The Fetus as a Patient
Karl Sylvester
Craig T. Albanese
Surgery, Stanford University Medical Center, Lucile Packard Children’s Hospital, Stanford, California 94143-5733.
Surgery, Pediatrics, and Obstetrics and Gynecology, Division of Pediatric Surgery, Stanford University Medical Center, Lucile Packard Children’s Hospital, Stanford, California 94143–5733.
Since the mid-1980s, fetal surgery has evolved from a research endeavor into a multidisciplinary clinical enterprise of comprehensive maternal and fetal care. The recognition of the fetus as a patient grew out of advances in prenatal imaging. Prenatal ultrasound, widely applied in the past two decades, and more recently fetal magnetic resonance imaging (MRI) have allowed for a broader understanding of the natural history of many fetal anomalies. Improved diagnostic and anatomic descriptions allowed for the pathophysiology of fetal lesions to be serially studied and experimentally modeled. This experience produced a series of techniques for the management of potentially correctable life-threatening fetal malformations. More recently, a closer examination of the discourse between fetal pathophysiology, fetal therapy, and the resultant biologic outcomes of fetal intervention is redefining the future of fetal therapy.
During the 1980s, Harrison and colleagues at the University of California, San Francisco, (UCSF) pursued extensive studies in fetal lambs and rhesus monkeys in an attempt to model their ongoing observations on human fetal anatomic anomalies by ultrasound (US). As information on the pathophysiology of particular abnormalities was being gained, the efficacy of in utero correction for some maladies was shown in these animal models. Selection criteria for anomalies possibly amenable to fetal surgical correction were established for uniformly life-threatening and, frequently, otherwise hopeless malformations. By the 1990s, clinical implementation was well underway and there followed an eventual growth in fetal centers. These clinical programs in fetal surgery published numerous series of their experience evaluating the efficacy, safety, and cost of fetal surgery. The largely anecdotal and equivocal results have prompted several prospective clinical trials sponsored by the National Institutes of Health (NIH). These trials will critically evaluate the efficacy of fetal therapy against standard postnatal care.
PRENATAL DIAGNOSIS
More than any other variable, ultrasonography and its wide implementation has permitted the greatest gain in understanding of fetal malformations. Routine prenatal ultrasonography is currently generally performed between 16 and 20 weeks’ gestation as both a screen for congenital anomalies and, more broadly, for pregnancy dating and assaying fetal growth and well-being. From this experience, sonographic markers of fetal outcome and criteria for fetal intervention have been developed. Moreover, in many instances, this knowledge has allowed for more thorough informed counseling of women with pregnancies complicated by a fetal anomaly. Fetal echocardiography and pulsed Doppler interrogation of the fetal circulation provide valuable diagnostic information that often allows for a physiologic correlation to be derived for a recognized structural defect. In addition to its diagnostic utility, US is paramount to the success of any proposed fetal intervention. Guidance for percutaneous fetal interventions such as draining fetal effusions, fetal shunt placement, fetal vesicocentesis for obstructive uropathy, and the mapping of the placental position prior to open fetal surgery are just a few of the examples (1,2).
Out of a desire to gain ever more prease fetal anatomic detail, echo planar imaging or ultrafast MRI has emerged as an additional critical imaging modality (3). Complementary to fetal US, prenatal MRI has much more limited indications for its use, but can provide a more detailed assessment of fetal anatomy that translates into improved diagnostic accuracy in special circumstances (4). Ultrafast fetal MRI, which represents an evolution in technique to provide faster scan times, thus obviating the
interference caused by inevitable fetal movement, is currently most useful for improving diagnostic accuracy in evaluation of the fetal brain, spine, neck, chest, abdomen, and urinary tract (3). For example, in cases of fetal renal anomalies not infrequently accompanied by oligohydramnios, fetal MRI can better discern anatomic details lost to US as a result of the diminished acoustic window. Fetal MRI was first used in demonstrating liver herniation in right-sided congenital diaphragmatic hernia (CDH) or in distinguishing other congenital cystic lung anomalies such as congenital cystic adenomatoid malformation (CCAM) from CDH (5,6). Currently, there are no known harmful effects on developing human fetuses from the powerful magnetic field strengths used during fetal MRI (7). The usefulness of echo planar imaging prior to 18 weeks’ gestation remains limited due to overall fetal size, once again highlighting the role of ultrafast fetal MRI as an adjunct to prenatal US (3).
interference caused by inevitable fetal movement, is currently most useful for improving diagnostic accuracy in evaluation of the fetal brain, spine, neck, chest, abdomen, and urinary tract (3). For example, in cases of fetal renal anomalies not infrequently accompanied by oligohydramnios, fetal MRI can better discern anatomic details lost to US as a result of the diminished acoustic window. Fetal MRI was first used in demonstrating liver herniation in right-sided congenital diaphragmatic hernia (CDH) or in distinguishing other congenital cystic lung anomalies such as congenital cystic adenomatoid malformation (CCAM) from CDH (5,6). Currently, there are no known harmful effects on developing human fetuses from the powerful magnetic field strengths used during fetal MRI (7). The usefulness of echo planar imaging prior to 18 weeks’ gestation remains limited due to overall fetal size, once again highlighting the role of ultrafast fetal MRI as an adjunct to prenatal US (3).
Along with the advances in prenatal imaging since the mid-1980s, there have been parallel developments in the techniques of biochemical and chromosomal screening. Disease-specific molecular probes have become complementary to findings on prenatal imaging (2). Biochemical and genetic screening are now routine elements of the multidisciplinary evaluation of patients being considered for fetal intervention. Traditionally, only isolated defects unaccompanied by chromosomal abnormalities have been considered appropriate for fetal therapy. This may change as fetal therapy enters a new era of broader molecular diagnosis. Similar to the era of open fetal surgery, which followed a decade in which increased knowledge of prenatal disease and more accurate diagnostic capability developed, a not-too-distant future of possible genetic and molecular correction via in utero gene therapy and stem cell transplantation may follow current advances in molecular and cell biology (8,9,10).
A biochemical screen for several maternal serum markers such as α-fetoprotein (AFP) may indicate a pregnancy at increased risk for both structural and chromosomal abnormalities. The triple test, which combines assays for AFP, β-hCG, and unconjugated estriol in maternal serum, is one of the most useful assays for the risk assessment of fetal Down syndrome (trisomy 21) or other abnormal karyotypes (2). An abnormal result indicates that further targeted evaluation by US and genetic testing should be undertaken. Although the incidence of an abnormal karyotype depends on the specific malformation, certain lesions such as congenital cardiac defects, duodenal atresia, and cystic hygroma are recognized as having a significant incidence of aneuploidy. In addition, the combination of two or more structural anomalies significantly increases the likelihood of chromosomal abnormalities (2).
There are several alternative sampling techniques available for genetic analysis with specific indications for the use of each dependent on the indication for the genetic screen, the gestational age of the fetus, and the overall urgency for obtaining a useful result (11). Chorionic villous sampling (CVS) can be performed from 10 weeks’ gestation age until term, provides fetal tissue by biopsy of the placental villi, and is performed in fetuses at significant risk for a chromosomal disorder. A particular advantage of CVS is the rapid return of a result on chromosomal spread within 24 to 48 hours, allowing for quick decision making. Amniocentesis can also be performed in a fetus at risk for a chromosomal abnormality from 14 weeks’ gestation to term by the sampling of amniotic fluid. Results on chromosomal analysis from amniocentesis are not available for up to 14 days after the sampling, but additional information on lung maturity and AFP levels is available from amniotic fluid. An additional estimate of fetal lung maturity can be obtained by measuring the composition of amniotic fluid phospholipids via the lecithin/sphingomyelin ratio (12). Percutaneous umbilical blood sampling (PUBS) or cordocentesis provides fetal blood for rapid karyotyping and assessment of prenatal infections or congenital cellular disorders, such as hemoglobinopathies and metabolic disorders. An additional advantage of PUBS is the potential for therapeutic fetal intravenous therapy such as blood transfusion for hemolytic anemia and alloimmunization, or medications to treat fetal cardiac arrhythmias (2).
FETAL SURGERY: EVALUATION
Based on the knowledge gained from the advances in the aforementioned diagnostic modalities, perinatal management recommendations have been formulated for select fetuses with life-threatening anomalies (Table 3-1). More precisely, the natural history, pathophysiology, associated anomalies, and therapeutic options have become the basis for the multidisciplinary fetal treatment team, which has enabled the approach to the fetus as patient (13). Prenatal intervention has, in large part, been predicated on those anomalies that result in either low- or high-output cardiac failure resulting in hydrops (skin and/or nuchal edema or fluid accumulation in two of three body cavities (pleura, pericardium, peritoneum)]. Hydrops resulting from a specific lesion results in nearly 100% fetal mortality. Examples include fetuses with hydrops from a CCAM, sacrococcygeal teratoma (SCT), pericardial teratoma, select heart defects, tension hydrothorax, twin–twin transfusion syndrome (TTTS), and twin reversed arterial perfusion (TRAP) sequence. Pulmonary and kidney failure can be predicted in a subset of fetuses with urinary tract obstruction (e.g., posterior urethral valves) and may benefit from US-guided vesicoamniotic shunt placement. Select fetuses with threatened postnatal airway obstruction from a cervical teratoma or lymphangioma may benefit from the ex utero intrapartum treatment (EXIT) strategy in which a cesarean delivery approach is used, but
myometrial bleeding is controlled and the umbilical cord is not cut until an airway is obtained—by orotracheal intubation, tracheostomy, or mass resection followed by intubation or tracheostomy. Selective reduction for complications of monochorionic twinning has been used with increasing frequency given the low relative risk and ease of the procedure. Presently, a percutaneous, needle-based, US-guided approach using radiofrequency energy can perform this procedure under local anesthesia in under 5 minutes. Controversial areas of maternal–fetal intervention include nonlethal anomalies such as laser therapy for amniotic band syndrome and prenatal repair of a myelomeningocele. There are a host of nonlethal fetal anomalies whose outcomes are enhanced by prenatal diagnosis and counseling since the timing, mode, and venue of delivery are often important variables that directly affect postnatal outcome. Examples include hydrocephalus, gastroschisis, omphalocele, and urinary tract obstruction.
myometrial bleeding is controlled and the umbilical cord is not cut until an airway is obtained—by orotracheal intubation, tracheostomy, or mass resection followed by intubation or tracheostomy. Selective reduction for complications of monochorionic twinning has been used with increasing frequency given the low relative risk and ease of the procedure. Presently, a percutaneous, needle-based, US-guided approach using radiofrequency energy can perform this procedure under local anesthesia in under 5 minutes. Controversial areas of maternal–fetal intervention include nonlethal anomalies such as laser therapy for amniotic band syndrome and prenatal repair of a myelomeningocele. There are a host of nonlethal fetal anomalies whose outcomes are enhanced by prenatal diagnosis and counseling since the timing, mode, and venue of delivery are often important variables that directly affect postnatal outcome. Examples include hydrocephalus, gastroschisis, omphalocele, and urinary tract obstruction.
TABLE 3-1 Malformations that Interfere with Development and that May Benefit from Prenatal Therapy. | ||||||||||||||||||||||||||||||||||||||||||||||||||||||||||||||||||||||
---|---|---|---|---|---|---|---|---|---|---|---|---|---|---|---|---|---|---|---|---|---|---|---|---|---|---|---|---|---|---|---|---|---|---|---|---|---|---|---|---|---|---|---|---|---|---|---|---|---|---|---|---|---|---|---|---|---|---|---|---|---|---|---|---|---|---|---|---|---|---|
|
The prerequisites for consideration of maternal–fetal intervention for specific anomalies include (1) an accurate prenatal diagnosis; (2) the absence of severe associated anomalies; (3) a well-defined natural history; (4) the presence of a correctable lesion that, if uncorrected, will lead to fetal death or irreversible organ dysfunction before birth; and (5) technical feasibility of repair. Specific inclusion/exclusion criteria developed at multiple centers for maternal–fetal surgery are listed in Table 3-2.
Upon referral to a fetal treatment program, potential patients undergo an evaluation that includes a comprehensive ultrasonographic assessment of the identified lesion and a screen for associated anomalies. If indicated, fetal ultrafast MRI can be performed to gain greater anatomic detail for those lesions with persistent vagaries that may impact final recommendations. All fetuses are evaluated by echocardiography to rule out concomitant congenital cardiac disease, which might preclude any fetal intervention when in association with a second anomaly. All patients undergo genetic screening via one of the sampling techniques discussed previously. Findings of any chromosomal abnormalities, multiple structural anomalies, or a multiple gestation (other than twinning-associated anomalies) all preclude further consideration for fetal surgery (14,15). Finally, the maternal social support structure and other maternal comorbidities are heavily considered.
Risks and Benefits
For the fetus, the risk of the procedure is weighed against the benefit of correction of a lethal or debilitating defect. However, the risks and benefits for the mother are more
difficult to assess. Maternal safety is paramount because most fetal malformations do not directly threaten the mother’s health. However, she must bear significant risk and discomfort from the surgical procedure and the postoperative tocolytic therapy.
difficult to assess. Maternal safety is paramount because most fetal malformations do not directly threaten the mother’s health. However, she must bear significant risk and discomfort from the surgical procedure and the postoperative tocolytic therapy.
TABLE 3-2 Fetal Surgery Criteria. | |
---|---|
|
There have been no reported maternal deaths and few postoperative maternal complications, but considerable morbidity primarily related to preterm labor and its treatment (16,17). Maternal–fetal intervention, particularly fetoscopic or open hysterotomy procedures, carry the risk of short-term morbidity in the form of bleeding, wound infection, amniotic fluid leak, oligohydramnios, preterm labor, premature rupture of membranes, amniotic band syndrome, chorioamnionitis, placental abruption, complications of tocolytic therapy, deep venous thrombosis, and pulmonary embolism. Long-term risk has been more difficult to assess. After a hysterotomy that is not in a well-developed lower uterine segment (this includes virtually all the midgestation hysterotomy procedures), the risk of uterine rupture before and during labor is increased. This risk exists in both the current and subsequent pregnancies. Thus, to avoid uterine rupture, the index and all future pregnancies cannot include any period of labor and should be delivered by cesarean section. Furthermore, the risk of placenta previa following cesarean delivery is increased up to 15-fold over the baseline risk of 0.5%. The risk of placenta accreta in a subsequent pregnancy is greater with any prior uterine surgery, even in the absence of a placenta previa. The risk of infertility related to maternal–fetal intervention appears to be low, but an increased risk cannot be excluded. Since 1981, there are follow-up data from 35 mothers who have attempted pregnancy after fetal surgery; 32 conceived and delivered 30 normal children (18). Of the 3 who could not conceive, 2 had a strong prefetal surgery history of infertility and the third had only attempted for 6 months.
Finally, the psychosocial impact of maternal–fetal surgery has not been assessed adequately and needs to be evaluated in three groups of patients: (1) those that received counseling but elected not to have maternal–fetal surgery, (2) those that underwent maternal-fetal surgery with successful outcomes, and (3) those that underwent maternal–fetal surgery with unsuccessful outcomes (either death or poor quality of life).
Maternal safety has always been and continues to be a primary and overriding consideration.
FETAL SURGERY: TECHNIQUES
The fetal surgery operative team is also a multidisciplinary effort and includes two pediatric surgeons, a maternal–fetal medicine specialist with particular skill in obstetrical ultrasonography, an obstetric anesthesiologist, and possibly a neonatology resuscitation team at the ready, depending on the gestational timing of surgery and its indications. The operative steps of the entire procedure are performed by the lead pediatric surgeon with assistance from the others as necessary.
The mother is positioned with left uterine displacement to avoid inferior vena cava compression by the gravid uterus, and she and her baby are anesthetized with a halogenated agent. Maternal monitoring is accomplished with routine noninvasive monitoring. There are two surgical approaches to the fetus; one involves opening the uterus
(open hysterotomy) and delivering the fetal part to be repaired, the other employs minimal access techniques.
(open hysterotomy) and delivering the fetal part to be repaired, the other employs minimal access techniques.
Perioperative Management and Preterm Labor
Postoperatively, preterm labor occurs in 100% of patients, but to a varying degree (19,20). Indomethacin, magnesium sulfate, and terbutaline are the mainstays of postoperative tocolysis. Before discharge to home, maintenance tocolysis is instituted in the form of oral Nifedipine or a terbutaline pump.
Fetal surgery thus far has established a very safe maternal track record. With the everpresent threat of preterm labor, all patients experience postoperative uterine contractions. The mean time to delivery following fetal surgery has been reported to be 10 weeks at a mean gestational age of 34 weeks (15). Despite the effect of uterine manipulation on the likely release of vasoactive mediators, there have been few other predictable maternal morbidities (21).
THE CONGENITAL DIAPHRAGMATIC HERNIA EXPERIENCE
Of all the malformations treated by pediatric surgeons, CDH and its successful management remains one of the most recalcitrant problems. The physiologic problems related to CDH are conveyed by pulmonary hypoplasia. This is due largely to the developmental effects on lung function accompanying viscera herniation into the chest as a result of an absent diaphragm muscle (21). All aspects of the pulmonary parenchyma and pulmonary vascular tree development are abnormally arrested, which also predisposes to severe neonatal pulmonary hypertension. The severity of these deficits and their accompanying clinical manifestations are variable and believed to reflect several recognizable clinical patterns (22,23). The timing of herniation in either early or late gestation is believed to affect both the amount of herniated viscera and the timing of arrest in lung development (23). In general, early herniation produces severe pulmonary hypoplasia, whereas late herniation produces only a mild deficit that is successfully treated with standard postnatal care and surgery. Historically, without accurate stratification data, the mortality rate of CDH reported in retrospective studies failed to accurately define what has become recognized as the “hidden mortality” of CDH (24). It has been argued that overall neonatal mortality from CDH was actually higher than that traditionally reported in live born infants, given underreporting of in utero and perinatal fetal deaths from CDH (24,25).
A prospective study was undertaken to determine the outcome in a cohort of patients with prenatally diagnosed CDH referred to UCSF between 1989 and 1993 (25). Despite the nonrandomization of this select group, the study reported an overall 58% mortality rate and an additional need for extracorporeal membrane oxygenation (ECMO) support in 22 of 35 survivors. These findings drove the search for better stratification criteria to identify the most severely affected fetuses that might benefit from fetal therapy. Various potential prognostic factors were studied. Of the purely anatomic associations, only liver herniation (“liver up”) was shown to correlate with survival (26). The absence of liver herniation (“liver down”) portends a favorable survival prognosis of 93% in contradistinction to fetuses with liver herniation prenatally that demonstrate a mere 43% survival rate (25,27). More recently, a potentially better predictor of fetal survival has been proposed. This is the lung-to-head ratio (LHR) measured at 24 to 26 weeks’ gestation. This ratio is a measure of the right lung area measured in two dimensions at the level of the four-chamber view of the heart, divided by the head circumference to adjust for gestational age (28). The usefulness of the LHR as a predictor of outcome for fetuses diagnosed prenatally with CDH has subsequently been verified in studies published by independent groups (28,29). In one published prospective study, an LHR less than 1.0 was uniformly fatal. If greater than 1.4, uniform survival was found, and between 1.0 and 1.4, 38% of fetuses survived.
The overall mortality rate of CDH remains difficult to establish given the continued lack of a central reporting database and continued changes in treating persistent pulmonary hypertension of the newborn.
From the lessons learned in the fetal lamb model of CDH, the first attempts to correct fetal CDH in humans were designed to achieve anatomic correction (30,31,32). Anatomic repair in utero proved to be possible, but an extraordinarily challenging technical endeavor (32,33). The first 14 attempts at human in utero correction of CDH were undertaken in fetuses with an isolated left-side CDH diagnosed prior to 25 weeks’ gestation. Despite nine successful repairs, there were no long-term survivors because of difficulties including preterm labor, membrane disruption, premature delivery and subsequent fetal demise, and difficulty with herniated liver, all proved to be formidable hurdles. Technical improvements in approach and equipment like the uterine stapler allowed many of these shortcomings to be surmounted with great effort after careful study (33). This procedure ultimately resulted in satisfactory lung function in a few survivors; however, when compared with more traditional postnatal care in a clinical trial, there was no improvement in survival for fetuses with a more favorable prognosis by liver-down prenatal criteria (34). In addition, this experience demonstrated that simply correcting the anatomic defect and relieving the compression of the lung by herniated viscera was not sufficient to reverse the physiologic detriment of pulmonary hypoplasia that is manifest by an underdeveloped pulmonary parenchyma and vascular bed.
With a concerted effort directed at those fetuses with the worst prognostic signs of liver herniation and unfavorable LHR (less than 1.4), efforts were refocused on developing techniques for prenatal correction. A promising approach that seemingly addressed both the physiologic and anatomic detriment was the strategy of prenatal tracheal occlusion (35,36). This concept was based on observations of babies with congenital high airway obstruction syndrome (CHAOS) noting that fetal lungs that are obstructed in utero (usually by laryngeal atresia) undergo a dramatic hyperplasia response. This concept was adapted to a strategy of temporary prenatal tracheal obstruction, or “plug the lung until it grows” for CDH (37). Because the developing lung continually produces fluid that is circulated by fetal breathing movements into the amniotic fluid, a pressure gradient of fetal lung fluid is deemed necessary for normal fetal lung development. By blocking the normal egress of fetal lung fluid, a dramatic hyperplastic lung growth response results. In cases of CDH with herniated viscera in the chest, it was noted that fetal tracheal occlusion in the fetal lamb model caused marked enlargement of the hypoplastic lungs and concomitant reduction of herniated viscera (37). This approach was utilized in human fetuses with some early success (34,38,39). The earliest cases of tracheal occlusion (TO) for CDH demonstrated a variable biologic response of lung growth via a variety of methods. At least three approaches to TO have been attempted, including open fetal surgery for placement of a titanium clip and two minimal access fetal surgical (MAFS) approaches. For MAFS, two different strategies were tried, one endotracheal occlusion (first with a gelfoam polymer plug, then with a catheter-based detachable balloon), and the other was external occlusion using fetoscopically placed clips. Both the UCSF and Children’s Hospital of Pennsylvania (CHOP) groups reported their results of TO for CDH (40,41). CHOP reported their prospective trial using only the open fetal clip procedure in fetuses before 25 weeks’ gestation with poor prognostic indicators, including liver herniation and an LHR less than 1.0. Although good compensatory lung growth was achieved, overall survival remained dismal with only 5 of 13 surviving long term, three of whom have significant neurologic sequelae. Most failures were attributed to either ongoing respiratory compromise or lack of predictable biologic response in lung growth. This caused the CHOP investigators to question the issue of the timing of TO in order to achieve predictable results.
The UCSF program pioneered the minimal access clip and fetoscopic endotracheal balloon placement (41,42,43). A retrospective review of the UCSF experience compared minimal access clip with open tracheal occlusion and standard postnatal therapy (41). The results demonstrated that an endoscopic approach to tracheal occlusion effectively enlarged hypoplastic lungs, avoided some of the complications associated with the open approach, and improved lung function and overall survival. Survival in the minimal access cohort was 75%, compared with 38% with postnatal care alone (historical controls, however) and 15% in the open TO method (41). Selection criteria were different than the CHOP experience with eligible fetuses considered up to an LHR of 1.4, liver herniation present, and diagnosis prior to 25 weeks’ gestation. There was some crossover from minimal access to open clip for complicated cases, all of whom eventually succumbed, and this may account for the large discrepancy in these two outcomes. Despite improved technique with potentially less uterine trauma, problems with preterm labor, premature rupture of membranes, and membrane separation persisted after the minimal access clip procedure. Still, the results were compelling enough to serve as the basis for a prospective randomized trial sponsored by the NIH comparing MAFS to standard postnatal care for fetuses with liver herniation and an LHR less than 1.4 diagnosed prior to 25 weeks’ gestation.
The results of the UCSF randomized trial were reported in the New England Journal of Medicine in 2003 (44). The primary outcome variable was fetal/neonatal survival at 90 days postdelivery. Of the 28 eligible women carrying a fetus with a left-side CDH with liver herniation and an LHR less than 1.4; 24 were successfully enrolled between 23 and 27 weeks’ gestation. The data safety monitoring board stopped the trial early due to the unexpected overall survival of 77% in the group receiving standard postnatal care. Although the TO group also demonstrated a 73% survival at 90 days, the TO group delivered significantly earlier (mean 30.8 ± 2.0 weeks) than the group undergoing postnatal care alone (mean 37.0 ± 1.5 weeks).
Current Management Recommendations for Prenatally Diagnosed Congenital Diaphragmatic Hernia
After a patient is identified as carrying a fetus with a CDH, a comprehensive evaluation (Table 3-3) should be undertaken to render proper family counseling. Both advanced-level ultrasonography and fetal echocardiography should be performed to screen for associated anomalies. A genetic screen and chromosomal analysis should also be performed. Being armed with this information, which includes particular specific determination of the LHR and liver herniation status, allows fetuses to be stratified according to expected outcome. In addition, those fetuses whose CDH is diagnosed before 24 weeks’ gestation have a poorer prognosis compared with those diagnosed later. If the issues regarding TO for CDH outlined previously can be resolved satisfactorily, then perhaps therapy for liver up, LHR less than 0.9 fetuses will once again be offered. It is yet to be determined if the promise of the theoretical therapeutic benefit of fetal intervention for CDH will be realized (45).
TABLE 3-3 Evaluation of the Fetus with Congenital Diaphragmatic Hernia. | ||||||||||
---|---|---|---|---|---|---|---|---|---|---|
|
FETAL THORACIC LESIONS
CCAM and bronchopulmonary sequestration (BPS) are the most commonly identified and well-understood fetal lung masses. Prenatal diagnosis and serial US examinations have provided for new insight to the natural history and pathophysiology of fetal lung lesions. It has now been documented that large lesions can act as space-occupying lesions and compress adjacent normal structures. The secondary physiologic derangements (Table 3-1) that result can include pulmonary hypoplasia of normal lung tissue, polyhydramnios, fetal mediastinal compression, and cardiovascular compromise leading to fetal hydrops and death. The largest lung masses associated with hydrops are usually fatal, whereas smaller lesions can cause respiratory distress in the newborn period or be entirely asymptomatic unless infection occurs. There are subsets of cystic lung masses that have shrunk and others that have disappeared entirely prenatally.
The prenatal diagnosis of CCAM and BPS can be made by US. Historically, CCAMs were classified by Stocker according to size of the cysts from macrocystic to microcystic (47). Stocker type I cysts are macrocystic, Stocker type II are medium-size cysts, and type III are solid lesions. In an effort to link size to clinical behavior, Adzick, Harrison, and Glick redefined CCAMs based on more gross morphologic criteria and ultrasonographic characteristics (48). According to Adzick et al., macrocystic lesions contain either a single dominant or multiple cyst measuring greater than 5 mm in diameter or larger, and appear echogenic by US. Microcystic CCAMs contain cysts smaller than 5 mm, appear solid, and therefore echodense by prenatal US. Microcystic lesions tend to produce more mass effect, produce physiologic derangements, and therefore have a tendency for a worse prognosis. In general, the overall prognosis for fetal CCAM depends on the size and growth characteristics of the lesion.
Despite the overall greater prevalence of intralobar sequestration, it is rarely diagnosed prenatally. Extralobar sequestrations are much more commonly identified in the fetus and newborn, and may be associated with concomitant anomalies in as many as 50% of patients (46,49). BPS normally appears as a well-defined echodense mass by prenatal US most commonly at the base of the left chest. It can be difficult to distinguish between BPS and type II CCAM in particular. However, visualization of a feeding vessel from either the thoracic or proximal abdominal aorta strongly supports the diagnosis of BPS. There are no particular diagnostic signs of ILS on prenatal US. Other fetal thoracic masses that can be confused with BPS and CCAM include CDH, bronchogenic cyst, enteric cyst, mediastinal teratoma, hemangioma, neuroblastoma, congenital lobar emphysema, and bronchial atresia. Fetal MRI can be used to distinguish these various lesions when definitive diagnosis is in doubt (3,50). This can be important when one considers the natural history of each of these anomalies and its potential pathophysiologic sequelae.
When a fetal cystic lung mass compresses the heart and great vessels, low cardiac output nonimmune fetal hydrops can result (48,51). Polyhydramnios can also occur as a result of hydrops or due to compression of the fetal esophagus, impeding normal fetal swallowing of amniotic fluid. In support of this is the associated finding of absent stomach fluid in fetuses with large chest masses and mediastinal compression. If placentomegaly ensues (a finding with late or severe hydrops), the mother is at risk for preeclampsia.
In this circumstance, maternal physiologic condition can begin to resemble that of her compromised fetus in what is known as the “maternal mirror syndrome”(52,53). This is characterized by vomiting, hypertension, peripheral edema, proteinuria, and pulmonary edema. The underlying mechanism to these findings is believed to be the release of vasoactive mediators from the edematous placenta. This condition can develop quickly, almost always following the development of fetal hydrops, and is not specifically reversible by treating the underlying fetal anomaly alone. Only delivering the placenta cures this syndrome. Therefore, early recognition of a chest mass with the potential for producing hydrops is critical and requires frequent ultrasonographic surveilance.
In this circumstance, maternal physiologic condition can begin to resemble that of her compromised fetus in what is known as the “maternal mirror syndrome”(52,53). This is characterized by vomiting, hypertension, peripheral edema, proteinuria, and pulmonary edema. The underlying mechanism to these findings is believed to be the release of vasoactive mediators from the edematous placenta. This condition can develop quickly, almost always following the development of fetal hydrops, and is not specifically reversible by treating the underlying fetal anomaly alone. Only delivering the placenta cures this syndrome. Therefore, early recognition of a chest mass with the potential for producing hydrops is critical and requires frequent ultrasonographic surveilance.
Although both CCAM and BPS can produce hydrops, it is most commonly noted with a large microcystic CCAM that is diagnosed prior to 24 weeks’ gestation (14,48). Extralobar sequestration can produce hydrops either from mass effect (very rarely) or from a tension hydrothorax resulting from lymphatic secretion from the mass. Hydrops itself is believed to be a harbinger of fetal demise. The development of nonimmune fetal hydrops in the setting of a mediastinal or thoracic mass portends a near 100% mortality (48,54). In separate studies, Adzick et al. and Miller, Corteville, and Langer reported an incidence of fetal hydrops in 20% and 23%, respectively, of fetuses with CCAM (48,54). All of the hydropic fetuses in both of these series expired without attempts at fetal intervention. The resolution of fetal hydrops in association with a large chest lesion has been reported; however, this is currently believed to be the very rare exception (55,56). Given this strong association of chest mass with the development of hydrops and fetal demise, this is perhaps an appropriate clinical scenario for fetal therapy.
Faced with the known spectrum of pathophysiology, it is understandable that the natural history of fetal lung masses is therefore equally variable. Spontaneous regression of lung masses not accompanied by hydrops has been reported even late into pregnancy (48,57). Numerous studies have reported a spontaneous regression rate from 6% to 43% for CCAM and up to 75% for prenatally diagnosed BPS (48,51,57). Perhaps more startling are the few case reports of regression in cases of both CCAM and BPS associated with hydrops (50,55,56). One small series of hydrops regression was associated with prenatal steroid therapy (50). Mechanisms of regression remain unclear, and predictive criteria remain elusive. It is speculated that involution may occur with loss of blood supply or decompression into the neighboring tracheobronchial tree (14). Nonetheless, despite the recognition of spontaneously regressing lesions, complete resolution does not appear to occur because postnatal computed tomography (CT) imaging uniformly identifies a residual mass.
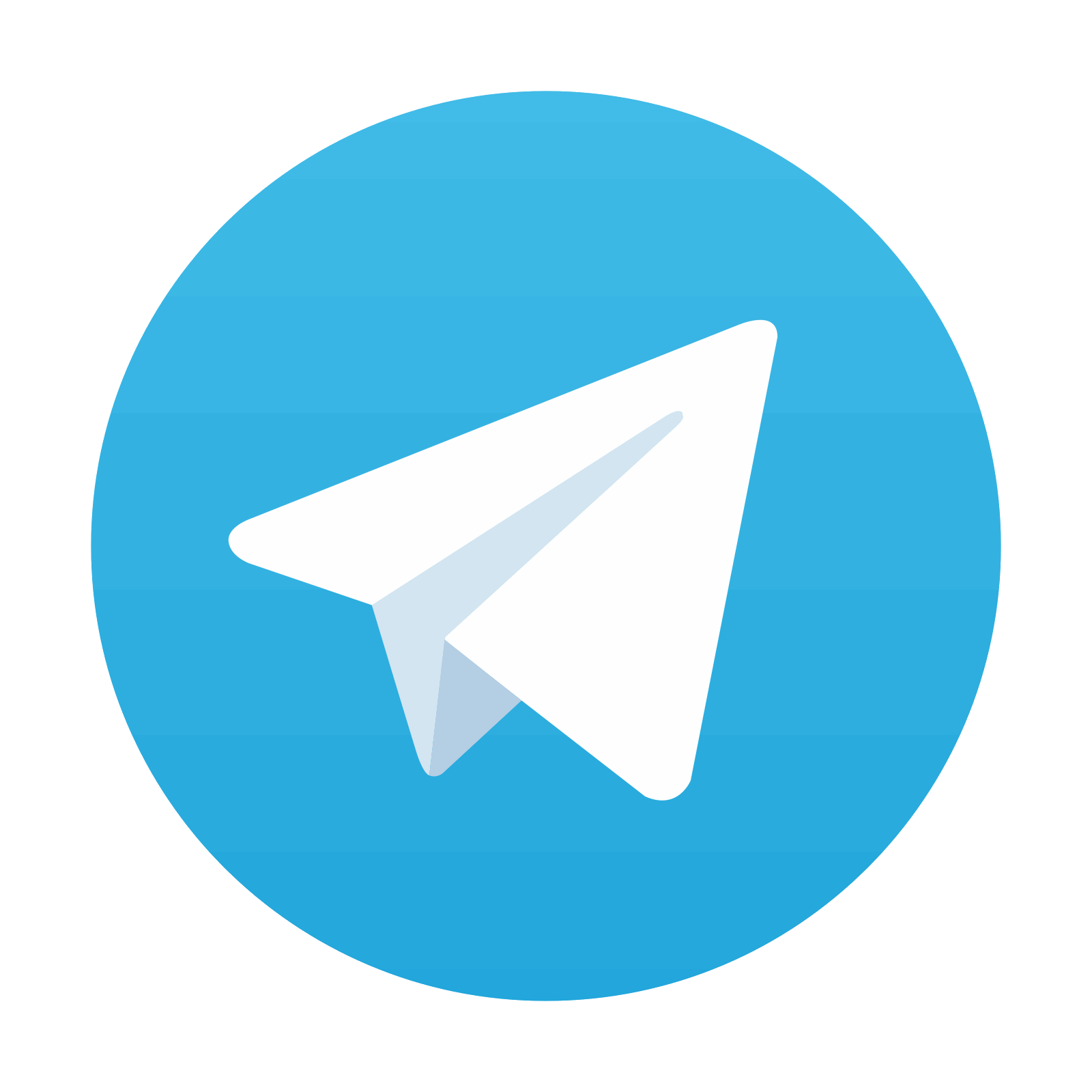
Stay updated, free articles. Join our Telegram channel

Full access? Get Clinical Tree
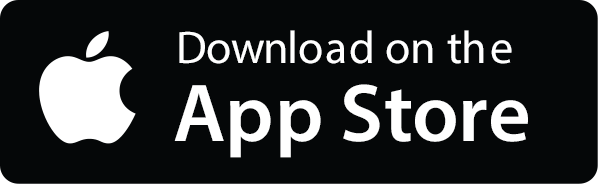
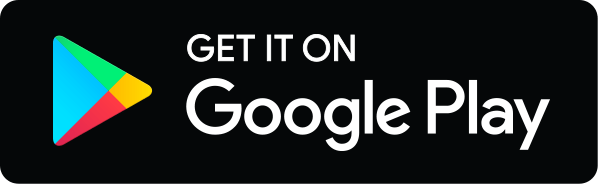