Development of the conceptus from the eight-cell stage to the time of implantation
In the preimplantation phase, new trophoblast develops from the base of the inner cell mass (polar trophoblast). This is the blastocyst stage of development. It is at this morula–blastocyst stage that one of the two X chromosomes of a female embryo becomes deactivated and condensed as a Barr body.
Implantation
Placental attachment to the maternal uterine wall is fundamental to successful human reproduction. Inadequate invasion of the decidua by the trophoblast is associated with various pregnancy complications, while excessive invasion compromises the health of the mother and, therefore, the infant.
The characteristics of trophoblast cells are:
-
paternal X chromosome inactivation
-
unmethylated DNA
-
ability to form multinucleated cells
-
variable expression of major histocompatibility complex (MHC) class 1 antigens; no MHC class 2 antigen expression.
The functions of the trophoblast cells are:
-
attachment of the placenta to the uterine wall
-
transport of nutrients, oxygen and maternal immunoglobulins to the fetus
-
elimination of fetal waste products
-
synthesis and secretion of proteins and hormones
-
barrier between maternal and fetal circulations
-
contact site between maternal immune system and conceptus.
Implantation can be thought of in three phases.
Apposition
Initially at the site of contact between the polar trophoblast and the endometrium, but subsequently more widely, the endometrium increases mitotic activity and undergoes localised changes in stromal cell morphology and intercellular matrix composition. There is also an influx of cells associated with host defence, including natural killer (NK) cells. This process is called decidualisation. The endometrium develops pinopodes, which remove fluid from the uterine cavity (by pinocytosis) and allow closer apposition between the blastocyst and the decidua.
Adhesion
Adhesion is accompanied by destruction of the zona pellucida, allowing contact between maternal and trophoblast cells. Within a few hours of attachment, the surface epithelium adjacent to the trophoblast becomes eroded. This, and the destruction of the zona pellucida, is accomplished by a variety of metalloproteases and by the expression of adhesion molecules on the trophoblast cell membrane that are specific for ligands on the forming decidua.
Penetration
The production of metalloproteases by trophoblast allows the digestion of the extracellular matrix. This facilitates trophoblast invasion into the uterine decidua and makes a variety of lipids, proteins, nucleotides and sugars available as metabolic substrates and fuel. Trophoblast cells can actively transport small molecules across their cell membranes and are able to transport large molecules and cellular fragments by pinocytosis and phagocytosis. This early nutrition is independent of any placental circulation. Trophoblast cells contain microfilaments as part of their internal cytoskeleton. These allow the trophoblast cells to squeeze between endometrial cells and the blastocyst to thereby invade the underlying stroma so deeply that the surface epithelium becomes restored over it (interstitial implantation).
The biochemical signalling involved in these processes is incompletely understood. There may be considerable biochemical redundancy in this control as well, because transgenic mice with genes inactivated for each of the supposed key control cytokines (so‐called ‘knockout mice’) are still able to reproduce successfully. The peptide hormone vascular endothelial growth factor (VEGF) is made in the endometrium with a time course that suggests a key role in the changes in vascularity within the decidua.
Anatomy of the developing placenta
The trophoblast differentiates into two layers: an inner layer of cytotrophoblast, where the cells remain recognisably individual, and an outer syncytiotrophoblast layer, where cellular walls are largely lost (Figure 15.2). Before implantation is complete (11–12 days), lacunae form in the polar syncytiotrophoblast. These trophoblast-lined lacunae will eventually be the intervillous space, allowing circulation of maternal blood in the placental bed. By 13 days after conception, some cells from the trophoblast shell will invade deeply through the decidua and into the myometrium, providing a supportive or attaching function. Also by the end of week 2, primary villous stems are formed. These comprise an outer layer of syncytiotrophoblast cells with a core of cytotrophoblast, which itself has a core of extraembryonic mesoderm (Figure 15.2).
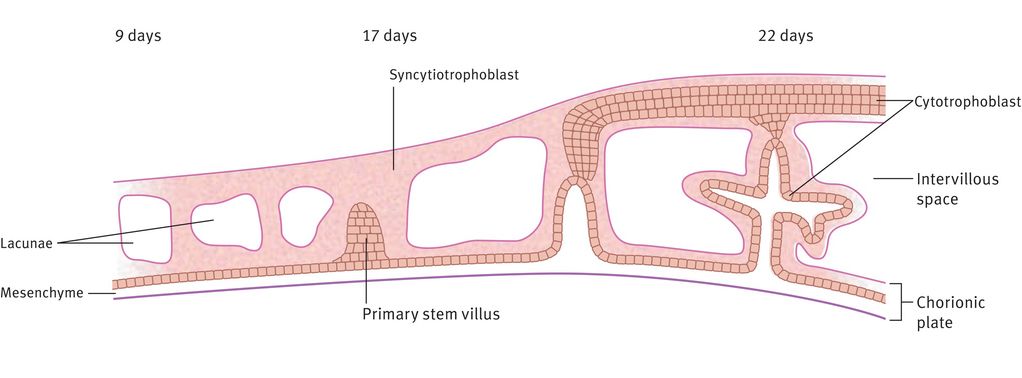
Formation of the intervillous space and primary stem villi
Differentiation of the extraembryonic mesoderm allows vascularisation of the stem villus, which then becomes continuous with the vessels of the body stalk (which will become the umbilical vessels) during week 3 (Figure 15.3). The primary villous stems give rise to syncytiotrophoblast buds, again with a cytotrophoblast and mesodermal core, which become primary villi. Those primary villi adjacent to the uterine cavity regress to form the chorion laeve (nonplacental part of the chorion). The villi on the decidual side of the conceptus (chorion frondosum) undergo further growth and branching as secondary and tertiary villi, filling the intervillous space to form the definitive placenta. It is from the tertiary villi that the true placental villi arise. The elaboration of villi centred on a single primary stem villus is called a placental lobule, of which the placenta has approximately 200. This process is complete by 8–10 weeks of gestation. There is no relation between placental lobules, or groups of lobules, and the cotyledons (or lobes) seen when examining the maternal surface of the term placenta.
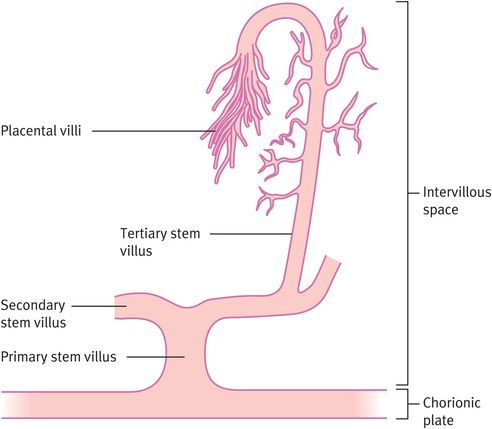
Formation of tertiary stem villi, which provide the architecture of the placenta
By 12 weeks, the placenta has reached its definitive form and subsequently enlarges laterally. The placenta exceeds the weight of the fetus until about 17 weeks and typically weighs about one-sixth of the fetus at term.
The Chorionic Villus
Several different patterns of placental function (Figure 15.4) have developed in different mammalian species and these can be classified on the basis of the cell types intervening between maternal and fetal blood cells. In epitheliochorial placentation, the trophoblast cells attach to the surface epithelium of the uterus (no invasion). Endotheliochorial placentation sees trophoblast invasion of the uterine epithelium but no invasion of maternal blood vessels. Haemochorial placentation shows the greatest invasion, with maternal blood vessels invaded by trophoblast cells, which become bathed in maternal blood. Species with epitheliochorial placentation increase maternal blood flow to the placental bed through decidual angiogenesis (an increased number of similar-sized vessels). This contrasts with human (haemochorial) pregnancy where an increase in blood flow through the placental bed is achieved through trophoblast invasion of the spiral arteries of the decidua and outer myometrium.
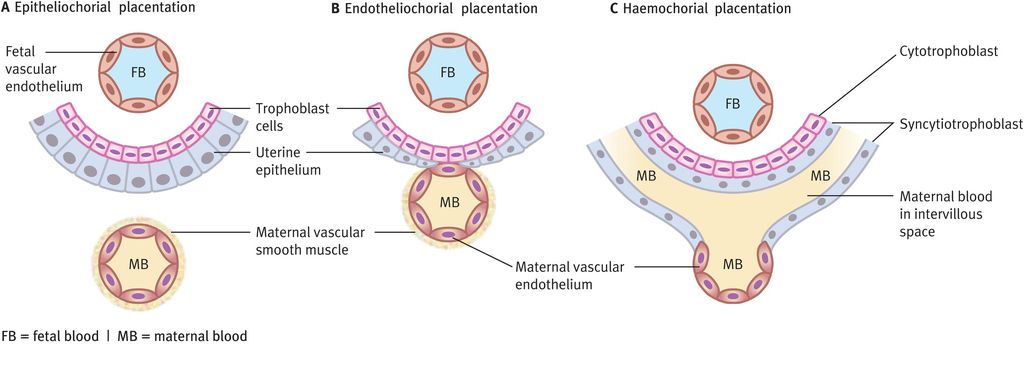
Types of placentation and relationships between fetal trophoblast cells and maternal blood
In the nonpregnant state, the spiral artery is a muscular high-resistance vessel. In pregnancy, trophoblast invasion converts the spiral artery to a wider bore capacitance vessel, allowing blood flow through the uterus to increase 10–15-fold. The mechanism by which trophoblast cells invade decidual spiral arteries and replace the vascular endothelium is only just beginning to be understood. It seems likely that trophoblast cells recognise vascular smooth muscle and endothelial cells through specific adhesion molecule expression. This recognition can induce an intracellular sequence of events that alters trophoblast motility, induces apoptosis in the vascular myocytes and endothelial cells and causes the functional and histological changes in spiral arteries (reduced muscular wall and endothelial lining replaced by trophoblast cells) that are the characteristics of haemochorial placentation.
Two observations about placental architecture in pre-eclampsia have remained constant despite the growing understanding of placental development. Placental villi are less developed in placentas taken from pre-eclamptic pregnancies, with fewer branches and less complex vascular loops than placentas associated with normal pregnancy outcome. The second observation is that change in spiral artery architecture is less evident or patchy when the placental bed is examined histologically at placental bed biopsy (Figure 15.5).
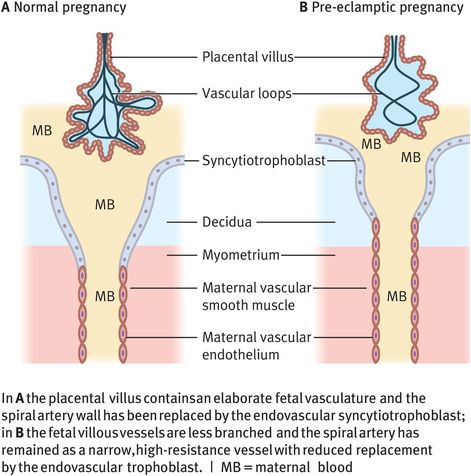
Differences in placental architecture for a normal pregnancy and a pre-eclamptic pregnancy
Immunology of pregnancy tolerance
While there are advantages of close contact between the trophoblast of placental villi and maternal blood (nutrition and waste elimination) as seen in the haemochorial placenta, the disadvantage is that the circulations are no longer separated by an epithelial barrier. Trophoblastic cells in human pregnancy are therefore exposed to potential allogenic immune responses by the mother.
Advances have been made in understanding the immune reactions of pregnancy by focusing on the relationship between trophoblast – the outermost layer of fetal cells – and the cells with which they are in contact. These are the cells of the uterus that undergo highly specialised differentiation during decidualisation. The most obvious features include enlargement of the uterine stromal cells and the presence of a distinct lymphocyte population of uterine NK cells. The presence of NK cells is seen widely in all species with a decidual response to invasive placentation. Similar cells are not seen in other tissues. The roles and characteristics of uterine NK cells are as follows:
-
CD56hiCD16– uterine NK cells represent 70% of leukocytes at the implantation site and increase in number in the late luteal phase of the menstrual cycle and in early pregnancy.
-
Uterine NK cells are similar to a minor (10%) fraction of blood NK cells, but have phenotypic differences, including unusual adhesion molecule expression and poor cytotoxicity.
-
The origin of decidual NK cells, as discrete lineage, or differentiated from circulating NK cells, is unclear.
-
Decidual NK cells produce a range of soluble products including angiogenic cytokines (angiopoietin-2 and vascular endothelial growth factor), immunoregulatory cytokines and chemokines and lytic enzymes.
-
The reduced cytotoxicity of decidual NK cells may relate to a lack of intracellular cytoskeletal and lytic granule organisation.
-
Diagnostic tests on NK cells in peripheral blood of women with reproductive failure give no information about decidual NK cell function.
-
Possible roles for decidual NK cells include maintenance of mucosa and blood vessel stability, modification of spiral arteries in the decidua and regulation of trophoblast invasion of the decidua and its structures.
The relationship between the decidual response in the uterus and invasion has been argued both to permit and to control the degree of invasion. Both roles may occur, allowing the balance of sufficient placental invasion without excess. Uncontrolled trophoblast cell invasion is seen where the decidua is deficient or absent (for example, in tubal ectopic pregnancy or implantation on scar tissue within the uterus). Trophoblast cells implanted at distant ectopic sites (in animal experiments and in in vitro cell culture) show inherent invasive growth. Overinvasion of the fetal–maternal interface compromises the mother’s health, resulting in haemorrhage in the absence of medical intervention.
At the opposite end of the spectrum, the decidua may prevent adequate invasion into the spiral arteries. This shift of fetal–maternal interface in favour of the mother is associated with reduced blood supply to the placental bed and a growth restricted, hypoxic fetus. Pre-eclampsia has a high maternal and fetal mortality and yet has an incidence of 5–10% in first-time mothers. That natural selection has not removed this complication may be a trade in human reproduction: balancing human trophoblasts’ highly invasive tendencies against the consequent risk of maternal haemorrhage, death and subsequent compromise of neonatal survival. Exactly how the degree of trophoblast invasion is controlled is not yet understood, although there is evidence that apoptosis in invasive trophoblast may be a limiting and controlling factor.
Placenta–Uterus Immune Interaction
A mother does not biologically reject her pregnancy but would reject a tissue graft from a genotypically dissimilar donor. The rejection of an allograft (for example, renal or heart transplant) is through powerful antibody and T-cell-mediated responses to allogeneic MHC molecules expressed by the vascularised graft. However, the fetus is not in contact with the mother’s immune system but is shielded by the trophoblast of the placental interface. Trophoblast cells never express MHC class 2 molecules and are thereby unable to present antigen to maternal CD4+ T cells. In humans, the placental trophoblast encounters the maternal immune system in two locations: (i) the villous syncytiotrophoblast is bathed by maternal blood; and (ii) the extravillous cytotrophoblast interacts with uterine tissue.
The syncytiotrophoblast expresses no MHC antigens, which is consistent with the concept that the placenta is immunologically neutral. This theory also fits with the observation that while fetal cells that enter the maternal circulation provoke an immune reaction (for example, rhesus isoimmunisation and haemolytic disease of the newborn), no such systemic T- or B-cell immune response to trophoblast cells has been described.
The second area of contact is between the decidua and the invasive, anchoring, extravillous cytotrophoblast. Unlike the syncytiotrophoblast, the extravillous trophoblast expresses unusual combinations of cell surface human leucocyte antigen (HLA) C, G and E molecules, but does not express polymorphic HLA-A and HLA-B, the molecules that are important in initiating allograft rejection. Polymorphic HLA-C is expressed but does not lead to allograft rejection. There are a number of hypotheses to explain this.1 One difference from allograft rejection is that CD8+ regulatory T cell populations present in the uterus in pregnancy are specific for a carcinoembryonic antigen-like ligand, rather than being MHC restricted. Another mechanism by which the placenta might alter the local immune environment is that trophoblast HLA-G binds with high avidity leukocyte immunoglobulin-like receptors on myelomonocytic cells. Increased expression of these receptors is associated with graft tolerance, possibly through reduced MHC class 2 pathways, and may be important in the decidua where macrophages and dendritic cells expressing MHC class 2 are found.
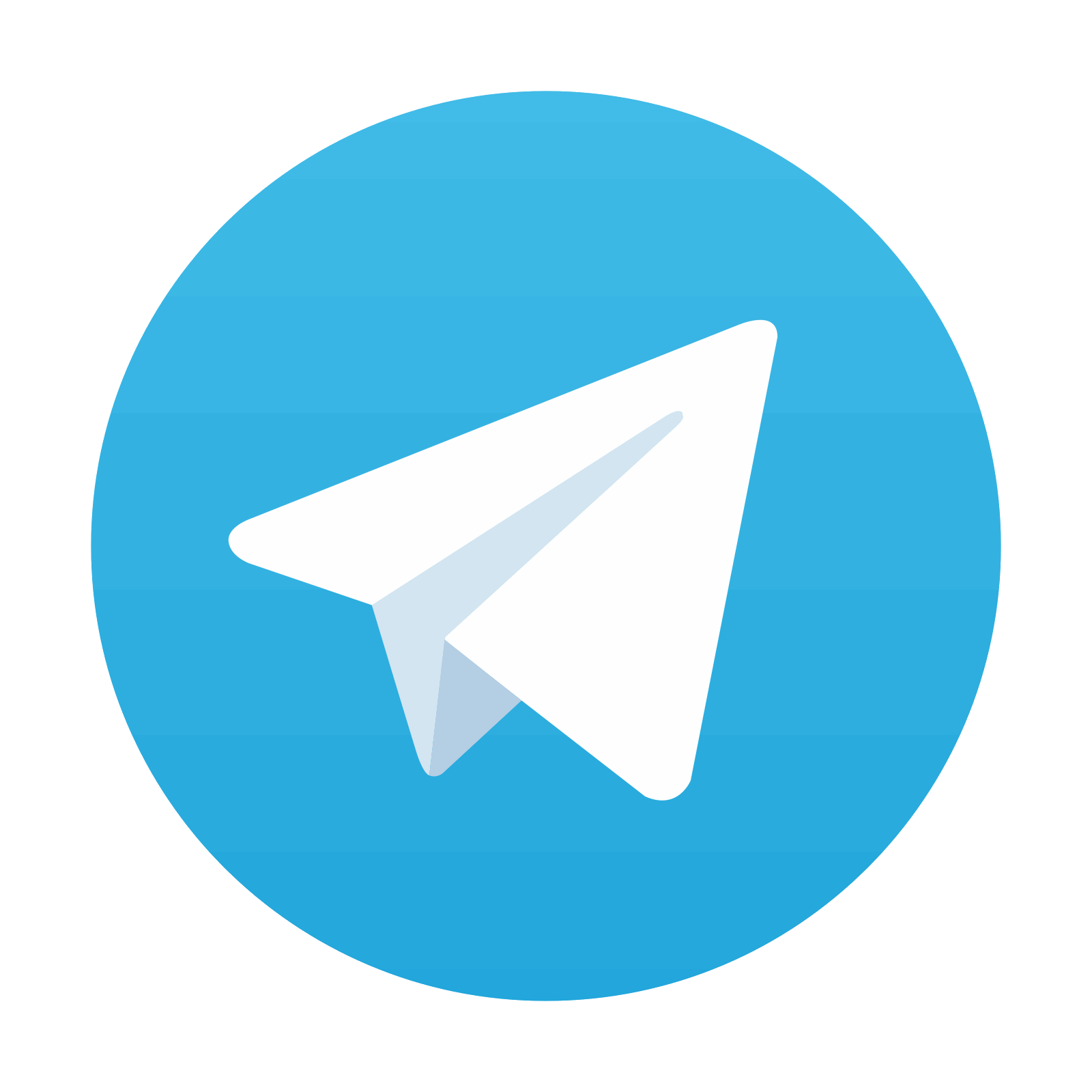
Stay updated, free articles. Join our Telegram channel

Full access? Get Clinical Tree
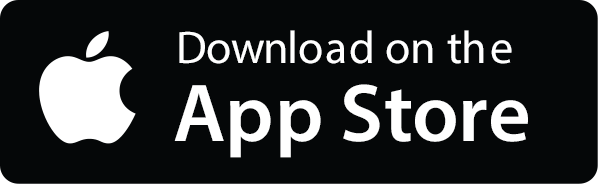
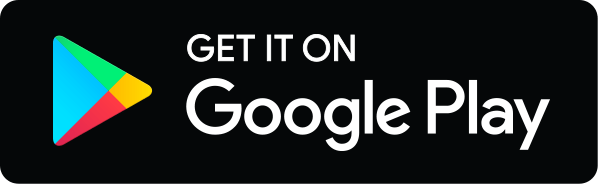