Organ | Function |
---|---|
Kidney | Response to Ca2+, PTH, vitamin D and calcitonin |
Activation of vitamin D | |
Regulation of Ca2+ and PO43− reabsorption and excretion | |
Bone a | Storage (buffer) of Ca2+, PO43− and Mg2+ |
Parathyroid glands | Response to changes in serum Ca2+ (express the CaSR) |
Synthesis and secretion of PTH | |
Gastrointestinal tract | Absorption of Ca2+ and PO43− |
Thyroid glands | Response to changes in serum Ca2+ (express the CaSR) |
Synthesis and secretion of calcitonin |
a Bone remodelling is regulated by PTH, calcitonin and vitamin D | CaSR = Ca2+-sensing receptor | PTH = parathyroid hormone | PO43− = phosphate
Mechanism of Calcium Absorption and Excretion
Ca2+ is absorbed from the intestine via transcellular or paracellular routes (Figure 8.1). Transcellular absorption involves entry of Ca2+ into the cell via the epithelial Ca2+ channel 2 (TRPV6) in the microvilli of the brush border. Bound to Ca2+-binding proteins (calmodulin and calbindin), Ca2+ then moves through the cell to the baso-lateral membrane. Export into the extracellular fluid of the lamina propria occurs by exocytosis or by the PMCA. Paracellular transport also requires the presence of Ca2+-binding proteins.
All of the free (ionised) Ca2+ and the Ca2+ bound to anions is filtered by the glomerulus and most (99%) of the filtered Ca2+ is reabsorbed (Figure 8.2). Ca2+ is reabsorbed by passive paracellular (80%) and active transcellular (20%) processes. Transcellular transport requires the presence of epithelial Ca2+ channel 1 (TRPV5) in the apical membrane.
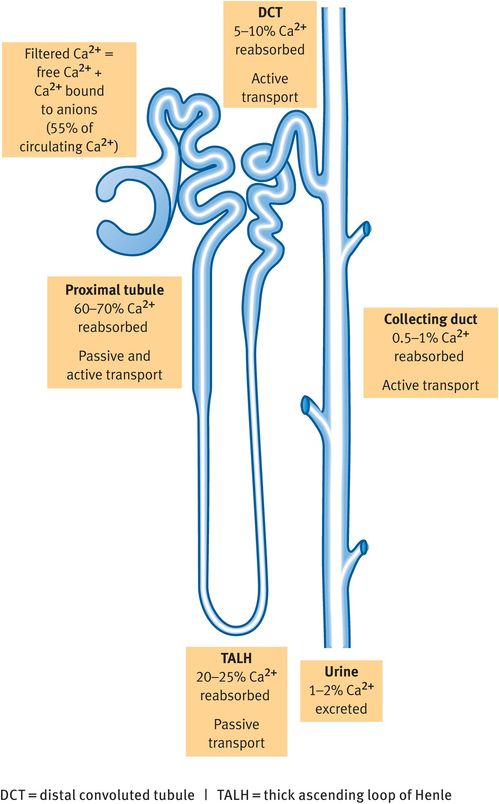
Reabsorption of calcium along the nephron
Calcium Balance
The Ca2+ balance depends on the amount of Ca2+ absorbed minus the amount of Ca2+ excreted, as well as the balance between the amount buffered in the bone and the concentration in the extracellular fluid. Generally, children have a positive Ca2+ balance, in adults the balance should be neutral (input equals output) and postmenopausal women and the elderly usually have a negative Ca2+ balance.
A disruption in Ca2+ homeostasis can result in hypo-calcaemia or hypercalcaemia and it is important to remember that changes in Ca2+ homeostasis will usually alter PO43− status.
Hormones that regulate serum Ca2+ levels
The three main hormones that regulate serum Ca2+ levels are parathyroid hormone (PTH) and vitamin D, which increase serum Ca2+ concentrations, and calcitonin, which reduces serum Ca2+ concentrations. Table 8.4 contains a summary of the characteristics and functions of these hormones.
Hormone | Hormone type | Receptor | Site of synthesis | Signal for synthesis and/or secretion | Physiological actions | Net effect on Ca2+ homeostasis |
---|---|---|---|---|---|---|
PTH | Peptide | G-protein-coupled receptor (PTHR1) | Chief cells in the parathyroid glands | Low Ca2+ | Bone: ↑ bone resorption Kidney: ↑ PO43− excretion Kidney: ↓ Ca2+ excretion Kidney: ↑ vitamin D activation | ↑ Serum Ca2+ ↓ Serum PO43− |
Vitamin D (calcitriol) | Secosteroid | Nuclear receptor (VDR) A member of the steroid/ thyroid receptor superfamily | Hydroxylated (activated) in the proximal convoluted tubules of the kidney Extra-renal activation of vitamin D can also occur | Low Ca2+ Low PO43− PTH | Bone: ↑ bone formation and mineralisation Bone: ↑ bone remodelling GI tract: ↑ Ca2+ absorption Kidney: ↑ Ca2+ and PO43− reabsorption | ↑ Serum Ca2+ ↑ Serum PO43− |
Calcitonin | Peptide | G-protein-coupled receptor | C cells in the parathyroid glands | High Ca2+ | Kidney: ↑ PO43− excretion Kidney: ↓ Ca2+ reabsorption Bone: inhibits osteoclast function | ↓ Serum Ca2+ |
↑ = increase | ↓ = decrease | GI = gastrointestinal | PO43− = phosphate | PTH = parathyroid hormone | PTHR1 = PTH receptor type 1 | VDR = vitamin D receptor
Parathyroid Hormone
PTH is a peptide hormone that is synthesised in the parathyroid glands. These glands (normally four) are located on the posterior of the thyroid gland. They originate from the pharyngeal pouches, are small (3–8 mm long, 2–5 mm wide, 1.5 mm deep with a weight of less than 40 mg each) and are supplied by branches of the inferior thyroid arteries. The parathyroid glands contain two cell types: oxyphil cells, and chief cells, which synthesise and secrete PTH.
Synthesis and release of parathyroid hormone
PTH is synthesised as a 115-amino-acid compound called prepro-PTH. Cleavage reactions produce PTH, an 84-amino-acid molecule that is stored in vesicles until required. The release of PTH is initiated by the Ca2+-sensing receptor (CaSR) in response to hypocalcaemia. PTH secretion occurs within seconds and the parathyroids contain sufficient PTH to maintain secretion for approximately 60 minutes. If prolonged PTH secretion (secretion for hours to days) is required, PTH synthesis is increased.
If the hypocalcaemia is not corrected and PTH secretion needs to be sustained for days or even months, proliferation of the parathyroid gland cells increases and this results in hyperplasia and unregulated synthesis and release of PTH (secondary hyperparathyroidism).
Parathyroid-hormone receptors
PTH binds to the PTH receptor (PTHR), which is a G-protein-coupled receptor. Ligand binding activates cyclic adenosine monophosphate (cAMP) as a second messenger and results in the phosphorylation of intracellular proteins. There are at least two PTHR subtypes, PTHR1 and PTHR2. The first 34 amino acids of PTH bind to PTHR1 and it has classically been assumed that the biological activity of PTH is only attributable to these 34 amino acids. The function of other fragments of the PTH peptide is currently under investigation.
Parathyroid hormone receptors
If the receptor subtype is not specified, reference is probably made to PTHR1.
Physiological actions of parathyroid hormone
PTH acts on the bone and kidney. In bone, it causes bone resorption and, thereby, Ca2+ and PO43− release. PTH binds to PTHRs on the osteoblasts, resulting in osteoblast-mediated activation of osteoclast differentiation. In vitro studies have also shown inhibition of collagen synthesis by PTH-activated osteoblasts.
In the kidney, PTH has three main actions. In the distal convoluted tubule, it increases Ca2+ reabsorption possibly by increasing the transport of Ca2+ that is mediated by TRPV5. In the proximal and distal convoluted tubules, PTH inhibits the reabsorption of PO43− and so increases PO43− excretion. In the proximal tubule, it blocks PO43− resorption by reducing expression of the Na+-dependent inorganic phosphate co-transporter type II (NPT2). PTH also increases the renal activation of vitamin D by increasing expression and activity of the enzyme 25-hydroxyvitamin D3 1α-hydroxylase (CYP27B1; 1α-hydroxylase).
Hyperparathyroidism
Hyperparathyroidism results from excess synthesis and release of PTH and is associated with increased bone resorption. There are two main forms: primary and secondary hyperparathyroidism.
Primary hyperparathyroidism is caused by a defect of the parathyroid glands, normally a benign tumour. Malignant tumours are rare and may be associated with multiple endocrine neoplasia syndrome. Primary hyperparathyroidism is characterised by increased levels of PTH and calcitriol and decreased levels of PO43− and hypercalcaemia. The increase in calcitriol levels and the decrease in PO43− levels indicate that the kidneys are functioning. Individual cells respond normally to feedback signals but the increase in cell numbers means that, on balance, PTH is synthesised and released in excess.
Primary hyperparathyroidism is one of the most frequent causes of hypercalcaemia and is the third most common endocrine disorder after diabetes and hyperthyroidism (prevalence 1 in 500–1000).
Secondary hyperparathyroidism occurs as a result of defective feedback control. Most commonly, the primary defect is in the kidneys and the development of secondary hyperparathyroidism is usually associated with chronic kidney disease.
In renal disease, the kidney is unable to respond normally to PTH, so renal reabsorption of Ca2+ is not stimulated and vitamin D activation is not promoted. As a result, Ca2+ absorption from the intestine cannot be increased on demand. One consequence of this is that the bone is the only place where PTH can act to increase serum Ca2+ levels. The resulting loss of Ca2+ from the bone causes high bone turnover, bone demineralisation (osteomalacia) and chronic kidney disease – mineral and bone disorder. As a consequence of the failure to increase serum Ca2+ levels and the lack of vitamin D signalling, the normal negative feedback on the parathyroid glands is absent and they continue to produce and secrete PTH. This results in hyperplasia and eventually the parathyroid glands lose their sensitivity to Ca2+ and vitamin D.
Ultimately, secondary hyperparathyroidism will progress to tertiary hyperparathyroidism, which is almost exclusively associated with end-stage renal disease and should be treated in the same way as primary hyperparathyroidism.
Calcium-sensing receptor
The CaSR is a G-protein-coupled receptor that is sensitive to serum Ca2+ concentrations in the physiological range. The receptor was originally cloned from bovine parathyroid glands but is known to be expressed in a number of tissues including the kidneys, the intestine, vascular smooth muscle cells and bone. Synthetic allosteric activators of the CaSR (calcimimetics) for the treatment of hyperparathyroidism have been developed. The development of CaSR antagonists (calcilytics) is being explored.
Several disorders related to mutations in the CaSR exist. Familial hypocalciuric hypercalcaemia and neonatal severe hyperparathyroidism are associated with loss-of-function mutations and autosomal dominant hypoparathyroidism is associated with an activating mutation.
Parathyroid-hormone-related peptide
PTH-related peptide (PTHrP, also known as PTH-like peptide) is able to bind to PTHR1 (the first 34 amino acids of PTHrP are similar to those of PTH and the first 13 amino acids of the two peptides are almost identical) and therefore mimics PTH actions. It is produced by many cells including cancer cells and is often responsible for hypercalcaemia associated with malignancy. Its physiological functions are not completely understood. It regulates chondrocyte development and therefore endochondral bone formation, placental Ca2+ transport and fetal development.
Vitamin D
The classification of vitamin D as a vitamin is incorrect because we make most of the vitamin D that we require and vitamin D is a pleiotrophic secosteroid. Dietary intake of vitamin D2 may account for 10–20% of our vitamin D levels but the majority (80–90%) is synthesised in the skin as vitamin D3. The final activation step occurs predominantly in the proximal convoluted tubule of the kidney, although extrarenal synthesis also occurs.
Vitamin D is required for normal bone remodelling and it increases serum levels of Ca2+ and PO43− mainly by increasing their intestinal absorption. A number of different vitamin D metabolites exist. The metabolite that is considered to be the biologically active molecule is 1α,25-dihydroxyvitamin D3 (calcitriol). All vitamin D metabolites are transported in blood bound to vitamin-D-binding protein. The major circulating form of vitamin D is 25-hydroxyvitamin D3 (calcidiol), which is considered to be a good surrogate marker of vitamin D status. It is the metabolite of vitamin D that is usually measured clinically.