrane Oxygenation
In ECMO, techniques of cardiopulmonary bypass, modified from those originally developed for open heart surgery, are used over a prolonged period to support heart and lung function. In newborns with hypoxic respiratory failure, this allows the lungs to rest and recover, and prevents the often damaging effects of aggressive mechanical ventilation and 100% Fio2.
The use of ECMO offers support for term or late-preterm neonates with life-threatening cardiopulmonary disease. Because of serious inherent risks, such as systemic and intracranial hemorrhage, the procedure is currently reserved for neonates with reversible pulmonary disease in whom trials of conventional or high-frequency ventilation as well as inhaled nitric oxide have failed.
Several randomized trials have demonstrated improved survival in infants supported with ECMO. The most rigorous prospective, randomized trial was conducted in the United Kingdom, where 185 infants with severe respiratory failure were randomized to ECMO or conventional ventilatory management.21 Survival in the ECMO-treated patients was 68% compared with 41% survival in the control arm (p = .0005), equivalent to one extra survivor for every three or four infants allocated to ECMO. Neurologic outcome was similar among survivors of either treatment arm, making it unlikely that ECMO contributed any added morbidity to this cohort of critically ill newborns.
The clinical course of such infants is variable, depending on the severity of the underlying disease process and the degree to which PPHN contributes to the cyanosis. Diagnoses associated with severe hypoxic respiratory failure in neonates include meconium aspiration syndrome, respiratory distress syndrome (RDS), sepsis, pneumonia, and congenital diaphragmatic hernia (CDH). Idiopathic pulmonary hypertension is sometimes seen in patients who exhibit minimal or no lung disease and have clear chest radiographs. The International Extracorporeal Life Support Organization (ELSO) Registry reports the distribution of primary diagnoses and survival for patients undergoing bypass; data are shown in Figure 78-1.20

Traditional therapy for respiratory failure complicated by PPHN consists of the use of oxygen, mechanical ventilation, alkalosis, and pharmacologic vasodilators in an attempt to decrease pulmonary vascular resistance and increase blood flow to the lungs. Inhaled nitric oxide is a selective pulmonary vasodilator that enhances pulmonary blood flow and improves oxygenation in PPHN. For infants who do not respond to these measures, ECMO can be a useful therapy.
Basic Techniques
The standard ECMO procedure used in most newborn intensive care units today is venoarterial (VA) bypass because it provides both pulmonary and cardiac support. The right atrium is cannulated via the right internal jugular vein with a Silastic or polyvinyl chloride catheter (8- to 14-French diameter); blood is siphoned to a level below the heart where a roller head or centrifugal pump circulates the blood through the artificial lung. These pumps are servo regulated to slow or shut down if venous return is not adequate to meet circuit flow demands. As the blood circulates through the artificial lung, gas exchange occurs against a filtered mixture of oxygen and CO2. The artificial lung currently used in neonates is a hydrophobic, pediatric-specific, polymethylpentene hollow fiber membrane (Quadrox iD [diffusion membrane oxygenator]) that serves as a blood-gas interface, similar to the alveolar capillary membrane. This technology initially had only been used in older children (>10 kg) and adults, but is currently available to be used in the neonatal population. Gas flows through the hollow fibers and blood flows around the outside of these fibers. Oxygen and carbon dioxide flow down their diffusion gradients, and gas exchange occurs across the hollow fiber membrane. Advantages of this newer artificial lung include a low priming volume and reduced blood/foreign surface area contact. This new generation of artificial lung also incorporates a heat exchanger to rewarm the oxygenated blood returning to the patient.
Oxygenation can be regulated by varying blood flow through the ECMO circuit. The higher the volume of cardiac output diverted through the membrane lung, the better the oxygen delivery from the ECMO circuit. Oxygenated blood exiting the artificial lung is returned to the infant via an 8- to 12-French catheter positioned in the ascending aortic arch through a right common carotid artery cannulation.
Blood flow through the ECMO circuit, at a rate of 90 to 120 mL/kg per minute, is usually adequate to provide excellent cardiac and respiratory support with maintenance of adequate blood pressure and oxygenation. Arterial wave dampening with narrowed pulse pressure is noticeable at flow rates approaching the infant’s cardiac output because the ECMO circuit provides relatively nonpulsatile blood flow. Pressor support, vasodilators, and paralytic agents can usually be stopped while the infant is on venoarterial ECMO support because perfusion pressure from the pump largely replaces cardiac output. Systemic anticoagulation therapy with unfractionated heparin is administered for the duration of the bypass procedure to prevent clotting in the circuit and possible thromboembolization. Activated clotting times are measured hourly and are maintained within a range of 180 to 220 seconds. Some centers monitor antifactor Xa assays every 4 to 6 hours, maintaining them between 0.3 and 0.7 unit/ml.
Once the infant is placed on ECMO, ventilator and oxygen support is reduced to a minimum to avoid any further barotrauma or oxygen toxicity to the lung. The “ideal” rest settings are a topic of considerable debate. Infants can be maintained in room air with a peak inspiratory pressure of 14 to 20 cm H2O, an end-expiratory pressure of 10 to 12 cm H2O (lower if there is an air leak), mean airway pressure of 10 to 20 cm H2O and a ventilator rate (intermittent mandatory ventilation) of 10 to 20 for the duration of VA bypass. If volume ventilation is preferred, 3 to 6 mL/kg tidal volume is used to achieve mild chest rise with each delivered breath. This helps maintain lung inflation and allows for continued pulmonary toilet. The respiratory status of the infant can be monitored with intermittent arterial blood gases obtained from the umbilical or peripheral arterial line. An oxygen saturation electrode inserted on the venous side of the circuit allows continuous monitoring of the mixed venous saturation at the level of the superior vena cava and right atrium. A mixed venous saturation of 70% or greater reflects adequate oxygen delivery.
As lung function improves or pulmonary hypertension abates, or both, the mixed venous saturation and partial pressure of arterial oxygen (Pao2) rise above the baseline oxygenation provided through the artificial lung. Blood flow through the artificial circuit can then be decreased in small increments (10 to 20 mL) while mixed venous and arterial oxygenation remain adequate. When ECMO flow has been reduced to 10 to 20 mL/kg per minute, the infant can be weaned from extracorporeal support.
Increased ventilator support is provided as the patient approaches decannulation. Bypass support may be required for 2 to 60 days with an average need for 8.5 days of support.20 Continued ventilator support following ECMO can be quite variable (days to weeks) depending on the underlying cause of respiratory/cardiac failure, as well as the degree of barotrauma incurred before initiation of ECMO.
In most ECMO centers, the right carotid artery and internal jugular vein are permanently ligated at decannulation. This approach is technically easier and prevents any concern about acute embolic complications associated with vascular reconstruction. However, some surgeons reanastomose these vessels if the patient has been on bypass less than 10 days to prevent future ischemic risks to the developing newborn brain as well as during later adult life. Studies show no disadvantage to this approach. Magnetic resonance angiograms and Doppler flow studies confirm good antegrade flow through the reconstructed carotid artery postoperatively in the majority of these infants. Few studies have evaluated the long-term patency of the reconstructed carotid and whether reconstruction improves long-term neurologic outcomes. In the few studies available, ultrasound vascular evaluations confirm good antegrade flow through the vessels in most cases, with no embolic sequelae reported.19,55 Neurodevelopmental outcome and neuroimaging were equally favorable. Longer-term neurologic follow-up of these patients compared with ECMO survivors with carotid ligation is of interest to surgeons and neonatologists alike. Carotid artery ligation carries a small but definite risk for development of ischemic sequelae during VA bypass, whereas the risk for future ischemic stroke remains unknown.
Venovenous Extracorporeal Membrane Oxygenation
A growing experience supports the ability of venovenous ECMO to provide adequate oxygen delivery in patients with serious respiratory failure but adequate heart function. In adults, this technique usually involves draining desaturated blood from the right atrium and returning oxygenated blood through the femoral vein.
Venovenous (VV) bypass in infants has been greatly improved by the development of double-lumen catheters of varying sizes (13, 15, 18 French) that allow bypass support with cannulation of the right atrium alone. Desaturated blood is withdrawn from the right atrium through the outer fenestrated venous catheter wall. Oxygenated blood from the ECMO circuit is returned to the inner arterial catheter, which is positioned to direct blood across the tricuspid valve. Higher flow rates are required to maintain adequate oxygen delivery with VV bypass owing to the obligatory mixing of fully saturated blood returning from the ECMO circuit with desaturated systemic venous return within the right atrium. However, care must be taken to assure that flow is not too high such that the recirculation fraction is too high, which in turn will cause more poorly oxygenated blood to be delivered systemically.
Advantages of VV bypass include avoidance of carotid artery cannulation and maintenance of pulmonary blood flow with improvement in oxygen delivery. The major disadvantage is that, unlike VA bypass, VV ECMO does not provide cardiac support. Oxygen delivery in VV bypass remains dependent on native cardiac output.
Survival data, complication rate, and length of bypass support compare favorably with those of VA bypass cases. The ELSO Registry reports that more than 6700 newborns have undergone VV bypass with only 12% requiring conversion to venoarterial support.20 Because it avoids carotid ligation, VV bypass has enormous appeal and has become the treatment of choice in newborns with adequate cardiac function.
Personnel Needs
The most labor-intensive procedure in the neonatal intensive care unit (NICU) is ECMO. Specialists trained in managing patients on ECMO must remain at the patient’s bedside for the duration of bypass. In addition to monitoring the infant’s respiratory status, specialists must regulate the rates of blood and gas flow through the extracorporeal circuit to meet the infant’s metabolic and respiratory demands. They must adjust anticoagulation by frequently measuring the activated clotting time or antifactor Xa assays in the blood every and titrate the heparin infusion accordingly. Additionally, they must evaluate the patient for bleeding and replace losses appropriately. The specialists can be physicians, nurses, perfusionists, or respiratory therapists who have completed extensive training in perfusion support.
An ECMO physician trained in the clinical management of bypass patients must always be readily available for consultation, especially in case of mechanical failure or acute clinical decompensation. Additional support services required include 24-hour availability of personnel trained in radiology (ultrasonography), pediatric surgery, neurology, genetics, cardiology, and cardiothoracic surgery. The expertise and personnel needed to support these patients are extensive and costly.
Criteria for Patient Selection
The cumulative experience of many ECMO centers has resulted in the establishment of criteria that are currently used to decide whether ECMO support is appropriate.75 These are described in Box 78-1.
Gestational Age of 34 Weeks or Older
Preterm newborns with respiratory failure carry a higher risk for intracranial hemorrhage (ICH) compared with term infants at baseline, and this risk is heightened with systemic heparinization required during ECMO support. Furthermore, changes in cerebral blood flow patterns associated with cardiopulmonary bypass can also place the immature brain at increased risk for bleeding.
In the early ECMO trials, premature infants less than 35 weeks’ gestation had an 89% incidence of spontaneous ICH associated with heparinization.6 Subsequent studies (all retrospective) revisiting this issue reported improvement in outcome but confirmed increasing rates of ICH as gestational age decreases, with the incidence of ICH approaching 50% at gestational ages younger than 34 weeks.75
ECMO management has changed with time, and technical refinements in bypass support have made ECMO possible for infants weighing as little as 1800 g. However, even with improvements in management, gestational age remains a strong predictor of ICH risk. In one study, postconceptional age (PCA) proved to be the highest predictor for ICH risk. In a retrospective study of 1524 neonates less than 37 weeks’ gestation treated with ECMO between 1992 and 2000, ICH developed in 26% of patients younger than 32 weeks’ PCA compared with 6% of patients with PCA of 38 weeks (p = .004).31 Neurodevelopmental outcome in surviving preterm infants treated with ECMO in the first 2 weeks of life has not been well studied. This, together with the higher morbidity and mortality among ECMO-treated preterm infants, forms the basis of the recommendation that ECMO support be reserved for term and late-preterm infants.
No Major Intracranial Hemorrhage
The catastrophic extension of ICH, along with the attendant neurologic sequelae, is the primary risk reported in the early series of Bartlett and associates.6 Patients with grade III or IV ICH should not be offered ECMO because it is likely to expand with exposure to anticoagulation, further augmenting poor long-term prognoses. Some centers have successfully managed infants with grade I or stable grade II intraventricular hemorrhage on bypass using a minimal heparin dosage to lessen bleeding complications and high ECMO flow rates to prevent clotting in the extracorporeal circuit.
Uncontrolled bleeding from surgical wounds, chest tubes, or other sites also worsens with heparin therapy and is a contraindication to ECMO. The septic infant is of concern in this regard because of the commonly associated coagulopathy. Although these infants have a higher risk of bleeding complications on ECMO, meticulous correction of their coagulopathy and careful heparin management have allowed them to be successfully treated.20
Absence of Complex Congenital Heart Disease
Infants in severe respiratory failure must have an echocardiogram to rule out congenital heart disease as the underlying cause for refractory hypoxemia. In some instances, the degree of hypoxemia is not easily explained based on the heart lesion alone (e.g., in a newborn with an atrioventricular canal complicated by meconium aspiration or sepsis). Use of ECMO can provide cardiovascular support to stabilize such a patient until the reversible component of the lung disease is no longer an issue, rendering the baby a more viable surgical candidate at some later date.
Similarly, an infant with suspected cyanotic congenital heart disease may present to an ECMO center with profound cyanosis and cardiogenic shock despite the use of prostaglandins and inotropes. Preoperative ECMO can stabilize such infants who are believed to have reparable cardiac defects, but who are deemed to be poor surgical candidates by virtue of their clinical instability. Both venovenous and venoarterial ECMO have been used preoperatively in infants with cyanotic congenital heart disease and cardiovascular instability.36,39 Indications for ECMO include arterial saturations of 60% or less accompanied by hypotension and metabolic acidosis unresponsive to mechanical ventilation and pharmacologic support with inotropes and vasodilators. For most infants presenting with isolated cyanotic congenital heart disease, however, prompt surgical intervention, not ECMO, is the obvious treatment of choice.
Less than 10 to 14 Days of Assisted Ventilation
Although ECMO can support cardiovascular function for days to weeks, it does not reverse serious pre-existing pulmonary damage. In early studies infants subjected to prolonged mechanical ventilation with high pressures and Fio2 before ECMO suffered extensive barotrauma and did not recover despite prolonged support (more than 2 weeks) on bypass.6 Severe bronchopulmonary dysplasia (BPD), or inability to wean from ECMO support, was the result. Infants who have recovered from BPD, however, are eligible for ECMO later in life. Survivors of BPD have been placed on ECMO in later infancy or toddlerhood for life-threatening respiratory infections with good survival results: 59 of 76 (78%) patients from the ELSO registry. However, during the same period, one center reported severe pulmonary and neurodevelopmental sequelae among four of seven survivors with BPD who required ECMO beyond the neonatal period.33
Reversible Lung Disease
Using reversible lung disease as a criterion to determine whether to use ECMO is intended to exclude infants with severe lung hypoplasia incompatible with life. Patients with marked renal dysplasia and prolonged oligohydramnios, large CDH presenting in extremis at birth, and hydrops fetalis fall into this category. However, infants with respiratory failure in all these categories have survived with ECMO support, making the judgment of irreversible lung disease in the newborn extremely problematic.
The Dilemma of Congenital Diaphragmatic Hernia
Congenital diaphragmatic hernia (see Chapter 74) is defined as an anatomic defect in the diaphragm that permits abdominal contents to herniate into the thoracic cavity. In some cases, the abdominal viscera are covered with a membranous sac. The incidence of CDH is 1 in 2500 live births.54 The type of hernia depends on the location of the diaphragmatic defect. The most common is the posterolateral hernia, also called the Bochdalek hernia, which accounts for ≈70% of CDH cases. The defect occurs more frequently on the left side of the diaphragm (85%) than on the right side (13%), whereas bilateral hernias occur rarely (2%). Other types of CDH include an anterior, Morgani type (≈27%) and a central septum-transverse type (≈2%-3%).42
In CDH, the fetal lungs have a reduced number of arterioles and conducting airways. There are fewer alveoli with thickened septae, resulting in markedly diminished alveolar airspace and gas exchange surface area. Vascular development parallels that of the airways. There are a reduced number of vessels, adventitial thickening, medial muscle hyperplasia, and peripheral extension of the muscular layer into the smaller intra-acinary arterioles, thus resulting in a fixed increase in pulmonary vascular resistance. Both lungs are affected, the ipsilateral one more than the contralateral one, and the hypoplasia is progressive beyond 30 weeks.30 After delivery, these morphologic changes compromise effective gas exchange, resulting in respiratory failure and pulmonary hypertension. Additionally, survivors suffer from variable degrees of pulmonary, gastrointestinal (gastroesophageal reflux and feeding problems), orthopedic (scoliosis, rib cage hypoplasia), and hearing and neurodevelopmental issues.
Infants in whom profound cyanosis develops, despite oxygen, mechanical ventilation, bowel decompression, and judicious volume and inotropic blood pressure support, make up the subset of patients with CDH for whom ECMO support is justified. As shown in Figure 78-1, CDH is the second most common diagnosis for which ECMO support is used in neonates. In the 2014 ELSO Registry (an international registry of ECMO cases), survival of newborns with CDH supported with ECMO (n = 7002) is 51%. This stands in marked contrast to the 94% survival achieved among infants with meconium aspiration treated with ECMO (n = 8517).20
Prenatal diagnosis of CDH is common, but up to one third of cases are missed on prenatal ultrasound.27 In a prospective, population-based study of congenital anomalies in the United Kingdom, only 63 of 100 cases of CDH were diagnosed prenatally between 1997 and 2000 out of 125,380 live births. By comparison, during the same time period the prenatal diagnosis rate for hypoplastic left heart syndrome was 88% and the detection rate for gastroschisis was 94%. The prenatal diagnosis rate for all registered anomalies reported improved significantly over each 4-year epoch recorded from 1985 through 2000. Of note, termination of pregnancy for all fetal malformations rose from 23 to 47 per 10,000 registered births between 1985 and 2000.69 In a retrospective study of 51 CDH cases recorded in the Auvergne Birth Defect Registry between 1992 and 2003, the prenatal detection rate of nonisolated CDH (detecting either the CDH or another major anomaly) was 73%, whereas the detection rate of isolated CDH was only 45% (p = .03). Prenatal diagnosis in the presence of associated anomalies (cardiac, renal, neurologic, and gastrointestinal) predicted poor outcome with survival of only 23%. Paradoxically, infants with isolated CDH who escaped prenatal detection had the highest survival rate (81%).26,28
The pathogenesis of CDH and associated pulmonary hypoplasia are slowly being unraveled in part owing to animal models. Nitrofen is a teratogenic herbicide (banned in the United States and United Kingdom since the 1980s) that produces CDH in rodents. When administered to pregnant dams between days 8 and 11 postconception, a high rate of CDH with associated pulmonary hypoplasia and pulmonary vascular anomalies occurs in the offspring, remarkably similar to the human malformation. However, the use of nitrofen has not yet been linked to human CDH.42
A possible link between the nitrofen mouse model and human CDH might be from a disturbance in the retinoic acid pathway. A defect in the retinoid signaling pathway, specifically, inhibition of retinal dehydrogenase-2 (Raldh2), has been proposed as the likely etiology of the embryonic disruption resulting in CDH.28,29,37,61 Retinoids play a central role during embryogenesis and lung development in particular. During early lung morphogenesis, retinoic acid promotes mesodermal proliferation and induces fibroblast growth factor expression in the foregut. Retinoic acid is decreased in nitrofen-induced hypoplastic lungs in rodents. Furthermore, coadministration of large doses of vitamin A (25,000 units) and nitrofen to pregnant rodent dams reduces the incidence of CDH by 15% to 30% and attenuates lung hypoplasia, suggesting that the teratogenic effect of nitrofen on the embryonic lung and diaphragm is mediated through suppression of the retinoic pathway. Right-sided diaphragmatic hernias are also seen in offspring of dams fed a vitamin A–deficient diet. The incidence of herniation is decreased when vitamin A is introduced into the diet at midgestation.29 Despite this convincing animal data, there are few data regarding retinol and human CDH. Currently, there is an ongoing international, multicenter study evaluating the retinoic acid status of CDH infants, their mothers, and age-matched controls.
To explain the severe respiratory failure at birth owing to pulmonary hypoplasia, a “dual hit hypothesis” has been proposed. Traditionally, it was thought that the pulmonary hypoplasia associated with CDH was caused by compression of the lungs by the herniated abdominal viscera during early development. However, as our understanding of the pathophysiology grew, especially from the data obtained from the nitrofen animal model, it has been demonstrated that pulmonary development has already been disturbed prior to diaphragm development and before compression from abdominal organs can occur. Thus, the dual hit hypothesis has emerged to explain the severe pulmonary hypoplasia often seen in this condition.43 The first insult affects both lungs before diaphragm development is complete. The second insult affects the growth of the ipsilateral lung due to compression of that lung by the herniated viscera. The origin of the diaphragmatic defect lies in the disruption of the mesenchymal substrata of the pleuroperitoneal folds, which form the scaffold for the eventual migration of muscular precursor cells into the nascent diaphragm. In human embryology, this would point to a defect as early as 4 to 5 weeks’ gestation, long before the formation of the “muscular” diaphragm.14,61 Human infants with CDH have a 40% to 60% risk for major nonpulmonary anomalies including serious cardiac, urogenital, musculoskeletal, central nervous system, and gastrointestinal anomalies, designated as CDH+. In some of these CDH+ infants, the constellation of anomalies suggests well-described genetic syndrome, the most frequent of which is Fryns syndrome, which has an autosomal recessive inheritance pattern.37 Another proportion of these CDH+ infants have been found to have chromosomal rearrangements including aneuploidy (trisomies 13, 18, 21, and Turner syndrome),37,72 segmental deletions, or duplications and rearrangements.72 Genetic analysis has identified a CDH critical region on chromosome 15q26, which codes for four genes involved in diaphragmatic morphogenesis.14 Microdeletions in this region have been described in several patients with nonisolated CDH and are associated with very high mortality. Other CDH “hot spots” include 1q41-q42, 3q22, 4p16, 8p23, 8q22, 11p13. Haploinsufficiency or decreased expression of one or more genes encoded in these regions may cause or predispose to the development of CDH.37,72
Recent advances in genetic diagnostic modalities along with the use of knockout mice have shed some light on specific genes that may be involved in the pathogenesis of CDH, including clues for both the diaphragmatic defect as well as the associated pulmonary hypoplasia. Several genes including Wilms tumor 1 (Wt-1) and members of the sonic hedgehog (Shh) pathway (Shh, Gli2, Gli3) have been implicated in CDH.45,46,52,63,67 Wt-1-, Gli2-, and Gli3-null mice have all been shown to have diaphragmatic defects. Shh has been demonstrated to be down-regulated in human hypoplastic lungs of CDH patients.77Another growth factor–related gene, platelet-derived growth factor (PDGFRα), was demonstrated to play a role in posterolateral diaphragm and lung development, but the mice knockouts demonstrate features of Fryns syndrome; thus PDGFRα may be involved in nonisolated CDH.7,76 As advances are made in molecular genetics, more candidate genes are being discovered.
For all the reasons discussed, amniocentesis for genetic analysis is recommended in all cases of CDH diagnosed antenatally. After birth, G-banded chromosomal analysis and/or array-based comparative genomic hybridization should be performed because this technique is useful for identifying small chromosomal deletions and duplications below the resolution of G-band chromosomal analysis.28,72
Infants delivered at or near term in tertiary care centers have seen improved outcomes with changes in postnatal management. Recent reports have quoted survival rates of 70% to 90% of infants with isolated CDH born at tertiary care centers. However, these rates do not take into account those fetuses who were terminated or died in utero. Among infants with isolated CDH identified in utero, liver herniation into the fetal chest and a lung area–to-head circumference ratio (LHR) less than 1.4 in the contralateral lung reflect an especially high-risk cohort.30 Both findings predict a marked degree of lung hypoplasia and high mortality risk. Fetal surgery intervention has been actively studied in this unique group of CDH patients. Using a novel tracheal occlusion technique, this pioneering procedure has been shown in animal models of CDH to induce often dramatic growth of the hypoplastic left lung.18
One of the first clinical randomized trials of fetal intervention for isolated CDH in the United States in the 1990s did not improve survival.32 Infants enrolled in this study between 22 and 28 weeks’ gestation all had liver herniation into the left chest and LHR less than 1.4, reflecting significant pulmonary hypoplasia. Eight of 11 (73%) infants treated with fetal surgery survived, compared with 10 of 13 (77%) infants receiving standard care at a tertiary care NICU with ECMO available. Moreover, preterm delivery (mean 31.8 weeks) was more common in the fetal intervention group as a consequence of the trial design, although rates of neonatal morbidity reportedly did not differ between the groups. This could suggest there was potential pulmonary benefit from tracheal occlusion, which was then counteracted by adverse effects of prematurity. At the same time, a nonrandomized prospective trial on open tracheal occlusion was performed at the Children’s Hospital of Philadelphia. The inclusion criteria were herniation of the liver into the chest and LHR predictive of 90% mortality at their center. The tracheal occlusion group had a 33% survival rate, but these infants had significant neurologic and pulmonary morbidities, leading to abandonment of this approach.24
The European fetal surgery group has made great strides with refinement of patient selection criteria as well as technical and medical management of patients who meet criteria for fetal surgery for CDH. Currently the surgical procedure is done under epidural anesthesia for the mother with fentanyl and pancuronium given to the fetus for anesthesia. Following anesthesia, a much smaller (3.3 mm versus 5 mm) catheter is introduced percutaneously into the uterus through which a 1.2 mm fetoscope is used to position and inflate a balloon between the vocal cords and carina of the fetus.17 Also, the group has changed its practice to a planned in utero balloon removal rather than the previous management of removal of the balloon at the time of delivery via an EXIT procedure.
The European group has published its experiences with 210 consecutive fetuses with isolated severe CDH (LHR <1, liver up or equivalent O : E LHR; see Chapter 74). With their techniques, the balloon is placed between 26 and 28 weeks’ gestation and subsequently removed between 34 and 36 weeks’ gestation unless complications necessitated unplanned removal. The median gestational age at delivery was 35.3 weeks (range 25 and to 41 weeks) with 30.9% delivered before 34 weeks. Overall survival to discharge home in patients treated with FETO was 49% in those patients with severe, isolated left-sided CDH who had a predicted survival of 24% with expectant management, and 35% survival for right-sided CDH, which compares to a predicted survival of 0% with expectant management (although this was a very small cohort of infants). Thus the investigators conclude that the FETO procedure improved survival, likely from improved pulmonary growth in utero. Unfortunately, there were still pregnancy complications, preterm premature rupture of membranes (PPROM) in particular. Rate of PPROM was 47%, but only 30% of their cohort delivered before 34 weeks and only 4 developed chorioamnionitis.41
Similar improved outcomes were seen in a small, randomized trial performed by Ruano and colleagues in Brazil in a center without access to ECMO. They report 50% survival in their FETO group compared with 5% in their control group. They also found that there was a lower incidence of severe pulmonary hypertension in the FETO group compared to controls (50% versus 86%) and suggested that this was likely due to increased lung and vascular growth.70
With the successes of these two groups, there is renewed excitement in the fetal intervention for CDH, and currently an international, multicenter, randomized control study is underway to evaluate not only FETO for severe CDH, but also for moderately severe CDH and effectiveness performing fetoscopic tracheal occlusion at later gestational ages (30-32 weeks); this is the Tracheal Occlusion To Accelerate Lung Growth Trial.11
The postnatal management of CDH has changed considerably in the past several years. For example, CDH is no longer treated as a surgical emergency. The timing of surgical repair is usually delayed until the patient’s condition is stabilized with regard to oxygenation, blood pressure, and acid-base status, sometimes for a period of several days. Two prospective studies of delayed versus immediate repair failed to show any survival advantage. Conversely, these studies did not show any adverse outcomes associated with delayed surgery.74 The CDH Study Group, a consortium of 62 international centers tracking the outcome of patients with CDH, reports that surgical repair is now accomplished at an average of 73 hours of life in newborns not requiring ECMO support.12
Surfactant treatment in CDH has been used in several tertiary care nurseries based on preliminary reports of surfactant deficiency in animal models of CDH and a few human case series, even at term gestation. However, a large retrospective study has failed to substantiate the efficacy of surfactant treatment in term and near-term CDH infants. Using the CDH Study Group Registry, investigators identified 448 neonates with isolated CDH who were 35 weeks’ or greater gestation and who were treated with ECMO within the first 7 days of life. One hundred fourteen infants received surfactant treatment, whereas 334 infants did not. Surfactant replacement did not provide significant benefit in the infants’ clinical course with respect to survival, length of intubation, or subsequent need for supplemental oxygen.15 In an autopsy study comparing ipsilateral lung samples from 16 preterm fetuses with CDH to age-matched controls who died of nonpulmonary disease, no differences were found in surfactant protein or phospholipid concentrations.10
Autopsy studies of CDH lungs confirm diminished lung volumes and show evidence of severe barotrauma even when ventilator exposure was relatively short. Wung and associates had previously established the efficacy of avoiding hyperventilation in infants with PPHN.84 Based on this experience, investigators at Morgan Stanley Children’s Hospital of New York Presbyterian first applied the technique of gentle ventilation to patients with CDH in the hope of avoiding barotrauma and preserving the integrity of the vulnerable hypoplastic lungs. Permissive hypercapnea, rather than hyperventilation, was the goal of this strategy, using low respiratory pressures (usually ≤25 cm H2O) to achieve adequate chest rise rather than a target CO2. Preductal saturations greater than 90% are the goals in oxygenation, but oxygen saturations between 80% and 89% are tolerated if the patient is otherwise stable. High-frequency oscillatory ventilation (HFOV) is used when the patient’s preductal oxygen saturation is less than 80% or the Pco2 is persistently greater than 65 torr. Postductal saturations are often lower. ECMO is used to support infants with isolated CDH whose condition cannot be stabilized with gentle ventilation.
Using this approach, Boloker and colleagues reported 76% (91 of 120) survival in a cohort of 120 consecutive infants with CDH treated with delayed surgery and gentle ventilation.9 Excluding 18 infants who were not offered surgery (lethal anomalies, overwhelming pulmonary hypoplasia, neurologic complications), 84% survived to discharge. ECMO was used in only 13.3% of infants.
Wilson and associates previously reported no significant improvement in CDH survival with the adoption of delayed surgery or ECMO at Boston Children’s Hospital.83 They subsequently adopted a treatment protocol emphasizing permissive hypercapnea, control of pulmonary hypertension, and judicious support of cardiac function. Using this strategy, 36 of 39 (93%) consecutive infants survived to discharge. Of particular interest was the survival in 8 of 10 (80%) infants with structural heart defects. Previously, few infants with serious congenital heart lesions and CDH had survived at Boston Children’s Hospital or elsewhere.
In fact, the true impact of ECMO on CDH survival remains unproved. Many small studies report improved survival with ECMO use, but these often use historical controls that tend to exaggerate treatment effect. The same is true for high-frequency ventilation studies in CDH. Of all therapeutic interventions used in the treatment of patients with CDH and hypoxic respiratory failure, only iNO has been studied in a prospective, randomized trial. Disappointingly, neither the mortality rate nor the need for ECMO was diminished in those infants receiving iNO in two studies involving 83 patients.13,22
Failure of Maximal Medical Therapy
Because ECMO is an invasive procedure, it is currently reserved for infants receiving optimal conventional therapy who meet criteria indicating a 60% to 80% or greater chance of dying. It is critical that these infants be identified quickly so that adjunctive therapies, such as surfactant, HFOV, and iNO, can be used to stabilize and hopefully reverse respiratory failure short of ECMO. For infants whose respiratory status cannot be stabilized, however, ECMO should be initiated without delay, certainly before the infant is moribund.
The nature of maximal conventional therapy for respiratory failure in the newborn varies considerably among tertiary care units around the world and remains the source of much debate and controversy. No randomized trials have addressed the efficacy of several modalities employed in the treatment of neonatal respiratory failure complicated by PPHN.82 These included muscle paralysis, hyperventilation to induce respiratory alkalosis, alkali therapy to promote metabolic alkalosis, and vasodilator therapy, including tolazoline (no longer commercially available), prostaglandins E and D, nitroprusside, and magnesium sulfate. The use of the aforementioned vasodilators has been limited by their unpredictable side effect of systemic hypotension.
Using high-frequency ventilation with the aim of decreasing ventilation-perfusion () mismatch and improving oxygenation has been successful in some series.12 Surfactant use has also been shown to decrease the need for ECMO in newborns with parenchymal disease (e.g., RDS, pneumonia, meconium aspiration) and respiratory failure.56 Inhaled NO is a pulmonary vasodilator that selectively lowers pulmonary vascular resistance without adversely affecting systemic blood pressure. In prospective randomized trials of iNO in newborns with PPHN (excluding CDH), iNO improved oxygenation in most infants and decreased the need for ECMO by 40%.23
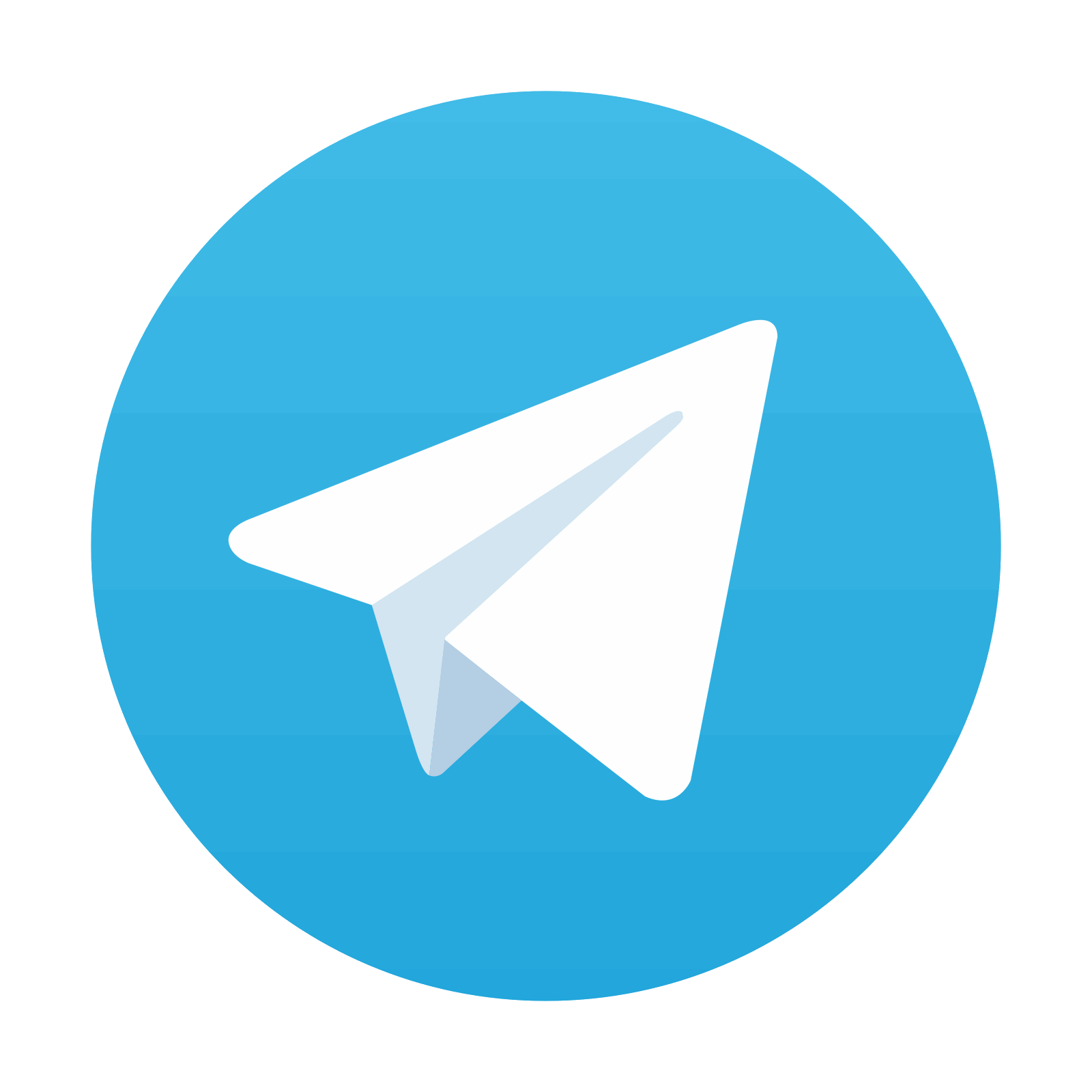
Stay updated, free articles. Join our Telegram channel

Full access? Get Clinical Tree
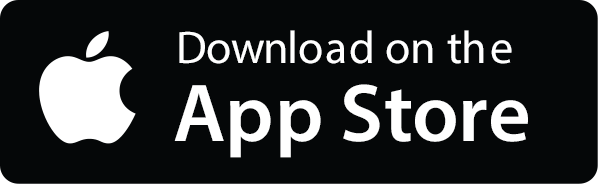
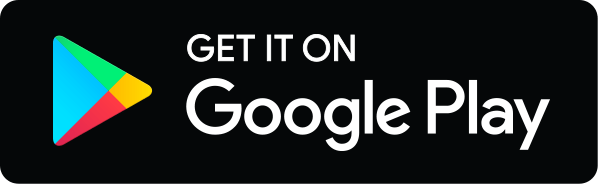