Chapter 2
Physiologic Changes of Pregnancy
Robert Gaiser MD
Chapter Outline
Physical Examination and Cardiac Studies
Hemodynamic Changes during Labor and the Puerperium
Metabolism and Respiration during Labor and the Puerperium
Hematology and Coagulation during the Puerperium
Anatomy, Barrier Pressure, and Gastroesophageal Reflux
Gastric Function during Labor and the Puerperium
Neuraxial Analgesia and Anesthesia
Marked anatomic and physiologic changes occur during pregnancy that allow the woman to adapt to the developing fetus and its metabolic demands. The enlarging uterus places mechanical strain on the woman’s body. Greater hormonal production by the ovaries and the placenta further alters maternal physiology. The hallmark of successful anesthetic management of the pregnant woman is recognition of these anatomic and physiologic changes and appropriate adaptation of anesthetic techniques to account for them. The physiologic alterations of normal pregnancy and their anesthetic implications are reviewed in this chapter.
Body Weight and Composition
The mean maternal weight increase during pregnancy is 17% of the prepregnancy weight or approximately 12 kg.1 It results from an increase in the size of the uterus and its contents (uterus, 1 kg; amniotic fluid, 1 kg; fetus and placenta, 4 kg), increases in blood volume and interstitial fluid (approximately 1 kg each), and deposition of new fat and protein (approximately 4 kg). The weight gain during pregnancy recommended by the Institute of Medicine reflects the increased incidence of obesity2 and depends on the prepregnancy body mass index (BMI; Table 2-1). The expected weight increase during the first trimester in a nonobese individual is 1 to 2 kg, and there is a 5- to 6-kg increase in each of the last two trimesters. The recommended gain is less in obese individuals. Obesity is a major problem in the United States and has many implications for obstetric anesthesia (see Chapter 50). Excessive weight gain during pregnancy is a risk factor for a long-term increase in BMI.3
TABLE 2-1
Recommended Weight Gain during Pregnancy
Prepregnancy Body Mass Index (kg/m2) | Total Weight Gain in kg (lb) | Rate of Weight Gain during 2nd and 3rd Trimester in kg/wk (lb/wk) |
< 18.5 | 12.7-18.2 (28-40) | 0.45 (1) |
18.5-24.9 | 11.4-15.9 (25-35) | 0.45 (1) |
25.0-29.9 | 6.8-11.4 (15-25) | 0.27 (0.6) |
≥ 30 | 5.0-9.1 (11-20) | 0.23 (0.5) |
Modified from Institute of Medicine (U.S.) Committee to Reexamine IOM Pregnancy Weight Guidelines, Rasmussen KM, Yaktine AL, editors. Weight Gain During Pregnancy: Reexamining the Guidelines. Washington, DC, National Academies Press, 2009.
Cardiovascular Changes
Physical Examination and Cardiac Studies
Pregnancy causes the heart to increase in size, a result of both greater blood volume and increased stretch and force of contraction.4 These changes, coupled with the elevation of the diaphragm from the expanding uterus, cause several changes in the physical examination and in cardiac studies.
Changes in heart sounds include accentuation of the first heart sound with exaggerated splitting of the mitral and tricuspid components (Box 2-1).5 The second heart sound changes little, although the aortic-pulmonic interval tends to vary less with respiration during the third trimester, a finding without clinical significance. A fourth heart sound may be heard in 16% of pregnant women, although typically it disappears at term. A grade II systolic ejection murmur is commonly heard at the left sternal border6; the murmur is considered a benign flow murmur, attributable to cardiac enlargement from increased intravascular volume, which causes dilation of the tricuspid annulus and regurgitation. Elevation of the diaphragm by the growing uterus shifts the heart anteriorly and to the left. The point of maximal cardiac impulse is displaced cephalad to the fourth intercostal space and also to the left to at least the midclavicular line.
The electrocardiogram typically changes, especially during the third trimester. Heart rate steadily increases during the first and second trimesters, and both the PR interval and the uncorrected QT interval are shortened. This has clinical implications for women with long QT syndrome (see Chapter 42). The QRS axis shifts to the right during the first trimester but may shift to the left during the third trimester.7 Depressed ST segments and isoelectric low-voltage T waves in the left-sided precordial and limb leads are commonly observed.8
Echocardiography demonstrates left ventricular hypertrophy by 12 weeks’ gestation with a 50% increase in mass at term.9 This hypertrophy results from an increase in the size of the preexisting cardiomyocytes rather than an increase in the number of cells. The hypertrophy is eccentric, resembling that occurring from exercise.1 The annular diameters of the mitral, tricuspid, and pulmonic valves increase; 94% of term pregnant women exhibit tricuspid and pulmonic regurgitation, and 27% exhibit mitral regurgitation.10 The aortic annulus is not dilated.
Central Hemodynamics
For accurate determination of central hemodynamic changes during pregnancy, measurements should be made with the patient in a resting position, tilted to the left, to minimize aortic and vena caval compression. Comparisons must be made with an appropriate control, such as prepregnancy values or a matched group of nonpregnant women. If control measurements are made during the postpartum period, a sufficient interval must have elapsed for hemodynamic parameters to have returned to prepregnancy values; this may take 24 weeks or more.11
Cardiac output begins to increase by 5 weeks’ gestation and is 35% to 40% above baseline by the end of the first trimester.9,12 It continues to increase throughout the second trimester until it is approximately 50% greater than nonpregnant values (Figures 2-1 and 2-2).9,11,13–15 Cardiac output does not change from this level during the third trimester. Some studies have reported a decrease in cardiac output during the third trimester; typically this is when measurements are made in the supine position and thus reflects aortocaval compression rather than a true gestational decline.
FIGURE 2-1 Central hemodynamic changes at term gestation. Changes are relative to the nonpregnant state. CO, cardiac output; SV, stroke volume; HR, heart rate; LVEDV, left ventricular end-diastolic volume; LVESV, left ventricular end-systolic volume; EF, ejection fraction; LVSWI, left ventricular stroke work index; PCWP, pulmonary capillary wedge pressure; PADP, pulmonary artery diastolic pressure; CVP, central venous pressure; SVR, systemic vascular resistance; NC, no change. (Data from Conklin KA. Maternal physiological adaptations during gestation, labor, and puerperium. Semin Anesth 1991; 10:221-34.)
FIGURE 2-2 Cardiac output during pregnancy, labor, and the puerperium. Values during pregnancy are measured at the end of the first, second, and third trimesters. Values during labor are measured between contractions. For each measurement, the relative contributions of heart rate (HR) and stroke volume (SV) to the change in cardiac output are illustrated.
The initial increase in cardiac output results from an increase in heart rate, which occurs by the fourth to fifth week of pregnancy.9 The heart rate increases 15% to 25% above baseline by the end of the first trimester and remains relatively unchanged from that level for the remainder of the pregnancy.9,11–16 Cardiac output continues to increase during the second trimester because of an increase in stroke volume. Stroke volume increases by approximately 20% during the first trimester and by 25% to 30% above baseline during the second trimester.9,11,12,16 The increase in stroke volume correlates with increasing estrogen levels.1 Left ventricular mass increases by 23% from the first to the third trimester.17 Cardiac output increases to meet the demands of the developing fetus, and the distribution of cardiac output to the uterine circulation increases from 5% to 12% during the second half of pregnancy.18
Left ventricular end-diastolic volume increases during pregnancy, whereas end-systolic volume remains unchanged, resulting in a larger ejection fraction.9,11–14,16 Central venous, pulmonary artery diastolic, and pulmonary capillary wedge pressures are within the normal nonpregnant range.15 The apparent discrepancy between left ventricular filling pressure and end-diastolic volume is explained by hypertrophy and dilation, with the dilated ventricle accommodating a greater volume without an increase in pressure.
Myocardial contractility increases, as demonstrated by higher velocity of left ventricular circumferential fiber shortening (Figure 2-3).9,13,16 Tissue Doppler imaging, which is relatively independent of preload, has been used to assess diastolic function.19 Left ventricular diastolic function is not impaired during pregnancy, whereas systolic function is increased during the second trimester.
FIGURE 2-3 Left ventricular function in late phase of third-trimester normotensive pregnant patients. LVSWI, left ventricular stroke work index; PCWP, pulmonary capillary wedge pressure. (Modified from Clark SL, Cotton DB, Lee W, et al. Central hemodynamic assessment of cardiac function. Am J Obstet Gynecol 1989; 161:439-42.)
The increase in cardiac output during pregnancy results in increased perfusion to the uterus, kidneys, and extremities. Uterine blood flow increases from a baseline value of approximately 50 mL/min to a level at term of 700 to 900 mL/min.20–24 Approximately 90% of this flow perfuses the intervillous space, with the balance perfusing the myometrium.22 At term, skin blood flow is approximately three to four times the nonpregnant level, resulting in higher skin temperature.25 Renal plasma flow is increased by 80% at 16 to 26 weeks’ gestation but declines to 50% above the nonpregnant baseline at term.26
The U.S. Department of Health and Human Services recommends that pregnant women have at least 150 minutes of moderate-intensity aerobic activity every week27; however, most women do not achieve this goal. Pregnant women are less active, with only half as many meeting guidelines for vigorous activity compared with nonpregnant women.28 For every two women who exercise before pregnancy, one will not do so during pregnancy. Failure to exercise results in greater gestational weight gain.29 Exercise is safe for the fetus29,30; in a study of 45 women, exercise on a treadmill of moderate intensity (40% to 59% of heart rate reserve) did not affect fetal heart or umbilical artery Doppler indices.30
During exercise, maximal oxygen consumption is greater in pregnancy,31 especially during cardiovascular exercise. The rate of increase in minute ventilation is greater with exercise during pregnancy.32 Cardiac output is also greater, primarily from increased stroke volume33 and increased oxygen delivery to the fetus.
Blood Pressure
Positioning, gestational age, and parity affect blood pressure measurements. Brachial sphygmomanometry yields the highest measurements in the supine position and the lowest measurements in the lateral position.14,34 Blood pressure increases with maternal age, and for a given age, nulliparous women have a higher mean pressure than parous women.35 Systolic, diastolic, and mean blood pressure decrease during midpregnancy and return toward baseline as the pregnancy approaches term.36 Diastolic blood pressure decreases more than systolic blood pressure, with early to mid-gestational decreases of approximately 20%.37 The changes in blood pressure are consistent with changes in systemic vascular resistance, which decreases during early gestation, reaches its nadir (35% decline) at 20 weeks’ gestation, and increases during late gestation. Unlike blood pressure, systemic vascular resistance remains approximately 20% below the nonpregnant level at term.11,15 A postulated explanation for the decreased systemic vascular resistance is the development of a low-resistance vascular bed (the intervillous space) as well as vasodilation caused by prostacyclin, estrogen, and progesterone. The lower blood pressure persists beyond the pregnancy. A longitudinal study of 2304 initially normotensive women over 20 years showed that nulliparous women at baseline who subsequently delivered one or more infants had a blood pressure that was 1 to 2 mm Hg lower than corresponding women who did not have children. This finding demonstrates that pregnancy may create long-lasting vascular changes.37
Aortocaval Compression
The extent of compression of the aorta and inferior vena cava by the gravid uterus depends on positioning and gestational age. At term, partial vena caval compression occurs when the woman is in the lateral position, as documented by angiography.38 This finding is consistent with the 75% elevation above baseline of femoral venous and lower inferior vena cava pressures.39 Despite caval compression, collateral circulation maintains venous return, as reflected by the right ventricular filling pressure, which is unaltered in the lateral position.15
In the supine position, nearly complete obstruction of the inferior vena cava is evident at term.40 Blood returns from the lower extremities through the intraosseous, vertebral, paravertebral, and epidural veins.41 However, this collateral venous return is less than would occur through the inferior vena cava, resulting in a decrease in right atrial pressure.42 Compression of the inferior vena cava occurs as early as 13 to 16 weeks’ gestation and is evident from the 50% increase in femoral venous pressure observed when these women assume the supine position (Figure 2-4).43 By term, femoral venous and lower inferior vena caval pressures are approximately 2.5 times the nonpregnant measurements in the supine position.39,43
FIGURE 2-4 Femoral and antecubital venous pressures in the supine position throughout normal pregnancy and the puerperium. (Modified from McLennan CE. Antecubital and femoral venous pressure in normal and toxemic pregnancy. Am J Obstet Gynecol 1943; 45:568-91.)
In the supine position, the aorta may be compressed by the term gravid uterus. This compression accounts for lower pressure in the femoral versus the brachial artery in the supine position.44,45 These findings are consistent with angiographic studies in supine pregnant women, which show partial obstruction of the aorta at the level of the lumbar lordosis and enhanced compression during periods of maternal hypotension.46
At term, the left lateral decubitus position results in less enhancement of cardiac sympathetic nervous system activity and less suppression of cardiac vagal activity than the supine or right lateral decubitus position.47 Women who assume the supine position at term gestation experience a 10% to 20% decline in stroke volume and cardiac output,48,49 consistent with the fall in right atrial filling pressure. Blood flow in the upper extremities is normal, whereas uterine blood flow decreases by 20% and lower extremity blood flow decreases by 50%.50 Perfusion of the uterus is less affected than that of the lower extremities because compression of the vena cava does not obstruct venous outflow via the ovarian veins.51 The adverse hemodynamic effects of aortocaval compression are reduced once the fetal head is engaged.44,45 The sitting position has also been shown to result in aortocaval compression, with a decrease in cardiac output of 10%.52 Flexing the legs rotates the uterus to compress against the vena cava. Short intervals in the sitting position, such as occurs during epidural catheter placement, have no impact on uteroplacental blood flow.
Some term pregnant women exhibit an increase in brachial artery blood pressure when they assume the supine position, which is caused by higher systemic vascular resistance from compression of the aorta. Up to 15% of women at term experience bradycardia and a substantial drop in blood pressure when supine, the so-called supine hypotension syndrome.53 It may take several minutes for the bradycardia and hypotension to develop, and the bradycardia is usually preceded by a period of tachycardia. The syndrome results from a profound drop in venous return for which the cardiovascular system is not able to compensate.
Hemodynamic Changes during Labor and the Puerperium
Cardiac output during labor (but between uterine contractions) increases from pre-labor values by approximately 10% in the early first stage, by 25% in the late first stage, and by 40% in the second stage of labor.54–56 In the immediate postpartum period, cardiac output may be as much as 75% above pre-delivery measurements.55 These changes result from an increase in stroke volume due to greater venous return and to alterations in sympathetic nervous system activity. During uterine contractions, 300 to 500 mL of blood is displaced from the intervillous space into the central circulation (“autotransfusion”).57–59 Increased intrauterine pressure forces blood from the intervillous space through the relatively unimpeded ovarian venous outflow system. The postpartum increase in cardiac output results from relief of vena caval compression, diminished lower extremity venous pressure, and a reduction of maternal vascular capacitance.56 Cardiac output decreases to just below pre-labor values at 24 hours postpartum57 and returns to prepregnancy levels between 12 and 24 weeks postpartum.11 Heart rate decreases rapidly after delivery, reaches prepregnancy levels by 2 weeks postpartum, and is slightly below the prepregnancy rate for the next several months.11,60 Other anatomic and functional changes of the heart are also fully reversible.18,61
The Respiratory System
Despite the multiple anatomic and physiologic changes that occur during pregnancy, it is remarkable that pregnancy has a relatively minor impact on lung function.
Anatomy
The thorax undergoes both mechanical and hormonal changes during pregnancy.62,63 Relaxin (the hormone responsible for relaxation of the pelvic ligaments) causes relaxation of the ligamentous attachments to the lower ribs.62,63 The subcostal angle progressively widens from 68.5 to 103.5 degrees. The anteroposterior and transverse diameters of the chest wall each increase by 2 cm, resulting in an increase of 5 to 7 cm in the circumference of the lower rib cage. These changes peak at 37 weeks’ gestation. The subcostal angle remains about 20% wider than the baseline value after delivery.64 The vertical measurement of the chest cavity decreases by as much as 4 cm as a result of the elevated position of the diaphragm.
Capillary engorgement of the larynx and the nasal and oropharyngeal mucosa begins early in the first trimester and increases progressively throughout pregnancy.65 The effect of estrogen on the nasal mucosa leads to symptoms of rhinitis and nosebleeds. Nasal breathing commonly becomes difficult, and epistaxis may occur. Nasal congestion may contribute to the perceived shortness of breath of pregnancy.66 Throughout the first and second trimesters, the voice has been described as rounded and well carried with good vibration. During the third trimester, vocal cord fatigue is more prevalent with a decrease in the maximum time of phonation. Both of these changes resolve in the immediate postpartum period.67
Airflow Mechanics
Inspiration in the term pregnant woman is almost totally attributable to diaphragmatic excursion68 because of greater descent of the diaphragm from its elevated resting position and limitation of thoracic cage expansion because of its expanded resting position (Table 2-2). Both large- and small-airway function are minimally altered during pregnancy. The shape of flow-volume loops, the absolute flow rates at normal lung volumes,69 forced expiratory volume in one second (FEV1), the ratio of FEV1 to forced vital capacity (FVC), and closing capacity are unchanged during pregnancy.70 There is no significant change in respiratory muscle strength during pregnancy despite the cephalad displacement of the diaphragm. Furthermore, despite the upward displacement of the diaphragm by the gravid uterus, diaphragm excursion actually increases by 2 cm.71
TABLE 2-2
Effects of Pregnancy on Respiratory Mechanics
Parameter | Change* |
Diaphragm excursion | Increased |
Chest wall excursion | Decreased |
Pulmonary resistance | Decreased 50% |
FEV1 | No change |
FEV1/FVC | No change |
Flow-volume loop | No change |
Closing capacity | No change |
* Relative to nonpregnant state.
FEV1, Forced expiratory volume in 1 second; FVC, forced vital capacity.
Adapted from Conklin KA. Maternal physiological adaptations during gestation, labor, and the puerperium. Semin Anesth 1991; 10:221-34.
The peak expiratory flow rate achieved with a maximal effort after a maximal inspiration is often considered a surrogate for the FEV1 and is often used to monitor asthma therapy. Studies of changes in peak expiratory flow rate during pregnancy have had conflicting results, most likely reflecting differences in measurement devices and patient position during measurements. Nonetheless, Harirah et al.72 found that peak expiratory flow rate declined throughout gestation in all positions and that flow rates in the supine position were lower than those during standing and sitting. The mean rate of decline was 0.65 L/min per week, and peak expiratory flow rate remained below normal at 6 weeks postpartum. By contrast, Grindheim et al.73 reported that peak expiratory flow rate increased in 100 pregnant women followed longitudinally, starting at an average of 6.7 L/s in the early second trimester and peaking at 7.2 L/s at term (Figure 2-5). These authors also reported that the FVC increased by 100 mL after 14 to 16 weeks’ gestation, with the change being greater in parous than in primigravid women.73 The changes in functional residual capacity (FRC) that occur during pregnancy may persist postpartum.
FIGURE 2-5 Changes in airflow mechanics during pregnancy. The magnitude of the increase in flow rates is small. The forced expiratory volume in one second (FEV1) is within the normal range of predictive values for nonpregnant individuals. FVC, forced vital capacity; PEF, peak expiratory flow. (Based on data from Grindheim G, Toska K, Estensen ME, Rosseland LA. Changes in pulmonary function during pregnancy: a longitudinal study. BJOG 2012; 119:94-101.)
Lung Volumes and Capacities
Lung volumes can be measured using body plethysmography or by inert gas techniques with slightly differing results.74 During pregnancy, total lung capacity is slightly reduced,75 whereas tidal volume increases by 45%, with approximately half the change occurring during the first trimester (Table 2-3 and Figure 2-6). The early change in tidal volume is associated with a reduction in inspiratory reserve volume. Residual volume tends to drop slightly, a change that maintains vital capacity. Inspiratory capacity increases by 15% during the third trimester because of increases in tidal volume and inspiratory reserve volume.76,77 There is a corresponding decrease in expiratory reserve volume.76,77 The FRC begins to decrease by the fifth month of pregnancy and decreases by 400 to 700 mL to 80% of the prepregnancy value at term.76,77 This change is caused by elevation of the diaphragm as the enlarging uterus enters the abdominal cavity and is accounted for by a 25% reduction in expiratory reserve volume (200 to 300 mL) and a 15% reduction in residual volume (200 to 400 mL). Assumption of the supine position causes the FRC to decrease further to 70% of the prepregnancy value. The supine FRC can be increased by 10% (approximately 188 mL) by placing the patient in a 30-degree head-up position.78
TABLE 2-3
Changes in Respiratory Physiology at Term Gestation
Parameter | Change* |
Lung Volumes | |
Inspiratory reserve volume | +5% |
Tidal volume | +45% |
Expiratory reserve volume | −25% |
Residual volume | −15% |
Lung Capacities | |
Inspiratory capacity | +15% |
Functional residual capacity | −20% |
Vital capacity | No change |
Total lung capacity | −5% |
Ventilation | |
Minute ventilation | +45% |
Alveolar ventilation | +45% |
* Relative to nonpregnant state.
From Conklin KA. Maternal physiological adaptations during gestation, labor and the puerperium. Semin Anesth 1991; 10:221-34.
Ventilation and Blood Gases
During pregnancy, respiratory rate and pattern remain relatively unchanged. Minute ventilation increases via an increase in tidal volume from 450 to 600 mL and a small increase in respiratory rate of 1 to 2 breaths/min.79 This occurs primarily during the first 12 weeks of gestation with a minimal increase thereafter. The ratio of total dead space to tidal volume remains constant during pregnancy, resulting in an increase in alveolar ventilation of 30% to 50% above baseline. The increase in minute ventilation results from hormonal changes and from an increase in CO2 production at rest by approximately 30% to 300 mL/min. The latter is closely related to the blood level of progesterone,80 which acts as a direct respiratory stimulant. The progesterone-induced increase in chemosensitivity results in a steeper slope and a leftward shift of the CO2-ventilatory response curve. This change occurs early in pregnancy and remains constant until delivery.69
Dyspnea is a common complaint during pregnancy, affecting up to 75% of women.81 Contributing factors include increased respiratory drive, decreased PaCO2, the enlarging uterus, larger pulmonary blood volume, anemia, and nasal congestion. Dyspnea typically begins in the first or second trimester but improves as the pregnancy progresses. In a study in which 35 women were observed closely during pregnancy and postpartum, dyspnea was not due to alterations in central ventilatory control or respiratory mechanical factors but rather to the awareness of the increased ventilation.82 Exercise has no effect on pregnancy-induced changes in ventilation or alveolar gas exchange.83 The hypoxic ventilatory response is increased during pregnancy to twice the normal level, secondary to elevations in estrogen and progesterone levels.84 This increase occurs despite blood and cerebrospinal fluid (CSF) alkalosis.
During pregnancy, PaO2 increases to 100 to 105 mm Hg (13.3 to 14.0 kPa) as a result of greater alveolar ventilation (Table 2-4).85 The higher PaO2 results from the decline in PaCO2 and a lower arteriovenous oxygen difference, which reduces the impact of venous admixture on PaO2.86,87 As pregnancy progresses, oxygen consumption continues to increase, and cardiac output increases to a lesser extent, resulting in a reduced mixed venous oxygen content and increased arteriovenous oxygen difference. After mid gestation, pregnant women in the supine position frequently have a PaO2 less than 100 mm Hg (13.3 kPa). This occurs because the FRC may be less than closing capacity, resulting in closure of small airways during normal tidal volume ventilation.85 Moving a pregnant woman from the supine to the erect or lateral decubitus position improves arterial oxygenation and reduces the alveolar-to-arterial oxygen gradient. The increased oxygen tension facilitates the transfer of oxygen across the placenta to the fetus.
TABLE 2-4
Blood Gas Measurements during Pregnancy
PaCO2 declines to approximately 30 mm Hg (4.0 kPa) by 12 weeks’ gestation but does not change further during the remainder of pregnancy. Although a gradient exists between the end-tidal CO2 tension and PaCO2 in nonpregnant women, the two measurements are equivalent during early pregnancy,88 at term gestation,89 and in the postpartum period.90 This is attributable to a reduction in alveolar dead space, which results from an increase in cardiac output during pregnancy. The mixed venous PCO2 is 6 to 8 mm Hg (0.8 to 1.1 kPa) below the nonpregnant level from the later first trimester until term.1
Metabolic compensation for the respiratory alkalosis of pregnancy reduces serum bicarbonate concentration to approximately 20 mEq/L, the base excess by 2 to 3 mEq/L, and the total buffer base by approximately 5 mEq/L.91 This compensation is incomplete, as demonstrated by the elevation of venous,92 capillary,93 and arterial85 blood pH by 0.02 to 0.06 units.
Metabolism and Respiration during Labor and the Puerperium
Minute ventilation in the unmedicated parturient increases by 70% to 140% in the first stage of labor and by 120% to 200% in the second stage of labor compared with prepregnancy values.94 Pain, anxiety, and coached breathing techniques increase minute ventilation. PaCO2 may decrease to as low as 10 to 15 mm Hg (1.3 to 2.0 kPa). Oxygen consumption increases above the pre-labor value by 40% in the first stage and by 75% in the second stage, secondary to the increased metabolic demands of hyperventilation, uterine activity, and maternal expulsive efforts.94,95 The maternal aerobic requirement for oxygen exceeds oxygen consumption during labor, as is evident from the progressive elevation of blood lactate concentration, an index of anaerobic metabolism.95–98 Provision of effective neuraxial analgesia prevents these changes during the first stage of labor and mitigates the changes during the second stage of labor.95,98
FRC increases after delivery but remains below the prepregnancy volume for 1 to 2 weeks. Although minute ventilation decreases halfway toward nonpregnant values by 72 hours, oxygen consumption, tidal volume, and minute ventilation remain elevated until at least 6 to 8 weeks after delivery. The alveolar and mixed venous PCO2 values increase slowly after delivery and are still slightly below prepregnancy levels at 6 to 8 weeks postpartum.1
Hematology
Blood Volume
Maternal plasma volume expansion begins as early as 6 weeks’ gestation and continues until it reaches a net increase of approximately 50% by 34 weeks’ gestation (Table 2-5, Figure 2-7).99–102 After 34 weeks’ gestation, the plasma volume stabilizes or decreases slightly. Red blood cell volume decreases during the first 8 weeks of pregnancy, increases to the prepregnancy level by 16 weeks, and undergoes a further rise to 30% above the prepregnancy level at term.100,102,103 The increase in plasma volume exceeds the increase in red blood cell volume, resulting in the physiologic anemia of pregnancy. Hemoglobin concentration, which typically ranges from 12 to 15.8 g/dL in the nonpregnant woman, decreases to 11.6 to 13.9 g/dL in the first trimester, 9.7 to 14.8 g/dL in the second trimester, and 9.5 to 15.0 g/dL in the third trimester.104 Hematocrit, which ranges from 35.4% to 44.4% in the nonpregnant woman, decreases to 31% to 41% in the first trimester, 30% to 39% in the second trimester, and 28% to 40% in the third trimester.100 There is an increase in plasma volume from 49 to 67 mL/kg, an increase in total blood volume from 76 to 94 mL/kg, and little change in red cell volume (27 mL/kg) (Figure 2-8).100 Blood volume is positively correlated with the size of the fetus in singleton pregnancies and is greater in multiple gestations.101 The physiologic hypervolemia facilitates delivery of nutrients to the fetus, protects the mother from hypotension, and reduces the risks associated with hemorrhage at delivery. The decrease in blood viscosity from the lower hematocrit creates lower resistance to blood flow, which may be an essential component of maintaining the patency of the uteroplacental vascular bed.
TABLE 2-5
Hematologic Parameters at Term Gestation
Parameter | Change* or Actual Measurement |
Blood volume | +45%* |
Plasma volume | +55%* |
Red blood cell volume | +30%* |
Hemoglobin concentration (g/dL) | 11.6 |
Hematocrit | 35.5% |
* Relative to nonpregnant state.
Adapted from Conklin KA. Maternal physiological adaptations during gestation, labor, and puerperium. Semin Anesth 1991; 10:221-34.
FIGURE 2-7 Blood volume during pregnancy and the puerperium. Values during pregnancy measured at the end of the first, second, and third trimesters. Postpartum values measured after a vaginal delivery. The values for red blood cell volume (RBC) and plasma volume (Plasma) do not represent the actual percentage of change in these parameters but rather reflect the relative contribution of each to the change in blood volume. The asterisk indicates that RBC volume is below the prepregnancy volume at the end of the first trimester.
FIGURE 2-8 The decrease in both hemoglobin concentration and hematocrit during pregnancy underlies the physiologic anemia of pregnancy. The decrease is greater for hematocrit and the greatest decreases occur during the third trimester. (Based on data from Abbassi-Ghanavati M, Greer LG, Cunningham FG. Pregnancy and laboratory studies: a reference table for clinicians. Obstet Gynecol 2009; 114:1326-31.)
The increase in plasma volume results from fetal and maternal hormone production, and several systems may play a role. Additionally, the expansion of plasma volume may help to maintain blood pressure in the presence of decreased vascular tone.103,105 The maternal concentrations of estrogen and progesterone increase nearly 100-fold during pregnancy. Estrogens increase plasma renin activity, enhancing renal sodium absorption and water retention via the renin-angiotensin-aldosterone system. Fetal adrenal production of the estrogen precursor dehydroepiandrosterone may be the underlying control mechanism. Progesterone also enhances aldosterone production. These changes result in marked increases in plasma renin activity and aldosterone level as well as in retention of approximately 900 mEq of sodium and 7000 mL of total body water. The concentration of plasma adrenomedullin, a potent vasodilating peptide, increases during pregnancy and correlates significantly with blood volume.106
Red blood cell volume increases in response to elevated erythropoietin concentration107 and the erythropoietic effects of progesterone, prolactin, and placental lactogen. Both hemoglobin concentration and hematocrit decrease after conception to approximately 11.2 g/dL and 34%, respectively, by mid gestation,102,103 which is a 15% decrease from prepregnancy levels. During the late third trimester, the hemoglobin concentration and hematocrit increase to approximately 11.6 g/dL and 35.5%, respectively, because red blood cell volume increases more than plasma volume. Women who do not receive iron supplements during pregnancy have greater decreases in hemoglobin concentration and hematocrit.102
Plasma Proteins
Plasma albumin concentration decreases from a nonpregnant range of 4.1-5.3 g/dL to 3.1-5.1 g/dL in the first trimester, 2.6-4.5 g/dL in the second trimester, and 2.3-4.2 g/dL in the third trimester (Table 2-6).104,108,109 The globulin level decreases by 10% in the first trimester and then increases throughout the remainder of pregnancy to 10% above the prepregnancy value at term.108 The albumin-globulin ratio decreases during pregnancy from 1.4 to 0.9, and the total plasma protein concentration decreases from 7.8 to 7.0 g/dL.109 Maternal colloid osmotic pressure decreases by approximately 5 mm Hg during pregnancy.15,110,111 The plasma cholinesterase concentration falls by approximately 25% during the first trimester and remains at that level until the end of pregnancy.112,113
TABLE 2-6
Plasma Protein Values during Pregnancy
Coagulation
Pregnancy is associated with enhanced platelet turnover, clotting, and fibrinolysis (Box 2-2). Thus, pregnancy represents a state of accelerated but compensated intravascular coagulation.
Increases in platelet factor 4 and beta-thromboglobulin signal elevated platelet activation, and the progressive increase in platelet distribution width and platelet volume are consistent with greater platelet consumption during pregnancy.114–116 Platelet aggregation in response to collagen, epinephrine, adenosine diphosphate, and arachidonic acid is increased.117
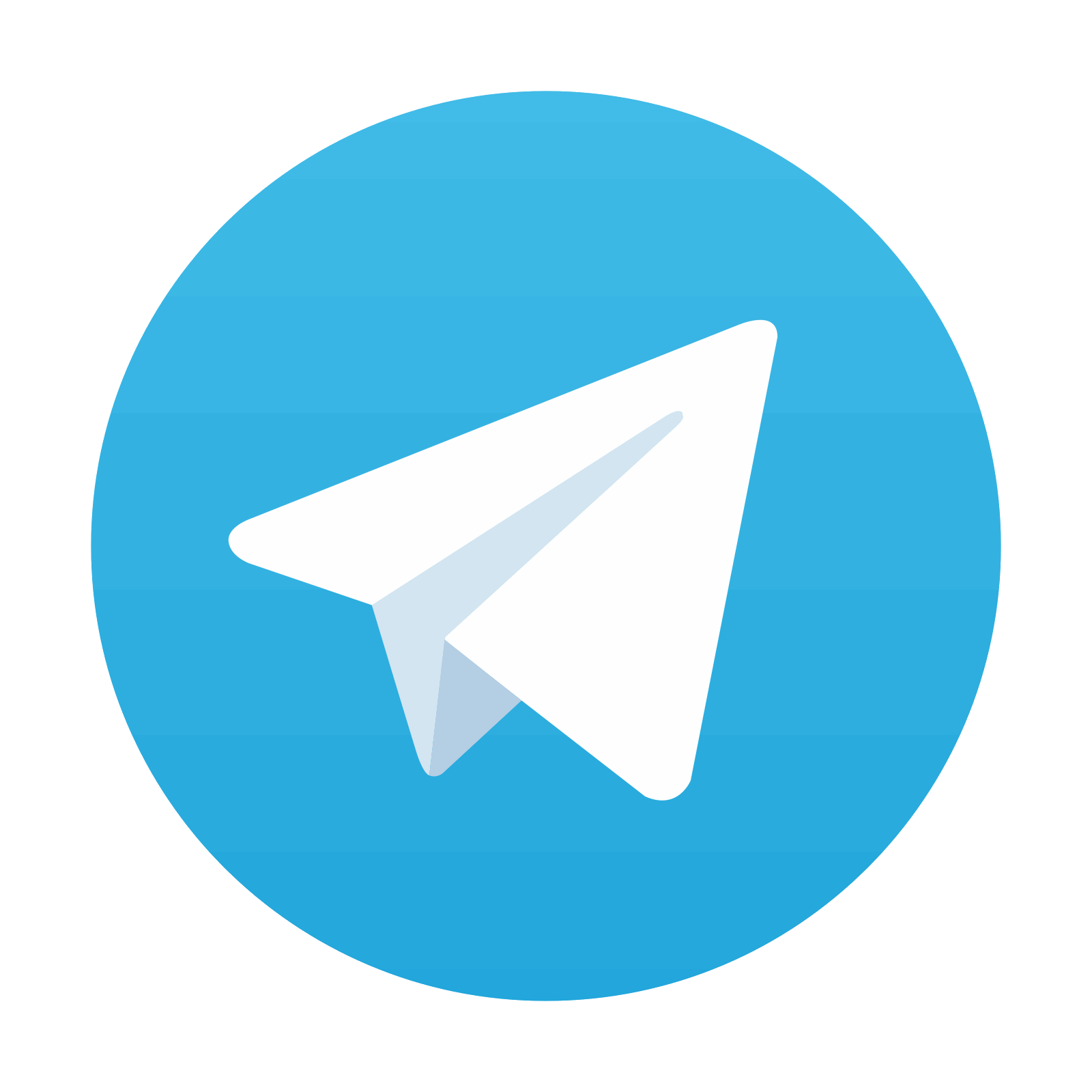
Stay updated, free articles. Join our Telegram channel

Full access? Get Clinical Tree
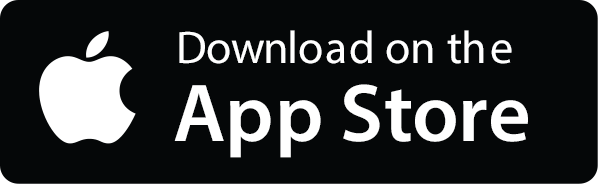
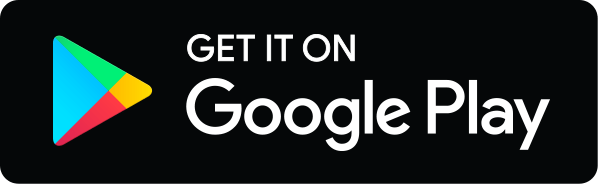