Refractory Hypoxemic Respiratory Failure
EPIDEMIOLOGY
Definitions and Incidence
Respiratory failure severe enough to require mechanical ventilation or other advanced respiratory support affects about 2% of all live births.1 In adult critical care, the term hypoxemic respiratory failure (HRF) defines the patient with respiratory failure and an arterial oxygen tension (PaO2) below 60 mm Hg. In neonatal intensive care, most babies with respiratory failure are preterm, while the term hypoxemic respiratory failure typically refers to the substantial subset of infants with respiratory failure that are term or near term. The term is often used interchangeably with persistent pulmonary hypertension of the newborn (PPHN), the clinical syndrome defined by the failure to achieve the normal neonatal drop in pulmonary vascular resistance (PVR) and increase in pulmonary blood flow and oxygenation. HRF or PPHN complicates a wide range of neonatal cardiopulmonary diseases and affects up to 10% of neonates who require neonatal intensive care unit (NICU) hospitalization. Timely recognition and therapy are important because of high associated rates of neonatal mortality as well as short- and long-term morbidities, including significant neurodevelopmental sequelae.
The incidence of PPHN is not well understood. An important study from the mid-1990s estimated its prevalence at 1.9/1000 live births,2 but only included infants referred to Level III NICUs in the United States; the majority of these infants were mechanically ventilated and receiving an FiO2 (fraction of inspired oxygen) greater than 0.9. More study is needed to better understand the incidence of more moderate disease, as well as the incidence of pulmonary arterial hypertension in mildly symptomatic or even asymptomatic babies. New national initiatives to perform routine pulse oximetry screening for critical congenital heart disease may provide valuable data to address the latter.3
General Risk Factors
A recent case-control surveillance study sought to determine risk factors for PPHN by interviewing mothers of infants who met criteria for PPHN and matched control subjects after discharge from the hospital.4 Maternal factors that were independently associated with an elevated risk for PPHN were black or Asian maternal race, elevated body mass index (BMI) (>27), diabetes, and asthma. Neonatal risk factors included male gender, delivery by cesarean section, infants large for gestational age, and delivery before 37 weeks’ gestation or after 41 weeks.
Genetic Risk Factors
Unlike pulmonary hypertension, which affects adults and older children, PPHN is rarely familial, and few genetic causes have been identified. A recent single-center report described the results of rigorous genotype analysis of 88 neonates with documented PPHN.5 No differences were noted in most candidate genes, including bone morphogenetic protein receptor type II (BMPR2) and nitric oxide synthase. However, a significant association was identified in the genes for corticotropin-releasing hormone receptor 1 (CRHR1) and CRH-binding protein. Significantly increased levels of 17-hydroxyprogesterone were also noted, adding weight to the hypothesis that genetic disorders of cortisol metabolism could contribute to PPHN. These data are supported by animal data indicating that antenatal and postnatal steroids reduce oxidant stress and normalize nitric oxide synthase and phosphodiesterase (PDE) function in experimental PPHN.6,7
Several studies have reported that children with Down syndrome (trisomy 21) have an elevated incidence of PPHN.8,9 In a Dutch cohort with excellent early ascertainment of Down syndrome, PPHN was documented in 5.2% of the infants.10 Infants with Down syndrome are also overrepresented in the registry maintained by the Extracorporeal Life Support Organization, and their survival to discharge is significantly decreased compared to the general population.11
Maternal Medications
The potential risk of maternal medications has also been examined for at least 2 commonly used agents. Maternal use of nonsteroidal anti-inflammatory drugs (NSAIDs) was identified as an independent risk factor in several studies.4,12 Upregulation of cyclooxygenases (COXs; both COX-1 and COX-2) in late gestation allows for increased production of prostacyclin (PGI2) at the time of birth,13,14 and maternal use of salicylates was 1 of the earliest triggers identified for PPHN.15,16 More recently, a single-center case-control study of 40 patients revealed a strong association between clinically significant PPHN and the presence of at least 1 NSAID (aspirin, ibuprofen, naproxen) in the meconium.17 At least 2 studies have indicated that NSAID levels correlate with the absence of a patent ductus arteriosus (PDA), suggesting that antenatal ductal constriction was a contributing factor.17,18
Selective serotonin reuptake inhibitors (SSRIs) are commonly used to treat maternal depression during pregnancy. In this case, conflicting evidence exists whether maternal SSRI use during pregnancy increases the risk of PPHN. In animal studies, newborn rats exposed in utero to fluoxetine developed pulmonary vascular remodeling and had clinical findings of abnormal oxygenation and higher mortality when compared with vehicle-treated controls.19 Brief infusions of sertraline and fluoxetine in fetal lambs directly increased PVR, and this elevation was sustained for at least 1 hour after the infusion was stopped.20 To date, 6 retrospective population-based studies have presented a range of findings: Three of the studies reported a positive association with adjusted odd ratios of 2.1 to 6.1, although little information is provided on the severity of PPHN.21–23 Three additional retrospective cohort studies did not find an association.24–26 In addition, other reviews have highlighted the challenges of distinguishing the impact of SSRI use from the impact of depression (including an increased risk of prematurity) on the risk for PPHN.27 The Food and Drug Administration (FDA) concluded (December 14, 2011) that the evidence is currently insufficient to conclude that SSRI use in pregnancy causes PPHN (Selective Serotonin Reuptake Inhibitor [SSRI] Antidepressant Use During Pregnancy and Reports of a Rare Heart and Lung Condition in Newborn Babies, http://www.fda.gov/Drugs/DrugSafety/ucm283375.htm) and currently recommends that health care professionals treat depression during pregnancy as clinically appropriate.
PATHOPHYSIOLOGY
General Categories of HRF
Table 26-1 outlines a broad range of diseases that can be associated with HRF or PPHN. Severe HRF usually presents as 1 of 3 types: (1) maladaptation, with constricted but structurally normal pulmonary vasculature, commonly associated with lung parenchymal diseases such as meconium aspiration syndrome, respiratory distress syndrome (RDS), or pneumonia; (2) excessive muscularization, in which the lung has normal parenchyma but remodeled pulmonary vasculature characterized by increased smooth muscle cell thickness and distal extension of muscle to vessels that are usually nonmuscular; or (3) the hypoplastic vasculature, associated with underdevelopment of the pulmonary vasculature, as seen in congenital diaphragmatic hernia (CDH). However, these designations are imprecise, and many patients have respiratory failure due to changes that overlap among these categories. For example, neonates with meconium aspiration typically have clinical evidence of altered vasoreactivity, but excessive muscularization is often found at autopsy. Neonates with CDH are primarily classified as having vascular hypoplasia, yet lung histology of fatal cases typically shows marked muscularization of pulmonary arteries, and clinically, these patients frequently respond to vasodilator therapy.
Table 26-1 Disorders Associated with Hypoxemic Respiratory Failure
Pulmonary
Idiopathic pulmonary hypertension
Meconium aspiration syndrome
Respiratory distress syndrome
Transient tachypnea of the newborn
Pneumonia/sepsis
Lung hypoplasia
Congenital diaphragmatic hernia
Associated abnormalities in lung development:
• Alveolar-capillary dysplasia
• Surfactant protein B deficiency
• ABCA3 deficiency
• Pulmonary lymphangiectasis
• Congenital lobar emphysema (rare association)
• Cystic adenomatoid malformation (rare association)
Cardiovascular
Myocardial dysfunction (asphyxia; infection; stress)
Structural cardiac diseases
• Mitral stenosis, cor triatriatum
• Endocardial fibroelastosis
• Pompe disease
• Aortic atresia, coarctation of the aorta, interrupted aortic arch
• Transposition of the great vessels
• Ebstein anomaly, tricuspid atresia
Pulmonary venous disease
• Pulmonary vein stenosis (isolated)
• Total anomalous pulmonary venous return
Pulmonary atresia
Hepatic arteriovenous malformations (AVMs)
Cerebral arteriovascular malformations
Associations with other diseases
Neuromuscular disease
Metabolic disease
Polycythemia
Thrombocytopenia
Maternal factors: nonsteroidal anti-inflammatory drug use, maternal diabetes or smoking
Pulmonary Vascular Remodeling
The first reports of PPHN described term newborns with profound hypoxemic pulmonary hypertension who lacked radiographic evidence of parenchymal lung disease. Idiopathic PPHN is sometimes called “black lung PPHN” because of the predominant vascular disease with little or no underlying parenchymal lung disease (Figure 26-1). It can cause profound hypoxemia due to shunting of blood through the extrapulmonary channels of the foramen ovale and ductus arteriosus. Autopsy studies of fatal PPHN suggest that severe hypertensive structural remodeling with vessel wall thickening and smooth muscle hyperplasia develops in utero, likely as a result of chronic intrauterine stress (Figure 26-2). In addition, the vascular smooth muscle extends to the level of the intra-acinar arteries, which does not normally occur until much later in postnatal lung development. Pulmonary vascular remodeling and extrapulmonary shunting can also contribute to the severity of disease in babies with meconium aspiration syndrome and other parenchymal lung diseases.
FIGURE 26-1 Typical chest radiograph of an infant with persistent pulmonary hypertension showing mild hyperexpansion and oligemic lung fields.
FIGURE 26-2 Histology of a pulmonary vessel from an infant who died of fatal hypoxemic respiratory failure (HRF); this illustrates the dramatic remodeling that can be associated with severe persistent pulmonary hypertension of the newborn.
Idiopathic PPHN is perhaps the best-studied form of neonatal pulmonary hypertension in the laboratory setting, and many groups have developed models to explain the fetal and neonatal pathophysiology that underlies the abnormal remodeling and vascular dysfunction of the fetal and early neonatal pulmonary vasculature. For instance, a well-known cause of idiopathic PPHN is constriction of the fetal ductus arteriosus in utero from exposure to NSAIDs during the third trimester. Subsequent studies developed a fetal lamb model in which the ductus is surgically closed in utero, which induces rapid increases in fetal pulmonary artery pressure, pulmonary vascular remodeling, and a subsequent failure to transition to extrauterine life.
Right ventricular dysfunction is a critically important component of severe PPHN and is a major contributor to poor outcomes.28 Although PVR is high during fetal life, the right ventricle pumps against the low-resistance placental circulation by directing most of its output through the ductus arteriosus. If transition occurs normally, the right ventricle continues to pump against a low-resistance circuit as PVR falls. On the other hand, if PPHN develops, the fetal right ventricle must rapidly adapt to a high-resistance circuit. Data from fetal lambs indicated that, when presented with elevated afterload,29 the fetal and neonatal right ventricle responded with a more dramatic reduction in stroke volume than the left ventricle, and this feature helps explain why right ventricular dysfunction is such an important determinant of outcome for HRF (Figure 26-3).
FIGURE 26-3 Hemodynamic changes in persistent pulmonary hypertension of the newborn/hypoxemic respiratory failure, demonstrating the interrelationship of cardiac, pulmonary, and vascular function. Surfactant deficiency (respiratory distress syndrome) or inactivation (meconium aspiration syndrome or pneumonia) produces parenchymal lung disease and ventilation-perfusion (V/Q) mismatch. Increased pulmonary vascular resistance results in reduced pulmonary blood flow and right-to-left shunt through the patent ductus arteriosus or patent foramen ovale. Pulmonary hypertension, often associated with systemic hypotension, results in septal deviation to the left. Left ventricular dysfunction secondary to asphyxia, sepsis, or congenital heart disease may contribute to pulmonary venous hypertension and complicate hypoxemic respiratory failure. (Used with permission of Satyan Lakshminrusimha.)
Parenchymal Lung Disease
Meconium Aspiration Syndrome
Approximately 13% of all live births are associated with meconium-stained fluid, although only 5% of these infants will go on to develop meconium aspiration syndrome. Intrauterine hypoxia and other stressful stimuli trigger passage meconium in utero. Aspiration of meconium either before or during delivery will obstruct small airways, cause severe pneumonitis, and induce inflammatory changes in the lung; all of these abnormalities will impair oxygenation after birth and acutely constrict the pulmonary vasculature. Hypertensive structural remodeling of small intra-acinar arteries is present in the most severe cases, most likely in response to chronic intrauterine hypoxia or lung injury.30 In support of this idea, another study found depressed expression of endothelial nitric oxide synthase in umbilical venous endothelial cells in infants who had severe PPHN in association with meconium-stained amniotic fluid.31 Meconium also induces postnatal release of pulmonary vasoconstrictors such as endothelin, thromboxane, and prostaglandin E2, all of which promote the development of pulmonary hypertension and proliferation of vascular smooth muscle that will lead to antenatal and postnatal vascular remodeling.32–34
Surfactant Deficiency
Surfactant deficiency (RDS) or inactivation (eg, from meconium aspiration syndrome or pneumonia) is a relatively common cause of HRF. Even subtle deficiencies of surfactant function or lung development may contribute to the development of HRF. Delivery prior to 39 weeks’ gestation is now appreciated to worsen neonatal outcomes, in large part because of an elevated incidence of neonatal respiratory failure.35,36 Furthermore, late-preterm and early-term infants are overrepresented in infants with the most severe respiratory distress who require extracorporeal membrane oxygenation (ECMO) support.37 Respiratory failure and alveolar hypoxia can trigger PPHN, adding further evidence that supports the ongoing national initiatives to reduce delivery prior to 39 weeks. When HRF is severe or does not resolve within the first week of life, an inherited disorder of surfactant synthesis should be considered.
Pulmonary Hypoplasia
Congenital Diaphragmatic Hernia
Congenital diaphragmatic hernia affects approximately 1 in 2000 to 3000 births and is the most common cause of pulmonary hypoplasia in the neonate. The majority are left-sided Bochdalek defects. CDH is characterized by a variable degree of pulmonary hypoplasia that reduces the alveolar surface area for gas exchange and may alter surfactant production or function. These epithelial abnormalities are accompanied by a decrease in the cross-sectional area of the pulmonary vasculature, increased muscularization of the intra-acinar pulmonary arteries, and in the most severe cases, left ventricular hypoplasia. Pulmonary capillary blood flow is decreased because of the small cross-sectional area of the pulmonary vascular bed, and flow may be further decreased by abnormal pulmonary vasoconstriction. Chromosomal abnormalities have been reported in as many as 30% of infants with CDH. CDH is a recognized finding in Cornelia de Lange syndrome, and as a prominent feature of Fryns syndrome, an autosomal recessive disorder with variable features, including diaphragmatic hernia, cleft lip and palate, and distal digital hypoplasia. Pallister-Killian syndrome (tetrasomy 12p mosaicism) presents with findings that are similar to those of Fryns syndrome, including coarse facial features, aortic stenosis, cardiac septal defects, and abnormal genitalia. Chromosome deletions on chromosomes 1q, 8p, and 15q have been reported in association with CDH, and deletions of chromosomes 8p and 15q appear to be associated with heart malformations.
DIFFERENTIAL DIAGNOSIS
The initial evaluation could also reveal whether rare lung abnormalities such as a congenital cystic adenomatoid malformation are causing cyanosis or pulmonary hypertension. Because infants with PPHN often present with cyanosis that is poorly responsive to oxygen therapy or mechanical ventilation, an early echocardiogram is essential to rule out congenital heart disease as a cause of fixed hypoxemia. Careful examination is required to ensure that there are no abnormalities of the pulmonary veins, and this component of the examination can be challenging in the presence of extensive right-to-left shunting across the ductus arteriosus.
Another diagnostic dilemma occurs in infants with severe refractory HRF that does not reverse despite optimal management. In these cases, the clinician must consider congenital abnormalities of surfactant synthesis or developmental lung defects such as lymphangiectasis or alveolar capillary dysplasia (ACD). This is particularly important for children with HRF that does not respond to ECMO.
Genetic Abnormalities of Surfactant Function
Genetic abnormalities of surfactant function were initially recognized in infants with PPHN who were refractory to inhaled nitric oxide (iNO) and ECMO. Surfactant protein B (SP-B) deficiency was the first to be reported and is characterized by early presentation, radiographic findings of severe RDS, and progressive respiratory failure and early death. The most common mutation is in codon 121 of the SP-B gene. Deficiencies in SP-C also occur, but PPHN is not a known association. Mutations in the adenosine triphosphate (ATP) binding cassette (ABC) transporter 3 gene are now recognized to occur in neonates with severe neonatal lung disease and symptoms of surfactant deficiency,38 are more common than SP-B deficiency, and have been reported to cause lethal PPHN.39
Alveolar Capillary Dysplasia
Alveolar capillary dysplasia with misalignment of the pulmonary veins is a rare form of interstitial lung disease that presents as severe pulmonary hypertension and hypoxemia early in life. It remains uniformly fatal despite treatment with all known modalities, including extracorporeal support.40 The etiology of ACD is not well understood, but the prevailing opinion is that an early antenatal injury or a genetic defect leads to insufficient development of the pulmonary capillary bed, followed by remodeling and muscularization of the pulmonary arterioles and the development of congested “misaligned pulmonary veins” residing in the same adventitial sheath. Approximately 10% of reported ACD cases have a familial association, indicating a probable genetic component. Mutations or deletions in the FOXF1 transcription factor gene or deletions upstream to FOXF1 are identified in up to 40% of infants with ACD.41
DIAGNOSTIC TESTING
Chest X-ray
Early evaluation with a chest x-ray is mandatory. The chest x-ray in classic idiopathic PPHN is oligemic, normally or slightly hyperinflated, and without parenchymal infiltrates (Figure 26-1). The chest x-ray will also evaluate for parenchymal lung disease, such as pneumonia, RDS, and meconium aspiration syndrome, as well as lung abnormalities such as CDH or cystic adenomatoid malformation.
Electrocardiogram
An electrocardiogram is occasionally performed but is not usually much help because a predominance of right-sided forces and moderate right ventricular hypertrophy are common and relatively nonspecific findings in newborn infants.
Echocardiography
The echocardiogram plays an essential diagnostic role and can serve as an important tool for the ongoing management of newborns with severe HRF. The initial echocardiographic evaluation rules out structural heart disease causing hypoxemia or ductal shunting (eg, coarctation of the aorta or total anomalous pulmonary venous return), determines the predominant direction of shunting at the patent foramen ovale and PDA, and assesses ventricular function. The echocardiogram will also help determine whether an infant with HRF has hypoxemia due to pulmonary hypertension. Although elevated pulmonary artery pressure is commonly found in association with neonatal lung disease, the diagnosis of PPHN is only established when there is evidence of bidirectional or predominantly right-to-left shunting across the foramen ovale or ductus arteriosus. Echocardiographic signs such as increased right ventricular systolic time intervals and septal flattening are suggestive, but less definitive, in establishing a diagnosis of pulmonary hypertension.
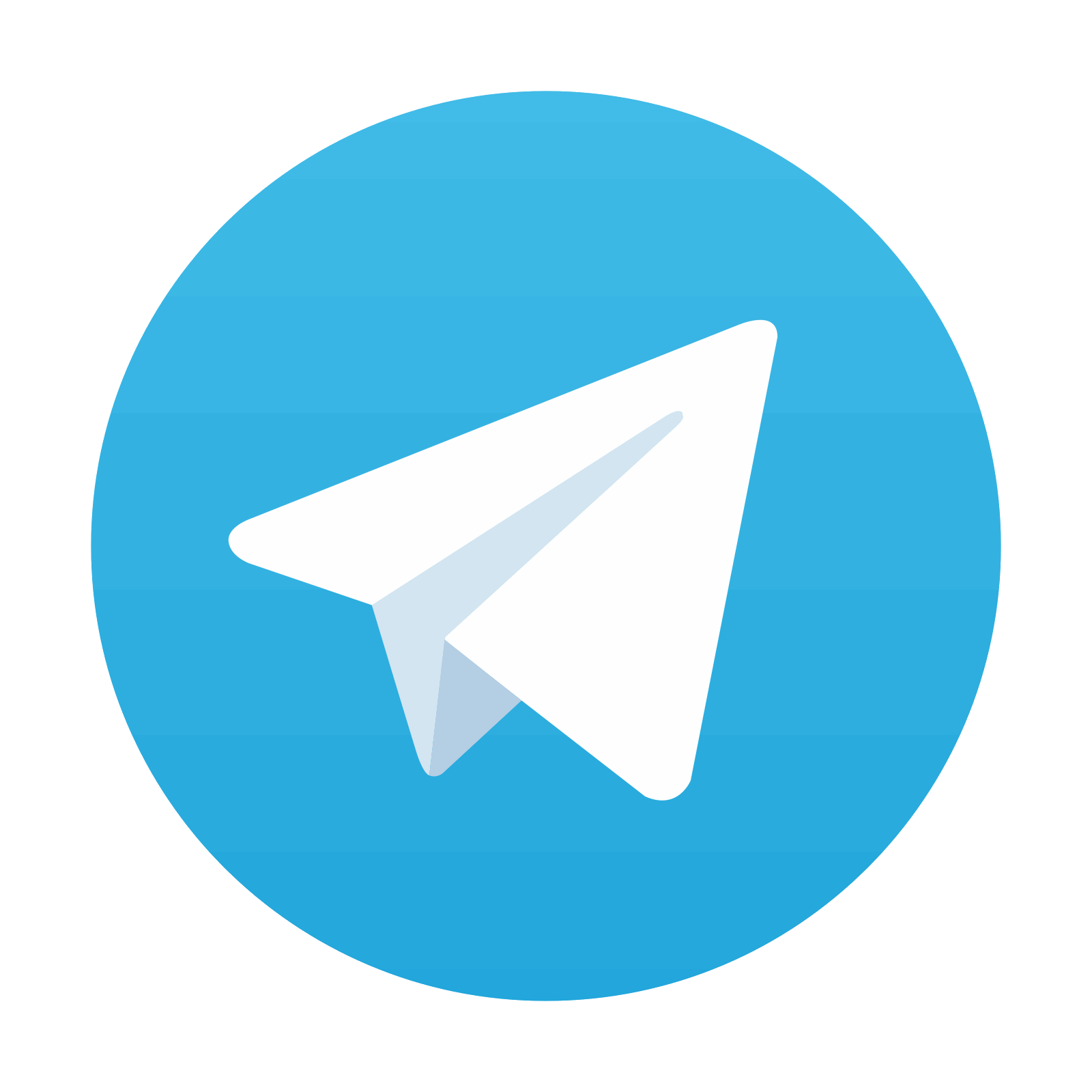
Stay updated, free articles. Join our Telegram channel

Full access? Get Clinical Tree
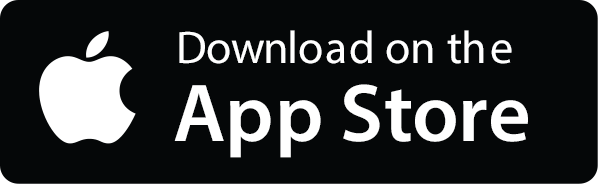
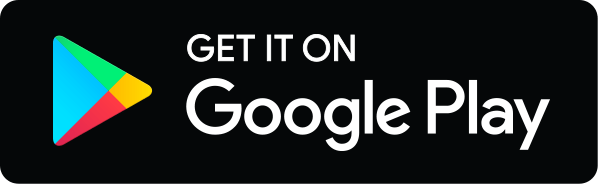