Perinatal Stroke
INTRODUCTION
Neonatal stroke is estimated to occur in 1 per 2300 to 5000 live births.1 This estimate may be an underrepresentation of the true incidence of neonatal stroke because of the inherent challenges in diagnosing this condition. In the adult, stroke is defined as “rapidly developed signs of focal (or global) disturbance of cerebral function lasting greater than 24 hours (unless interrupted by surgery or death), with no apparent nonvascular cause.”2 In this definition, there is heavy reliance on the clinical signs of neurological dysfunction. These signs are not usually apparent in the neonate because motor dysfunction is not easily appreciated, and sophisticated repertoires of behaviors such as language or the ability to follow commands have not yet developed. In the newborn, symptoms are vague, including encephalopathy, seizures, hypotonia, poor feeding, and apnea. This list of symptoms may be present in many of the diseases treated in the neonatal intensive care unit; therefore, trying to ascertain which are caused by stroke can be immensely challenging. The correct identification of stroke, however, is paramount for many reasons, ranging from identifying potentially modifiable risk factors to acute therapeutic intervention (particularly for sinus venous thrombosis). Managing long-term outcomes, which tend to be poor, is difficult but may improve with aggressive early intervention and emerging therapies such as transcranial magnetic stimulation and constraint-induced therapy.
In neonates, a proposed definition of stroke is as follows: “A group of heterogeneous conditions in which there is a focal disruption of cerebral blood flow secondary to arterial or venous thrombosis or embolization, between 20 weeks of fetal life through the 28th post-natal day, and confirmed by neuroimaging or neuropathological studies.”3 In this definition, there is a conspicuous absence of any clinical sign or physical exam finding suggesting neurologic dysfunction caused by stroke. As in adults, stroke in neonates can be subcategorized based on the affected vascular distribution (arterial vs venous), by whether the primary mechanism is ischemic or hemorrhagic, and by the presumed timing of the event (prenatal or postnatal). In this chapter, arterial ischemic stroke is discussed first, followed by venous stroke (better known as cerebral venous sinus thrombosis, CVST), and then hemorrhagic stroke. Prenatal and perinatal ischemic events defined by the term presumed perinatal ischemic stroke (PPIS) are also discussed.
PERINATAL ARTERIAL ISCHEMIC STROKE
Definitions
Arterial ischemic stroke occurs when an arterial vessel is occluded by a thrombus or an embolus, resulting in ischemic injury to the brain tissue distal to the occlusion. A thrombus is defined as a clot that is adherent to the wall of a vessel, whereas an embolus is a nonadherent thrombus. The embolus may be moving within arterial vessels or venous vessels or may become paradoxical by crossing from venous to arterial circulation. Paradoxical emboli may be more likely to occur in the neonate as right-to-left shunts are a necessary part of cardiovascular physiology during the transition from fetal to neonatal circulation.
Epidemiology
Perinatal arterial ischemic stroke (PAIS) is often a single lesion (70%) and is frequently located in the anterior circulation (71%).4 A large international cohort revealed that there is a predominance of left-sided strokes affecting the middle cerebral artery (MCA) when only 1 hemisphere is involved (51% for isolated left vs 25% for isolated right) and that bilateral strokes occur in approximately 24% of patients. The left-sided predominance often leads to language deficits and right hemiparesis.4 Seizures are a common presenting symptom and are often focal in manifestation.5 Mortality was estimated in 1 study as 18 of 134 or 13.4% (8 died of hemorrhagic stroke, 9 died from arterial ischemic stroke, and 1 died from asphyxia and sinus thrombosis).6
Pathophysiology
Perinatal arterial ischemic stroke is dominated by necrosis. After 24 hours, activated microglia invade the lesion. They become foamy macrophages in 36–48 hours, and astrocytic hypertrophy develops over 3–5 days. Over the longer term, the lesion becomes gliotic as glial fibrillary processes are laid down. There may be mineralization or cavity formation over the next weeks to months. After 4 to 6 weeks, cystic evolution occurs, leading frequently to ex vacuo dilation of the ipsilateral ventricle. The ischemic penumbra is the area surrounding the focal ischemic area destined for necrosis. In the penumbra, cellular metabolism is deranged because of decreased cerebral blood flow.
Risk factors for PAIS (see Figure 14-1) can be grouped according to maternal, fetal, or placental origin; naturally, there is great overlap among the 3 categories. Pregnancy itself is a hypercoagulable state because of innate protective thrombophilic factors that come into play to protect the mother from postpartum hemorrhage. In fact, this is true to such an extent that the maternal risk of stroke in the 3 days postpartum is elevated 34-fold when compared to the nonpregnant state.1 In a normal pregnancy, there is a marked increase in the procoagulant activity in the maternal blood, characterized by elevation of factors V, VII, VIII, IX, X, and XII and fibrinogen (increases 2-fold) and von Willebrand factor, which is maximal around term.7 In addition, there is a decrease in protein S activity and an acquired activated protein C resistance, leading to less-effective thrombolysis.7 Other maternal factors increasing stroke risk include preeclampsia3; eclampsia; hemolysis, elevated liver enzyme levels, and low platelets (HELLP syndrome); elevated homocysteine and 677 C → T mutation in MTHFR gene; factor V Leiden mutation; chorioamnionitis; drug exposure (cocaine or amphetamine with associated vasospasm and poor perfusion of placenta); primiparity with a prolonged second stage of labor8; and autoimmune diseases such as lupus with associated autoantibodies. Fetal risk factors include birth-related trauma, which may result in stretch injury of carotid or vertebral arteries, leading to dissection, thrombus formation, and subsequent stroke. Congenital cardiac disease may lead to embolic stroke from thrombus that may occur in the heart because of diminished flow or the presence of right to left shunts. Cardiac surgery,9 atrial septostomy,10 and the presence of catheters11 have been shown to increase the risk of stroke. Infection, such as meningitis, increases the risk of stroke. Interestingly, there is a male predominance among children with stroke, and in neonates in the International Pediatric Stroke Study (IPSS) registry, there were 249 with PAIS, 149 (57%) of whom were boys.12
FIGURE 14-1 Risk factors associated with perinatal stroke. Hypercoagulable states and infection affect maternal, fetal, and placental categories. HELLP, hemolysis, elevated liver enzyme levels, and low platelets.
The placenta is a low-flow system, which makes it more susceptible to clotting because of stasis effects. Thrombotic lesions are often found in the placenta of those neonates diagnosed with stroke. In a paper dedicated to the role of placental pathology in neonatal stroke, Elbers described abnormal placental pathology in 10 of 12 patients with neonatal stroke (7 with arterial ischemic stroke and 5 with CVST). In these patients, 50% demonstrated thromboinflammatory processes. A further 42% demonstrated acute catastrophic events, and 25% demonstrated decreased placental reserve. Two patients had evidence of chorioamnionitis,13 suggesting that infection also plays an important role.
Clinical Presentation
Seizures are the most common sign of PAIS. Seizures commonly occur in the first hours or days of life and are focal and clonic5 (see Figure 14-2). A focal seizure in a neonate who is not encephalopathic is highly suggestive of PAIS. Stroke, however, can also be the etiology for severe encephalopathy to the extent that it is no longer uncommon to provide therapeutic hypothermia to an encephalopathic infant and later discover evidence of stroke on imaging rather than global injury caused by hypoxic ischemic encephalopathy. In a prospective cohort of 124 encephalopathic neonates, 6 (5%) were found to have focal stroke on imaging.14
FIGURE 14-2 Conventional electroencephalogram (EEG) demonstrating a typical focal seizure. This is a 2-Hz occipital seizure on the right (P4-O2 and P8-O2 leads; see arrows) that was associated with a posterior cerebral artery infarct (see magnetic resonance image).
Recognition of seizures can be challenging because of the variable clinical presentation of seizures, the complete lack of clinical correlate with many neonatal seizures, the dissociation between electrographic events and clinical events that occurs frequently after seizures are treated, and the frequent use of paralytic or sedating agents, especially during therapeutic hypothermia. A subclinical seizure is defined as one that occurs in the absence of any overt clinical repetitive or rhythmic movement that would be readily identified as seizure. In a study of 41 neonates, there were a total of 293 electrographic seizures (seizure apparent on electroencephalogram [EEG] only), 84 of which had a clinical correlate,15 thus illustrating the fact that many neonatal seizures have no clinically visible features. In another study, 51 neonates at high risk for seizure were monitored with EEG, and 12 had a total of 526 seizures. Of these 526 electrographic seizures, only 179 or 34% had clinically recognizable symptoms when the video and EEG were reviewed simultaneously. Of the 179 seizures with clinical correlate, only 48 were recognized as seizures by care providers. Conversely, there were 129 clinical seizure-like events documented by providers that had no EEG correlate. In other words, 73% of the time, seizure-like events were incorrectly identified at the bedside as seizures.16 This study clearly illustrates the need to monitor with EEG not only to correctly identify true seizures but also to limit the incorrect identification of seizure-like events and inappropriate administration of anticonvulsant drugs.
Another challenge to the recognition of seizures is that treatment with anticonvulsants can cause “electroclinical dissociation.” This condition exists after administration of a drug such as phenobarbital, which may lead to the cessation of clinical seizures while electrographic seizures continue. Last, there is the unanswered question regarding whether aggressive treatment of subclinical seizures has any positive impact on developmental outcome or whether overtreatment of movements incorrectly perceived as seizures has potential adverse effect on neurodevelopmental outcome.
Because of the challenges of correctly identifying seizures, EEG monitoring should be used to identify seizures, monitor for electroclinical dissociation, and monitor the effectiveness of treatment. Conventional EEG (cEEG) is preferred because full coverage of the head using the 10- to 20-electrode placement system yields more accurate identification of the area of brain from which the seizure originates. However, cEEG is of limited utility at the bedside for non-neurology-based personnel because of the difficulty of interpreting the complex waveforms. Limited channel-monitoring devices, such as amplitude-integrated EEG (aEEG), are being used as an adjunct or in place of cEEG to improve bedside seizure detection. Although short seizures, those lasting less than 30 seconds, or those of low voltage will be missed by aEEG,17 it is unclear at this time whether treating these seizures is imperative or improves outcome. aEEG is capable of detecting status epilepticus, and there is no ambiguity about whether prolonged status is deleterious to the newborn brain.18 aEEG is easily employed by trained personnel, and if used in conjunction with cEEG, artifacts that appear seizure-like can be readily evaluated with great accuracy. The other advantage to aEEG is the ability to comment on prognosis based on the background activity, which is reassuring when there is evidence of state change in hypoxic-injured term newborns in the first 24–48 hours.19
Differential Diagnosis
The differential diagnosis (see Table 14-1) for PAIS and CVST is essentially the same as for neonatal encephalopathy, a broad term used to describe a heterogeneous syndrome characterized by neurological dysfunction in the neonate. Hypoxic ischemic encephalopathy should be considered foremost for this differential diagnosis, and because of the significant overlap of symptoms, it is not uncommon for neonates with PAIS or CVST to be treated with therapeutic hypothermia. Any form of intracranial hemorrhage, including intraventricular (IVH), subarachnoid (SAH), subdural (SDH), or intraparenchymal hemorrhage (IPH), may have symptoms similar to PAIS or CVST (especially seizures and depressed mental status). Bedside cranial ultrasound can be used to quickly assess for the presence of blood. Trauma (incidental because of birth or inflicted) must not be overlooked as a potential etiology for intracranial hemorrhage and stroke.20 Central nervous system infection, whether bacterial or viral (encephalitis or meningitis), may also present similarly with altered mental status and seizures and should prompt consideration of stroke. Lumbar puncture is a necessary and frequent component of the workup for these infants once imaging has been performed and the risk of herniation is deemed minimal. Hypoglycemia and electrolyte derangements (sodium, calcium, and magnesium) are treatable causes of neonatal seizures and thus should be ruled out. More uncommonly, brain tumors may present in the neonate with symptoms of neonatal encephalopathy and seizure. Mitochondrial disease, other metabolic disorders, and kernicterus should also be considered as part of the wider differential diagnosis when there is persistence of seizures and progressive alterations in mental status.
Table 14-1 Differential Diagnosis of Stroke
• Neonatal encephalopathy (hypoxic ischemic encephalopathy)
• Intracranial hemorrhage
– Intraventricular, subarachnoid, subdural, or intraparenchymal hemorrhage
• Cerebral venous sinus thrombosis
• Central nervous system infection
– Meningitis (bacterial or viral)
– Encephalitis
• Electrolyte derangement
– Hyponatremia
– Hypocalcemia
– Hypomagnesemia
• Hypoglycemia
• Metabolic diseases
– Mitochondrial disease
– Inborn errors of metabolism
– Kernicterus
• Tumors
• Trauma
Diagnostic Tests
Imaging
Cranial ultrasound has a low likelihood of diagnosing PAIS.21 Computed tomography (CT) is another option, but there is higher risk to the neonate because of the significant dose of ionizing radiation,22 and CT may not be sensitive for infarcts that are less than 24 hours old.21 The advantages of CT, however, are the relative speed of image acquisition (several minutes) as opposed to upward of 30 minutes for magnetic resonance imaging (MRI) and could be of utility in the unstable neonate for whom redirection of care is a consideration. CT is particularly sensitive at detecting intracranial blood, but this can be achieved equally well with MRI or even with ultrasound.
The gold standard for diagnosing PAIS is MRI. This provides the highest-resolution detail regarding the extent and timing of injury. It is essential to have a basic comprehension of diffusion sequences and conventional T1 and T2 sequences when evaluating stroke. In the first 1–14 days of life (maximal in the normothermic patient by day of life 4),23 diffusion-weighted images (DWIs) depict areas of “diffusion restriction” as bright with “high signal intensity” compared to the surrounding noninjured brain tissue (see Figure 14-3, images A and E). Areas with restricted diffusion are areas where there has been injury resulting in energy depletion and failure of molecular pump machinery with subsequent decreased movement or “diffusion” of water molecules in the extracellular compartment. DWIs are compared to an apparent diffusion coefficient (ADC) map, where the areas of injury on the DWIs have areas of corresponding darkness (see Figure 14-3, images B and F). After approximately 10 to 14 days, these diffusion changes normalize, and the injury is no longer apparent on these sequences. Care must be taken with interpretation of diffusion images as the extent of injury may appear greater than what is ultimately seen on the conventional images. Pseudonormalization occurs 7 to 8 days after the injury; therefore, caution must be used in the interpretation of diffusion imaging as the absence of high signal intensity could represent the absence of injury or equally that the study was performed after day 7 or 8 when the diffusion changes are no longer apparent.24
FIGURE 14-3 Diffusion-weighted and conventional magnetic resonance imaging (MRI) images of entire middle cerebral artery (MCA) distribution stroke on the left and partial MCA stroke on the right. A, hyperintensity in the left MCA distribution indicates acute MCA stroke confirmed by corresponding darkness on the apparent diffusion coefficient (ADC) map in image B. The T1 sequence shown on C reveals subtle changes in the affected area that are more prominent in the T2 sequence shown on image D (loss of cortical ribbon; see arrows). Images E (diffusion weighted) and F (ADC map) demonstrate a partial MCA territory stroke with corresponding T1 and T2 images again demonstrating subtle loss of the cortical ribbon on T1 and more apparent loss on T2 (see arrows).
The conventional T1- and T2-weighted images are useful to clarify the injury because these abnormalities should be visible on these sequences by day 7 or 8. On T1 images in the neonate, the white matter appears gray, the gray matter appears white, and the cerebral spinal fluid (CSF) is dark. Both injured cortex and injured white matter appear relatively hypointense or have “low signal intensity” (see Figure 14-3, images C and G) compared to normal tissue and, most important, lose the distinct boundary delineating gray vs white matter (blurring of the cortical ribbon). This change is evident from day 2 onward until the injured area undergoes cystic degeneration, at which point it appears dark like CSF. On T2-weighted images in the neonate, the white matter appears white, the gray matter appears gray, and the CSF is bright. Injured areas, including both cortex and white matter, appear relatively hyperintense or have “high signal intensity” compared to uninjured tissue, and the loss of the cortical ribbon is more evident than on T1 (see Figure 14-3, images D and H). This change can be appreciated from day 2 onward and is eventually replaced with bright CSF signal as the injured area undergoes cystic degeneration.
Magnetic resonance angiography (MRA) permits the evaluation of the intracranial and neck arterial vasculature. This technique is sensitive to motion artifact but may be useful to reveal underlying vessel pathology, such as dissection from birth trauma or arterial malformation. MRI and MRA are often performed with anesthesia to improve image quality by sedating the neonate so there is no movement. Wrapping techniques have been developed that employ vacuum devices to immobilize the unsedated neonate (see Figure 14-4),25 thus eliminating risk of sedation with minimal to no sacrifice of image quality.
FIGURE 14-4 Transporting the unsedated neonate. In the upper left corner, the recently fed, sleepy, and unsedated neonate has ear protection applied and then is wrapped snugly in a blanket. The blue vacuum device is then wrapped around the neonate and secured with straps. A wall suction device is then used to remove the air from the vacuum device and the neonate is immobilized. The final image shows the neonate in the appropriate size head coil ready for magnetic resonance imaging (MRI). (From Mathur et al.22)
Management
Currently, the acute management of neonatal stroke is predominantly supportive. In adult stroke, normothermia, euglycemia, and permissive hypertension are accepted principles. Hyperthermia in animal models is known to increase metabolic demands if there is diminished capacity to deliver both oxygen and nutrients, leading to additional neuronal injury. In adults with stroke, hyperthermia is associated with a poorer prognosis, and in children with traumatic brain injury, hyperthermia is associated with longer hospitalization and lower Glasgow Coma Scale score.26 Hyperthermia has not been specifically studied in children or neonates with stroke, but based on the known adverse outcomes in adults, normothermia is a reasonable goal in the management of neonatal stroke. In the subset of encephalopathic neonates who receive therapeutic hypothermia and are subsequently found to have PAIS, one study has shown a decrease in the frequency of seizures (0/5 cooled neonates had seizures vs 7/10 with seizures who were not cooled)27 and thus suggests at least a need for future investigation into hypothermia as an intentional treatment for PAIS. Likewise, this study also suggests that treating seizures may decrease secondary neuronal injury. There is currently no evidenced-based approach to guide seizure treatment in the neonate; however, consensus seems to exist that status epilepticus (either clinical or subclinical) merits aggressive intervention18 and, depending on the institution, will often result in escalating doses of phenobarbital, followed by fosphenytoin. There is less clarity regarding the treatment of subclinical seizures, and ongoing research efforts are under way to assess this.
In terms of euglycemia, neonates do not tend to have the same elevations in serum glucose as adults because of the absence of comorbid diabetes and hyperglycemia; therefore, this tends to be less of an issue. It is important, however, to avoid hypoglycemia as symptomatic hypoglycemia in term newborns has been shown on early MRI to have an association with white matter, cortex, basal ganglia, posterior limb of the internal capsule, and thalamic injury.28 It is justified, then, to make a theoretical leap and assume that hypoglycemia can conceivably worsen an existing injury caused by PAIS and should therefore be avoided.
Adjunctive treatments currently investigated for hypoxic ischemic encephalopathy include xenon gas, erythropoeitin, and prophylactic use of anticonvulsant drugs, including phenobarbital, levetiracetam, and topiramate, but these are not yet being studied clinically for PAIS. Neonates are not usually treated with aspirin or antiplatelet drugs. On a case-by-case basis, particular disorders of hypercoagulability or cardiac diseases with large clot burden have been treated with either low molecular weight heparin (LMWH) or heparin drip. In one report, 3 neonates were discovered to have aortic arch thrombosis and were successfully treated with LMWH.29
With respect to the workup of neonatal stroke, it is essential to obtain MRI of the brain and vasculature and is reasonable to consider echocardiogram in the context of congenital heart disease and to perform Doppler ultrasound to evaluate flow. The hypercoagulable workup may be deferred from the acute setting, in which values are often reflective of the pregnancy state rather than intrinsic abnormalities of the neonate. The mother, especially in a first pregnancy, should have a hypercoagulable workup that includes examination of antiphospholipid antibodies and anticardiolipin antibodies. The neonate as part of outpatient follow-up can receive routine testing, including for antithrombin III, protein C, protein S, activated protein C resistance, factor V Leiden mutation, prothrombin gene mutation (G20210A), and antiphospholipid antibodies.
Outcome and Follow-up
Cerebral palsy (CP) is defined as a static, predominantly motor, deficit that results in spasticity in the extremities because of upper motor neuron injury of the cortical spinal tract. Of 36 infants with arterial stroke, 58% had CP,30 and other estimates of motor deficit after stroke range from 30% to 40%.31
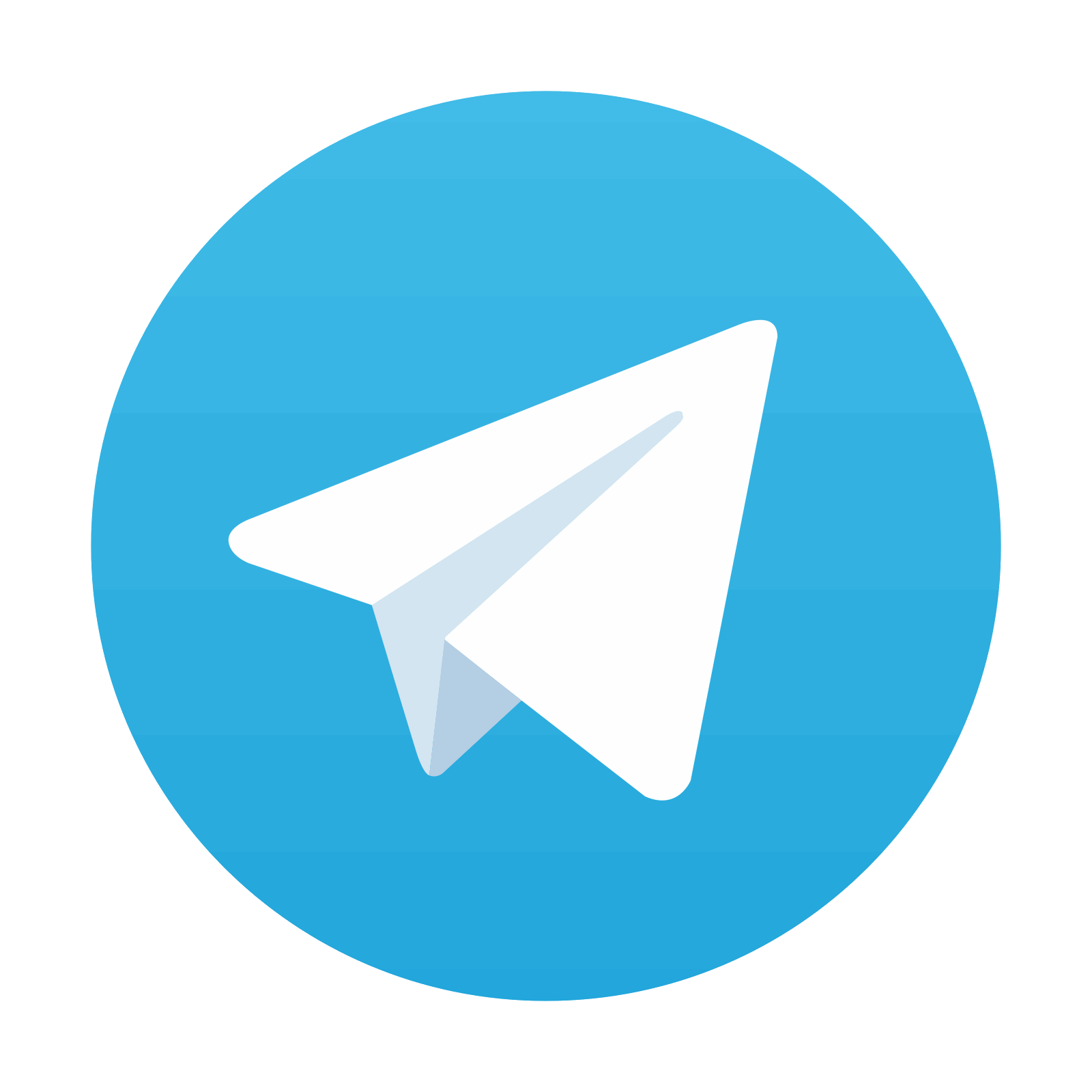
Stay updated, free articles. Join our Telegram channel

Full access? Get Clinical Tree
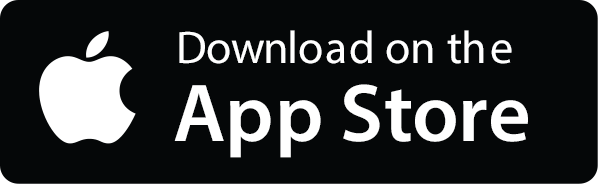
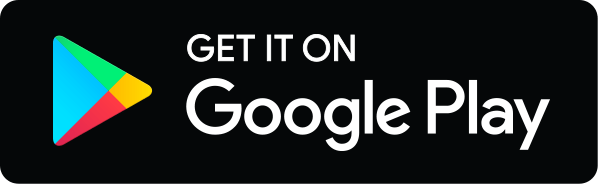