© Springer International Publishing Switzerland 2015
Howard J.A. Carp (ed.)Progestogens in Obstetrics and Gynecology10.1007/978-3-319-14385-9_1010. Progestogens and Breast Cancer
(1)
Gyneco-Endocrinology Unit, Rambam Health Campus, Technion School of Medicine, Haifa, Israel
1 Introduction
Progesterone plays a crucial role in the development of the breast alveolar tissue, and ductal branching.
When pregnancy takes place, the placenta produces progesterone in increasing amounts to support the pregnancy and prepare the breast for lactation. As a woman ages, the amount of progesterone produced by the corpus luteum slowly decreases. The clinical results are shortening of the luteal phase, with concomitant shortening of the menstrual cycle and increasing incidence of premenstrual syndrome (PMS) [1].
Progesterone and progestogens are have many uses in gynecology:- including treatment of menstrual disorders, combined oral contraception, and progesterone only contraception, hormone releasing IUD’s (LNG-IUS) PMS, prevention of premature uterine contractions, hormonal support of implantation after assisted reproduction, prevention of miscarrage etc [2].
The use of progestogens in menopausal hormone therapy (MHT) gained popularity after Ziel and Finkle’s [3] report showing that treatment with estrogen alone causes endometrial hyperplasia and cancer. However, these effects were completely counteracted by the addition of progestone to the estrogen replacement therapy. As hormone replacement therapy (HRT gained popularity, many synthetic forms of progestogen were introduced and are commonly used nowadays). These synthetic progestogens differ from one another in their structure, receptor affinity, metabolism and biological effects as described in other chapters.
The Women’s Health Initiative (WHI) study examined post menopausal hormone replacement therapy with conjugated equine estrogens (CEE) and medroxyprogesterone acetate (MPA). The study was stopped prematurely because of an increased risk of breast cancer (HR-1.26). However in women treated with CEE alone where MPA was not added due to previous hysterectomy there was no increase in the breast cancer risk compared to placebo (HR-0.80) [4]. The lower incidence of breast cancer was also seen in the WHI extended follow-up up study of 11.8 years [5]. Since the publication of the WHI trial there has been additional epidemiological data, all confirming that the addition of progestins to estrogen increases the risk of breast cancer when compared to estrogen treatment alone. Jick et al. [6] reported a case control study of 33,000 women. The reported odds ratio was not significant for developing invasive breast cancer in women using estrogen alone (OR 0.96 95 % CI 0.88–1.06) versus a significantly increased odds ratio of 1.44 (95 % CI 1.31–1.58); for those women using estrogen and progestogen. A meta-analysis [7] of four randomized trials published up to 2006, found a non significant RR of 0.79 (95 % CI = 0.61–1.02) for invasive breast cancer in estrogen only users and a statistically significant increased RR of 1.24 (95 % CI = 1.03–1.50) with estrogen and progestogen. Epidemiological studies have reported a non significant RR of 1.18 (95 % CI = 1.01–1.38) in estrogen only users, and a significantly increased RR of 1.70 (95 % CI = 1.36–2.17) with the use of estrogen plus progestin [7]. In view of the accumulated data cited above, some of the original authors of the WHI report have reiterated their original conclusions, stating that the use of “estrogen-alone treatment” reduces the breast cancer risk and does not substantially interfere with breast cancer detection by mammography [8].
As the molecular understanding of hormonal actions has expanded, with increased clarification of the different modes of action with specific co-activators and co-regulators when attached to their specific receptors, it became clear that not all the progestogens are the same. The French E3N Study comprised of 80,377 postmenopausal women followed up for more than 8 years, using different regimens of hormone therapy. The use of transdermal estrogen with a dehydroprogesterone combination was not associated with an increased risk of breast cancer. All other forms of estrogen progestogen combinations showed an increased breast cancer risk [9].
From a practical and clinical point of view, each progestogen has its own unique biological profile which may, or may not, be shared with other progestogens. The different actions of progestogens are fully described in Chap. 2 on the Pharmacology of the progestogens.
The effect of the normal menstrual cycle on the breast is well known. At the mid-luteal phase, corresponding to the peak level of luteal progesterone, mammary epithelial DNA synthesis and mitosis is at its highest. Hence, progesterone can be clearly seen to have a proliferative effect on the breast epithelium.
During pregnancy, placentally derived high levels of progesterone induce further lobular-alveolar development of the breast, in preparation for lactation. However, if high doses of progesterone are administered before breast surgery, there is less mitotic activity than after treatment with estrogen and progesterone. Therefore, estrogen and progesterone seem to act synergistically in the breast (and not antagonistically as in the endometrium). It has been shown that a single pulse of progesterone has growth stimulatory effect on cultured breast cells, but subsequent effects of further pulses of progesterone are growth inhibitors. There is an arrest of further cell cycles in the late G1stage. Several factors, such as cyclins D1 D3, decrease, or become inactivated by hypophosphorylation and inhibitory factors (such as kinase inhibitors p21, p27) which are induced by progestins. Hence, there is failure to induce further mitosis by frequent progesterone pulses [10].
2 Progesterone Receptors
The progesterone receptor is a single gene expressed in two isoforms: PR-A (94 kDa) and PR-B (116 kDa). There are homodimers (A-A), (B-B) and heterodimers (A-B) occurring naturally, in different proportions in different target tissues in the female body. They exhibit distinct transcriptional regulatory functions targeting various subsets of genes [11]. The inactivated receptor is activated by hormone (ligand) binding. This hormone-receptor complex translocates to the nucleus where it binds to specific DNA sequences in the promoter regions of target genes to activate gene expression. Alternatively, the expression of specific target genes can be repressed through interaction with various transcriptional factors such as nuclear factor kappa β (NFkβ). Consequently, the clinical significance of activation of the receptor is critically dependent on the transcriptional co-activators and repressors [12]. PR-A is mainly located in the nucleus. This receptor is required for uterine development. PR-B, which is essential for breast development, continuously shuttles between nuclear and cytoplasmatic compartments [13, 14]. Both receptors are co-expressed in the same tissues usually in equal ratios. In humans, normal mammary gland function may rely upon the balanced expression of the two PR isoforms. In breast cancer cells, however this ratio is often altered. An imbalance between the two isoforms appears to be linked to different cancerous phenotypes in the breast [15]. PR-A receptors are more stable and less active. PR-B receptors undergo extensive cross-talk with mitogenic protein kinases and are therefore heavily phosphorylated (more often via action of growth factors) [16]. The PRs can be phosphorylated (and therefore modified) after ligand treatment in response to local growth factors. Mitogenic protein kinase activity is very high in a cancerous environment. They have been shown to persistently phosphorylate, and thus modify PRs action, even in the absence of a ligand. This causes an inappropriate activation of PRs affecting PR modifying binding partners. Therefore PR action differs in normal breast tissue compared to neoplastic breast tissue. Additionally, the presence or absence of estrogen has a significant modifying effect on the PR. These effects are also organ specific, proliferating in the breast inhibitory in the endometrium. In summary, the PR has complex and versatile actions which are:—(1) different in normal and neoplastic tissue. (2) Tissue specific. (3) have different biological clinical actions regarding PR-A and PR-B. (4) May be ER dependent or ER independent. (5) Can act independently without binding ligand (progesterone). (6) different and specific for each progestogen. (7) different if given un a continuous or cyclic fashion. The above effects clearly indicate the varied effects that progestogens have on breast cancer cells biology, but the mechanisms are still not fully elucidated [17].
3 Breast Cancer: The Progesterone Effect
Breast cancer is the most common tumor in women. The life time risk of developing breast cancer is 12 %. Most of the neoplasms present after the menopause and the incidence rises sharply with age. Approximately 5–8 % of tumors arise due to a hereditary predisposition e:g, BRCA-1 mutation. Most breast cancers are initially hormone dependent with estrogen playing a crucial role in development and progression. Breast cancer may develop very slowly having a mean doubling time of 90 days. After a period that may last several years, many breast cancers become hormone independent [18]. Hormone independence may be due a mutation in the estrogen receptor.
Breast cancers are subdivided into two major subtypes:- 1.) Luminal, estrogen receptor positive (ER+), progesterone receptor positive (PR+), cytokeratin 18 positive (CK18+). These tumors make up approximately 80 % of all breast cancers. 2.) Basal ER-, PR-, CK5+. These tumors have a much worse clinical prognosis [19, 20]. In the human breast, normal stem cells are found. They can be defined by expression of epithelial specific antigen (ESA) and α6-integrin. Some believe that ER+ /PR+ luminal cancer cells arise from distinct ER+/PR+ stem cells [21]. However, in recent years it was discovered that in solid ER+/PR+ experimental tumors, CK5+ cells persist that are ER-/PR- stem cells that are actually up regulated by progestins [22]. These colonies, though small in size (up to 100 or less cells) expand and can differentiate into the more common ER+/PR+/CK5-,tumors. Approximately, 2 % of cells in these receptor positive breast cancers retain the ER-/PR-/CK5- stem like signature. [23]. Treatment of ER-/PR-/CK5+ colonies with progesterone, but not with estrogen, leads to an increase in the ER-/PR-/CK5+ stem cell subpopulation from 1 to 2 % to over 20 %. MPA (medroxy-progesterone acetate) has been found to have a more significant effect than other progestins. This increase is independent of estrogen. Hence, it seems that tumor cells can regress from a differentiated state to a more stem-like state, in response to progestins. [24]. Reactivation of the CK5+ cells (with strong CK18 expression, proving it to be of luminal origin) by 24 h of MPA exposure is usually more pronounced in the younger recently formed tumors.
It can be thus concluded that progestins, acting via their progesterone receptor affect the more abundant ER+/PR+/CK5 differentiated cells to reactivate ER-/PR-/CK5+ stem cells. It seems that progestogens have the ability to restore cancer stem cell like properties to some ER+/PR+ breast cancer cells. Only after this effect takes place, can estrogen resume growth and proliferation of the stem cells to expand ER+/PR+/CK5- breast tumors.
4 Local Production and Action of Progestins
The pharmacological properties of progestogens vary according to the parent molecule from which they are derived (testosterone, progesterone, aldosterone etc.) This, together with their ability to activate or deactivate related glucocorticoid receptors, mineralocorticoid receptors and androgen receptors is the reason for the different biological activities specific to each progestogen [25]. The enzyme, 5α-reductase, converts progesterone to 5α-pregnanes (e.g. 5α-dihydro-progesterone= 5α-P). 5α-reductase is highly expressed in cancerous cells but not in normal breast cells. 5α-P which is mainly produced by the cancerous cells stimulates cell proliferation and metastasis via activation of the MAP-kinase pathway [26]. In contrast, in normal breast cells, 5α-reductase activity is low. However, other enzymes, namely 3α-hydroxysteroid oxidoreductase and 20α-hydroxysteroid oxidoreductase metabolize progesterone to 3α-dihydroprogesterone (3α-HP) and 20α-dihydroprogesterone (20α-HP). These metabolites exert an anti-mitogenic effect and increase apoptosis and cell adhesion [27]. In tumor tissue, the concentration of mitogenic 5α-pregnane was 14 times that of the anti mitogenic 3α-HP. In contrast, in the adjacent normal breast cells, the concentration of antimitogenic 3α-HP was more than three times that of the mitogenic 5α-pregnane [26]. In view of the fact that 5α-P stimulates cell proliferation and metastasis, while 3α-HP and 20α-HP exert an antimitogenic effect, increasing apoptosis and cell adhesion, treatment with progesterone may stimulate cell growth only in the presence of malignant breast tissue [28].
Both ER/PR positive and ER/PR negative breast tumors are able to convert progesterone to 3α-HP. Specific high affinity membrane receptors exists for both 5α-P and 3α-HP. These receptors are completely distinct not only from each other but also from known ER, PR, AR and corticosteroid receptors [26]. Levels of 5α-P receptors are up-regulated by 5α-P and estradiol, and down-regulated by 3α-HP in both ER/PR positive and negative tumor cells [28]. 5α-P and 3α-HP have opposing effects on initiation and growth of ER/PR negative human breast tumors. These metabolites, which are independly produced by breast cancer cells, underscore the importance of the microenvironment in regulating expression of receptors, adhesion molecules, growth promoters and inhibitors within the breast cancer cells. 3α-HP maintains normal breast tissue. It inhibits proliferation, tumor initiation and tumor growth. 5α-P has exactly the opposite effects. It induces proliferation, tumor growth and detachment and reduces apoptosis.
5 Progesterone and Migration of Breast Cancer Cells
Death from breast cancer is usually due to invasion and metastasis to distant target organs (e.g. brain, bone lungs). Progesterone and progestins stimulate breast cancer migration and invasion [29]. The migration and invasion of cancer cells involve complex mechanisms. The first step is reorganization of the cells actin/myosin cytoskeleton. This reorganization enables the cells to develop membrane protrusion (called filopodia and lamellipodia). These protrusions are involved in the cell’s ability to detach itself from the main tumor body and, to enter lymphatic vessels and migrate. There are several 17β-estradiol up-regulating families of actin-binding protein which leads to this phenomena [30]. Studies comparing the specific effect of different synthetic progestins have demonstrated their individual and different effect on PR+ breast cancer cell migration and invasion in vitro. This activation is mediated in synergy with the activation of the actin-binding protein moesin to increase the formation of membranous structures. These specialized structures interact with the extra cellular matrix of nearby cells, allowing locomotion of the breast cells. [31]. It has been suggested that an in vitro “invasion index” for the effect of specific progestins on breast cancer cells lines incubated in vitro, with the addition of each progestin, with or without estrogen in the media can indicate the different and variable effects of progestins [32].
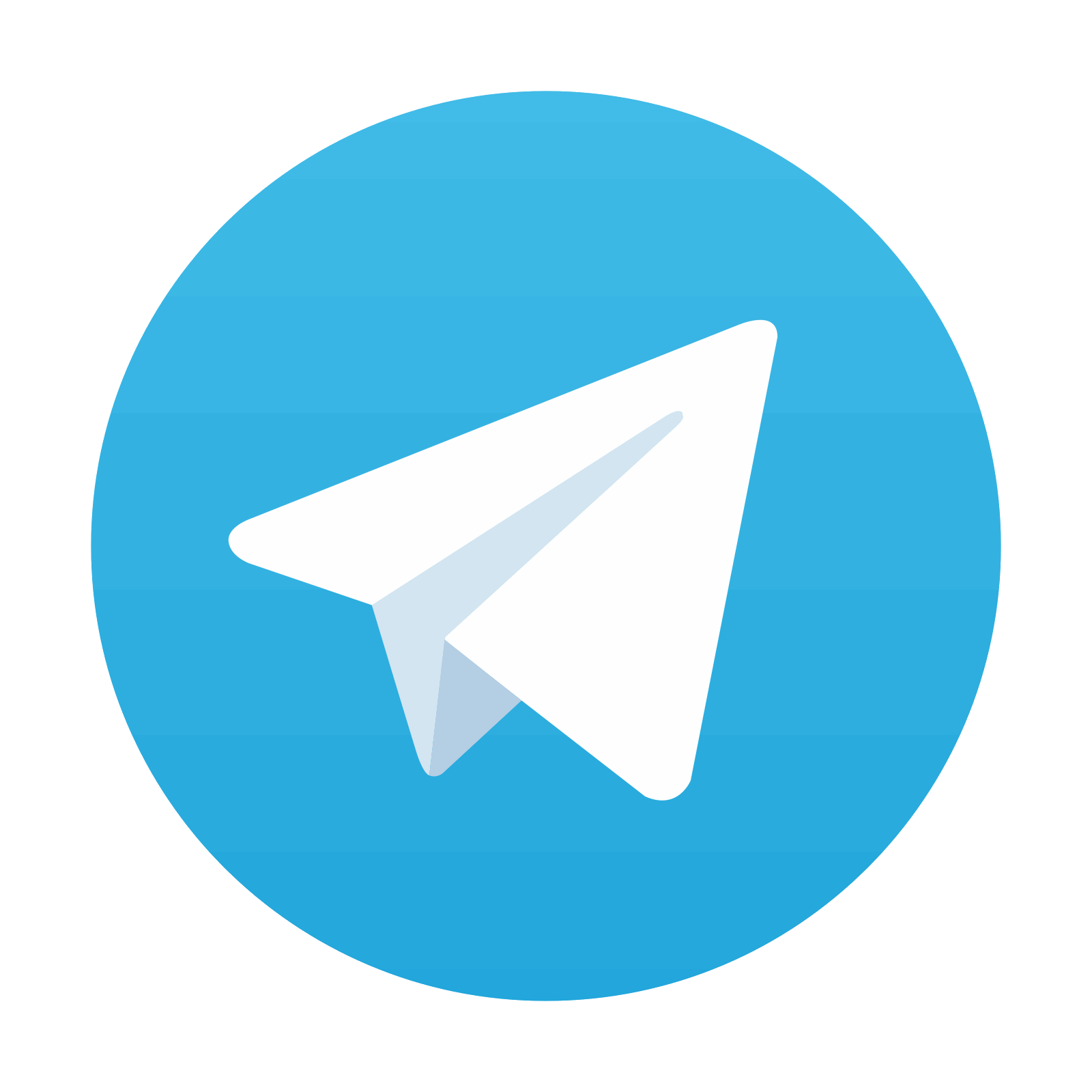
Stay updated, free articles. Join our Telegram channel

Full access? Get Clinical Tree
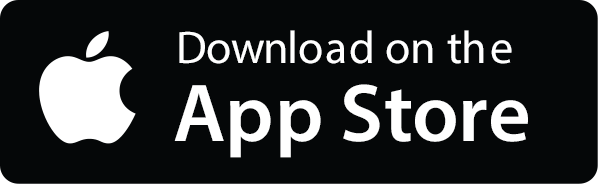
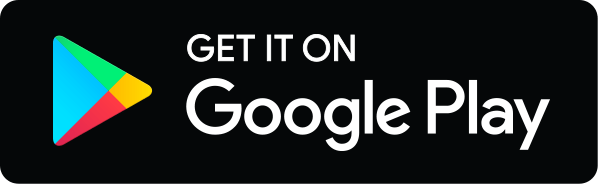