Principles of Drug Use in the Fetus and Neonate
Fetal Drug Disposition
The use of medications during pregnancy, prescription and over-the-counter, is a common and increasing occurrence. Multiple studies have demonstrated that approximately 90% of pregnant women take at least one medication during the pregnancy.17 The average number of medications used during pregnancy has increased by 68%, from 2.5 to 4.2, over the past 30 years, and the use of four or more medications has increased from 23% to 50% of pregnant women.17
Maternal Pharmacokinetics
Drug absorption is affected by a number of factors, including gastric emptying time and intestinal motility, which significantly change during pregnancy. Gastric emptying time is increased and intestinal motility is decreased during pregnancy, resulting in a reduced rate of absorption of many medications. The extent of oral absorption is not typically altered; therefore the steady-state concentration should not be impacted by maternal changes in absorption throughout pregnancy.
Steady-state concentration may be altered by physiologic changes that occur during pregnancy and that influence drug distribution (Tables 49-1 and 49-2). Maternal total body water, extracellular fluid, and plasma volume all increase gradually during pregnancy by about 32%, 36%, and 52%, respectively (see Table 49-2). Maternal cardiac output also increases by about 40% to 50% as a result of increases in both heart rate and stroke volume. The increase in body water is the primary reason for the observed decreases in peak drug concentrations with standard dosing of water-soluble drugs. When the drug dose is kept constant in the presence of increasing body water, the drug’s apparent volume of distribution (Vd) increases, and steady-state drug concentrations decrease. Such a decrease in steady-state concentrations may alter the therapeutic effect of the drug in the mother and influence the amount of drug available for diffusion across the placenta. Similarly, the increase in cardiac output affects drug transfer by limiting the time a drug resides in the villous space of the placenta and is available for transfer to the fetus.
TABLE 49-1
Influence of Pregnancy on the Physiologic Aspects of Drug Disposition
Pharmacokinetic Parameter by System | Change |
Absorption | |
Gastric emptying time | Increased |
Intestinal motility | Decreased |
Distribution | |
Plasma volume | Increased |
Total body water | Increased |
Cardiac output | Increased |
Plasma proteins | Decreased |
Body fat | Increased |
Metabolism | |
Hepatic metabolism | ± |
Extrahepatic metabolism | ± |
Excretion | |
Uterine blood flow | Increased |
Renal blood flow | Increased |
Glomerular filtration rate | Increased |
Modified from Mattison DR, et al. Physiologic adaptations to pregnancy: impact on pharmacokinetics. In: Yaffe SJ, et al, eds. Pediatric pharmacology: therapeutic principles in practice. Philadelphia: Saunders; 1992:81.
TABLE 49-2
Changes in Maternal Body Composition That Can Influence the Characteristics of Maternal Drug Distribution
Time of Gestation (wk) | Body Weight (kg) | Body Fat (%) | Plasma Volume (L) | Extracellular Fluid Volume (L) |
0 | 50.0 | 16.5 | 2.50 | 11 |
10 | 50.6 | 16.8 | 2.75 | 12 |
20 | 54.0 | 18.6 | 3.00 | 13 |
30 | 58.5 | 20.0 | 3.60 | 14 |
40 | 62.5 | 19.8 | 3.75 | 15 |
Data from Mattison DR, et al. Physiologic adaptations to pregnancy: impact on pharmacokinetics. In: Yaffe SJ, et al, eds. Pediatric pharmacology: therapeutic principles in practice. Philadelphia: Saunders; 1992:81.
Coincident with the changes in body water distribution and cardiac output, the amount of maternal fat increases at a relatively constant proportion to the increase in body weight (see Table 49-2). The result is an increase in Vd for lipophilic drugs and the potential for accumulation within the adipose tissue. Minimal changes in drug efficacy are expected under these circumstances; however, the adipose tissue can act as a reservoir and slowly release the drug into systemic circulation. An increased half-life and prolonged drug effects may be observed.
Complicating the picture even further is the fluctuation in serum proteins during gestation. Both albumin and α1-acid glycoprotein are decreased during pregnancy, resulting in an increase in the free (active) fraction of various highly protein-bound drugs. For example, the free fraction of tacrolimus may increase as much as 91% during mid and late pregnancy.14 This unbound drug is not only the active moiety, but is also the form that is available for metabolism and elimination by the mother and transfer to the fetus.
Changes in hepatic metabolism during pregnancy affect the normal rate and extent of drug transformation and ultimately renal elimination. During pregnancy, hepatic blood flow may increase, leading to increased drug metabolism in the liver; however, this is controversial. Less debated is the alteration in the activity of hepatic enzymes during pregnancy. Enzymes from the cytochrome P450 (CYP) family and those responsible for glucuronidation reactions (UGT) exhibit changes in activity as a result of pregnancy, which can potentially affect maternal and fetal drug exposure (Table 49-3). For example, labetalol is a commonly used antihypertensive agent in pregnant women, and it is extensively metabolized by UGT enzymes that have increased activity during pregnancy. Therefore, as expected, the clearance of labetalol after oral administration is increased during the second and third trimester by as much as 30%, and the half-life is significantly shorter in the pregnant compared with nonpregnant patient.3 The consequence for the mother is an increase in total daily dose to maintain the same steady-state concentration and well-controlled blood pressure, which is critical for both mother and fetus.
TABLE 49-3
Pregnancy-Induced Effects on Metabolizing Enzymes
Enzyme | First Trimester | Second Trimester | Third Trimester | Drugs Impacted |
CYP1A2 | Decreased 33% | Decreased 50% | Decreased 65% | Caffeine |
CYP2A6 | No data | Increased 54% | Increased 54% | Nicotine |
CYP2C9 | No change | No change | Increased 20% | Phenytoin |
CYP2D6 | No data | No data | Increased 50% | Dextromethorphan, fluoxetine |
CYP3A4 | No data | No data | Increased 50%-100% | Nifedipine, protease inhibitors |
UGT1A4 | Increased 200% | Increased 200% | Increased 300% | Lamotrigine |
UGT2B7 | No data | No data | Increased 50%-200% | Digoxin, enoxaparin |
Modified from Anderson GD, Carr DB. Effect of pregnancy on the pharmacokinetics of antihypertensive drugs. Clin Pharmacokinet. 2009;48:161.
Alterations in renal elimination during pregnancy also impact mother and fetus. These changes result from the increase in renal blood flow by about 60% to 80% and the increase in glomerular filtration rate by about 50%.8 As a result, there is enhanced elimination of drugs typically excreted unchanged in the urine (e.g., penicillin, digoxin). The lower steady-state concentrations that result are not often clinically significant for the mother, but may result in less drug exposure to the fetus.
Placental Handling of Drug
In addition to maternal pharmacokinetics, the placenta also plays a major role in determining fetal drug exposure. From the fourth week of gestation until term, the surface area of the placenta increases dramatically to accommodate the increasing needs of the maturing fetus. The surface area of the villi, which represents the real area for exchange, is about 3.4 m2 at 28 weeks’ gestation, compared with about 12.6 m2 at term. In contrast, the thickness of the placental membrane decreases with advancing gestation. During late gestation (about 32 weeks), the tissue barrier separating the maternal and fetal circulations may be less than 2 mm, and the areas become specialized in the transport functions of the placenta (i.e., rapid diffusion of substances) rather than the metabolic ones. By term, the placenta has only a single cell layer of fetal chorionic tissue separating the fetal capillary endothelium from the maternal blood. This separation, with its loose intercellular connections, presents little hindrance to small molecule transfer. Both the decreasing membrane thickness and increasing surface area with advancing gestation favor the greater transfer of drugs as gestation progresses.
Very little specific information defines the mechanisms of the processes and extent of drug transfer across the placenta. A number of interdependent variables influence the rate and extent of drug transfer across the placenta from the maternal to the fetal compartment. Most investigators and clinicians believe that the vast majority of compounds found in the maternal circulation will cross the placenta and enter the fetal compartment. Therefore, the fundamental questions are about the rate and extent of this activity. Unfortunately, even today this information is only partially available for just a few chemical entities. Similarly, the mechanisms by which drugs are transferred across the placenta are not always clear, and more than one type of transfer may be involved.
The possible modes of drug transfer across the placenta are listed in order of importance in Box 49-1. For most drugs and other compounds it is presumed that the primary mode of transfer is by simple, passive, nonionic diffusion, which requires no energy. Pinocytosis and phagocytosis require energy and likely occur much too slowly to have any significant impact on drug transfer, whereas facilitated and active transport play a role for some drugs.
Corticosteroids are an example of a drug class that undergoes facilitated diffusion. No energy is required to transfer steroids across the placenta, but the steroid must form a complex with a specific carrier on the placenta to be transferred from maternal to fetal circulation. Active transport occurs in a similar way, but energy is required for active transport to take place. Valproic acid is thought to be at least partially transferred through the active transport mechanism.30 Another important aspect of active transport involves the efflux of drugs back to maternal circulation subsequent to binding with a carrier on the placenta. Digoxin is one such drug that binds to the carrier protein Pgp.15 Pgp is expressed on the maternal side of the placental-trophoblastic layer, where it mediates active efflux from the fetal compartment. This efflux is unidirectional from the fetal to maternal circulation and is probably responsible for the removal of a number of highly lipid-soluble compounds and drugs from the fetal compartment.35
where K is the diffusion constant of the drug, which is dependent on the drug’s physicochemical characteristics; A is the surface area of the membrane to be traversed; Cm and Cf are the concentrations of the drug in maternal and fetal blood, respectively; and d is the thickness of the membrane to be traversed. Thus Cm − Cf represents the concentration gradient across the placenta, which is primarily regulated by the surface area (A) and thickness (d) of the placenta. As previously mentioned, changes in these characteristics as pregnancy progresses allow for easier transfer of drugs from maternal to fetal circulation.
The important physicochemical characteristics that influence passive drug transfer across membranes (i.e., placenta), as represented by K in the Fick equation, are outlined in Box 49-2. The characteristics that facilitate placental transfer include high lipid solubility, the un-ionized form under physiologic and pathophysiologic conditions, low molecular weight (<500 daltons), and low protein binding. Very few clinically important drugs meet all these “ideal” characteristics, which explains the high degree of variability reported in various studies of placental transfer.
Other factors important to drug transfer across the placenta include differences between the maternal and fetal osmotic pressures and pH, as well as changes in uterine or placental blood flow. Under normal circumstances, the maternal osmotic pressure is higher than that in the fetal compartment, and a pH difference of 0.1 to 0.15 unit exists between the fetal and maternal blood (i.e., fetal umbilical blood is −0.1 unit of pH lower than maternal blood). If alterations in either the osmotic pressures or pH occur, drug transfer will be affected. With regard to changes in blood flow, the placental flow increases from 50 mL per minute at 10 weeks to 600 mL per minute at term, whereas uterine blood flow approaches 150 mL/kg per minute of newborn body weight at term.30 Depending on the physicochemical characteristics of the drug, blood flow may have an important influence on the rate and amount of drug transfer.
The degree to which the placenta metabolizes a drug, if at all, also influences the amount that is estimated to cross the placenta and the amount of active drug that reaches the fetus. Many cytochrome P450 (CYP) mixed-function oxidase enzymes have been isolated from the human placenta.35 Most of these enzymes appear to be located in the smooth endoplasmic reticulum and mitochondria of trophoblasts. Although each enzyme possesses its own substrate specificity, substantial overlap exists in the metabolizing capacity of these enzymes. It is clear that a number of these enzymes are also susceptible to modulation (i.e., induction or inhibition) by exogenous influences. For example, CYP1A activity is increased with cigarette smoking during pregnancy. In addition to mixed-function oxidase activity, the human placenta also possesses some capacity to catalyze phase II conjugation reactions, which enhance water solubility of the affected drugs and ultimately drug transfer.
The number of different substrates biotransformed by enzymes located in the placenta is large, but the actual content of CYP enzymes in placental tissue is low, and the activity appears to be much lower than that determined in the fetal or adult liver. Thus, the actual contribution of placental enzyme activity to the biotransformation of administered drugs is believed to be inconsequential and of limited clinical significance for most drugs administered maternally. Nevertheless, the differential placental metabolism of certain corticosteroids (e.g., prednisolone, in contrast to betamethasone)5a and the resulting poor clinical outcomes with prednisolone compared with betamethasone in enhancing fetal lung maturation suggest a significant effect of placental metabolism in certain clinical scenarios.
Fetal Pharmacokinetics
Contrary to the popular belief that the fetus resides in a privileged and protected environment, it is clear that it is exposed to virtually every chemical entity to which the mother is exposed. Thus the absorption of most drugs into the fetal circulation is both rapid and complete. Most of this absorption is believed to occur through passive nonionic diffusion down the concentration gradients between maternal and fetal blood (which oppose each other in the placental villi), where they are separated by only a single layer of cells. However, as described earlier, a number of energy-dependent placental transporters have been characterized and are responsible for drug influx and efflux.35 In addition, the fetus may undergo continued drug exposure after its elimination because of the recirculation of amniotic fluid through the fetal gastrointestinal tract.
Once a drug is within the fetal circulation, we have little knowledge of its fate or potential activity. It will likely be distributed to the fetal organs in a manner similar to that observed after birth. However, it is not known whether there are any unusual fetal barriers to drug distribution analogous to those of the blood-brain barrier or the anterior chamber of the eye. In fact, it is not even known whether these barriers actually exist during fetal life. Additionally, a drug may have an increased affinity for a specific fetal tissue that is not the typical target tissue. For example, tetracycline has a high affinity for fetal teeth and warfarin for fetal bones.5 Protein binding will also affect drug distribution. The fetus has lower levels of serum proteins, and the proteins that are present have a lower affinity for binding drugs. The serum protein levels increase with gestational age, but free drug levels remain high secondary to the binding affinity of fetal proteins.
There is no question that the mean residence time for drugs in the fetus is longer than that observed in older children and adults because of the immaturity of drug clearance mechanisms. For drugs undergoing significant hepatic metabolism, there are many unknown factors. Although most hepatic metabolic pathways are less active in the fetus than in the adult, this is not universally true. Moreover, in the fetus, the adrenal gland has a significant complement of drug-metabolizing enzymes that, although active during fetal and neonatal life, disappear by 6 months of postnatal age. Thus the true ability of the fetus to metabolize drugs is largely unknown, but it at least partially depends on the particular drug under consideration, the gestational age of the fetus, and its drug metabolism phenotype.
As noted, multiple enzyme systems are involved in xenobiotic metabolism within the body. Of the phase I or oxidative enzymes, cytochrome P450 predominates. These CYP enzymes constitute a superfamily of heme-containing monooxygenases, with a minimum of 14 CYP gene families appearing to exist in mammals. The CYP1, CYP2, and CYP3 families are the most important in human drug metabolism, with the largest number of therapeutic agents undergoing CYP metabolism by the 3A family. Although the fetuses of many species appear to possess a very poor capacity to metabolize xenobiotics, the liver of the human fetus is relatively well developed in its capacity to metabolize various compounds, and it appears to mirror those processes performed by the placenta.2 Nevertheless, the overall functional capacity of fetal CYP activity is very low, and its metabolic capacity is qualitatively and quantitatively very different from the activity observed in the adult liver. The human fetal liver contains many different CYP forms (Table 49-4), although they are present in fewer numbers and with less density than in the adult liver. The enzyme content may also be impacted by maternal factors such as obesity, which has been shown to decrease CYP1A1 activity in the fetal liver.10 In general, the total amount of CYP in the fetal liver approximates 0.2 to 0.4 nmol/mg of microsomal protein, which represents about 20% to 70% of that found in the adult liver.2
TABLE 49-4
Expression of Cytochrome P450 Forms in the Fetal Liver and the Adrenal Gland
Organ/Gland* | CYP Form |
Liver† | 1A, 2D6, 3A, 3A5, 3A7† |
Adrenal† | 1A1, 2A5, 3A,† 3A7, B1, 17 |
Questionable expression | 2C, E1 |
*Primary form/isoform expressed at specific site.
†Primary site for CYP expression in the human fetus (fetal adrenal > liver containing CYP protein).
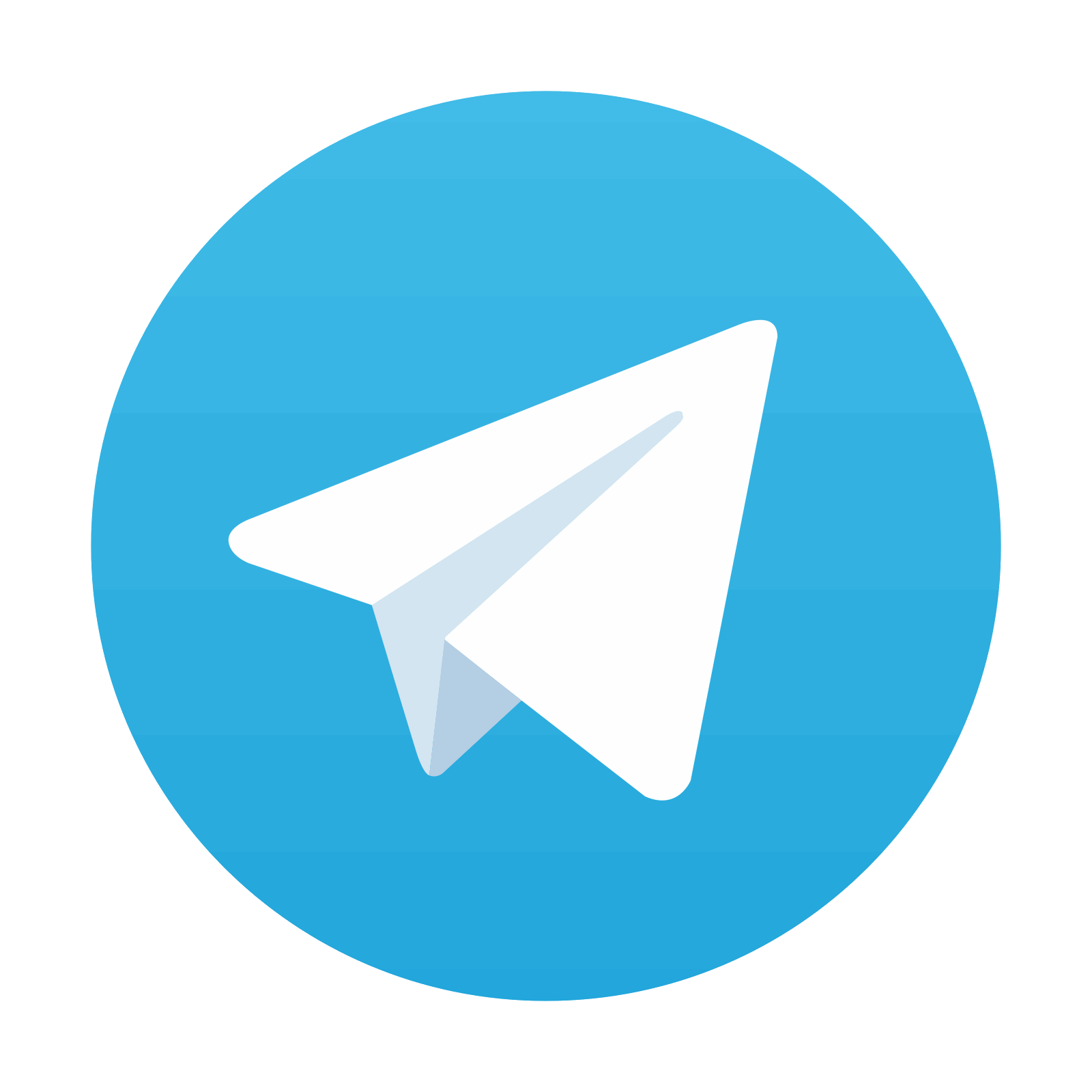
Stay updated, free articles. Join our Telegram channel

Full access? Get Clinical Tree
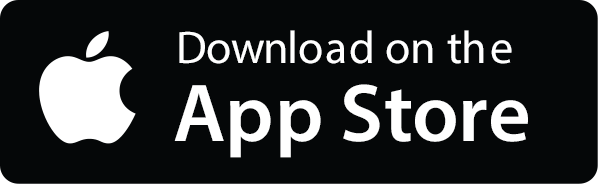
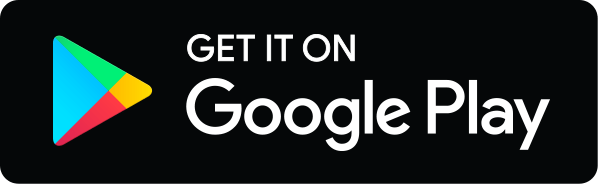