Noninvasive Support of Respiratory Failure
INTRODUCTION
Despite its clear association with lung injury, mechanical ventilation (MV) continues to be widely practiced in many neonatal intensive care units (NICUs). Recent data suggest that trauma to the respiratory system can be avoided by gentle respiratory support initiated soon after birth. Accordingly, in the past decade there has been increasing interest in using noninvasive respiratory support with nasal continuous positive airway pressure (CPAP) or nasal intermittent positive pressure ventilation (NIPPV) for managing respiratory failure in infants, with the focus to reduce the use of MV and protect the lung. Nasal CPAP therapy has been the mainstay of noninvasive respiratory support. However, the literature reflects conflicting results on the effectiveness of nasal CPAP in infants with respiratory failure because of inconsistencies in the guidelines for CPAP therapy, use of different devices and ventilator modes, and variations in training and levels of experience with nasal CPAP use. Little attention is paid to the best ways of practicing nasal CPAP therapy.
This chapter discusses the physiological effects, indications for use, application aspects, weaning strategies, complications, and controversies of nasal CPAP therapy based on the firsthand personal experience using binasal prongs CPAP for over 3 decades. These management protocols1 have been practiced successfully in our NICU for nearly 40 years and have been shown to reduce chronic lung disease in preterm infants without increasing morbidity or mortality. They have been increasingly adopted and practiced at many NICUs throughout the world with good results. We also provide an overview of some of the other types of noninvasive respiratory support strategies used in newborn infants.
NASAL CPAP THERAPY
The mainstay nasal CPAP is a particular form of noninvasive respiratory support that applies positive pressure to the airway of a spontaneously breathing patient throughout the respiratory cycle.
Physiological Effects
1. Increases transpulmonary pressure and functional residual capacity (FRC) and improves lung compliance.
2. Prevents alveolar collapse (atelectrauma), decreases intrapulmonary shunt, and provides progressive alveolar recruitment.
3. Conserves surfactant.
4. Prevents pharyngeal wall collapse.
5. Stabilizes the chest wall and decreases thoracoabdominal asynchrony and work of breathing.2
6. Increases airway diameter and splints the airways.
7. Splints the diaphragm.
8. Stimulates lung growth.3
9. Bubble CPAP adds high-frequency ventilation4 and stochastic resonance effects.5
Excessive CPAP pressure may lead to serious consequences, such as air leak syndromes and increased dead space ventilation, leading to a rise in PaCO2. Furthermore, high levels of CPAP can increase intrathoracic pressure, resulting in diminished venous return to the heart and reduced cardiac output, decreased pulmonary perfusion, and enhanced ventilation-perfusion mismatch.
Indications for Nasal CPAP Therapy
Evidence has accumulated over the past 4 decades to support the use of nasal CPAP not only to facilitate weaning in intubated infants but also as a primary mode of support in infants with various types of respiratory compromise situations, including the following:
1. Diseases with low FRC, such as respiratory distress syndrome (RDS), transient tachypnea of the newborn, patent ductus arteriosus, pulmonary edema
2. Apnea and bradycardia of prematurity
3. Meconium aspiration syndrome
4. Airway closure disease (eg, bronchiolitis, bronchopulmonary dysplasia [BPD])
5. Tracheomalacia
6. Partial paralysis of diaphragm
7. Respiratory support after extubation
Early initiation of CPAP (preferably before fetal lung fluid is absorbed) in preterm infants helps prevent alveolar collapse by continuously applying positive pressure and allowing these infants to produce endogenous surfactant. Spain and colleagues6 studied the amount and redistribution of lung surfactant during the perinatal period in rats and concluded that packaging of surfactant may be active immediately postbirth. Within 10 minutes of the initiation of air breathing, small increases in type II cell lamellar body content are observed. By 24 hours, the whole lung and alveolar extracellular pool surfactant lipid increases substantially. In our experience since 1973, early application of nasal CPAP therapy, usually within 5 minutes of life, in spontaneously breathing preterm infants prevents alveolar collapse, allows infants to breathe and produce adequate endogenous surfactant, decreases work of breathing, and helps lower the fraction of inspired oxygen (FiO2). More than 77% of infants 1250 g or less at birth do not require intubation or surfactant replacement therapy.7 The extended use of CPAP can also stimulate the growth of the premature lung3 and further decrease the incidence of BPD. Thus, CPAP is both a corrective and a supportive therapy that help the process of gas exchange while allowing the premature lungs to grow.
CPAP Delivery Devices
A good CPAP delivery system should include a patient system that is easily and rapidly applicable to very low birth weight (VLBW) infants; is readily removable and reconnectable; causes the least trauma to the infant; is capable of producing stable desired pressure levels; readily accepts humidification and supplementary oxygen at a desired temperature; is associated with low resistance to breathing; offers minimal dead space; is easily understood, maintained, and sterilized; and is safe and cost effective. Given the infrequent use of nonnasal methods of CPAP in current clinical practice, this review focuses exclusively on nasal interfaces and modes of pressure generation utilized in nasal CPAP delivery systems.
Essentially, a CPAP delivery system consists of 3 components:
1. Circuit for continuous flow of inspired gases: Oxygen and compressed air sources provide inspired gases at the desired FiO2. The flowmeter controls the rate of flow. The minimum flow rate used should be sufficient to prevent rebreathing of carbon dioxide, for instance, usually 2.5 times the infant’s minute ventilation. The flow should also compensate for leaks around connectors and the CPAP prongs. Excessive flow through the circuit will generate high pressure against which the infant must exhale. Usually, a flow rate of 5–10 L/min is sufficient in infants. The inspired gases are warmed and humidified prior to delivery to the infant.
2. Nasal interface to connect the CPAP circuit to the infant’s airway: Single and binasal tubes/prongs of varying lengths, ending in the nares or nasopharynx, have been used as nasal interfaces. Nasal masks were an early means of applying CPAP to infants. However, they lost popularity because of the difficulty in maintaining an adequate seal and keeping them in place. Nasal cannulas are often used in infants to deliver supplemental oxygen at low flows (<0.5 L/min) with no intention of generating CPAP. Binasal prongs, when first introduced to apply CPAP, were felt to be simple to use, effective, and safe, but they can potentially cause nasal trauma. A number of binasal devices are now in common use, including Hudson prongs1 and Argyle prongs.8
Efforts were directed at designing a nasal interface that would lower work of breathing. The resultant short-pronged binasal devices, currently known as Infant Flow (EME Medical Inc) and Arabella Generators (Hamilton Medical), are structured to allow the jet flow to flip between inspiratory and expiratory routes (coanal effect). They aim to provide sufficient demand flow on inspiration while minimizing expiratory resistance. Work with lung models and a small study on preterm infants with minimal lung disease9 demonstrated reduced work of breathing when compared with conventional devices. Limited randomized crossover and nonrandomized clinical studies, in preterm infants, have compared the Infant Flow nasal CPAP system with single-prong nasal CPAP. They found no significant difference in short-term measurement of physiological variables. Prongs inserted to the nasopharyngeal level have also been shown to deliver effective CPAP.10 They received early criticism because they were perceived to be poorly tolerated, difficult to insert, and increased resistance when compared with short nasal tube insertion. Despite these shortcomings, the use of nasopharyngeal tubes became established in clinical practice and featured in several trials that examined both binasal and single forms. In common with nasoendotracheal tubes, nasal CPAP interfaces have the potential to cause nasal excoriation and scarring if inappropriately applied or infrequently monitored.11
3. Modes of positive pressure generation in the CPAP circuit: The CPAP pressure is usually provided by varying the resistance to flow in expired tubing. This can be achieved by several techniques. In variable pressure-flow resistors, the level of peak end-expiratory pressure (PEEP)/CPAP is directly proportional to the product of the gas flow through the orifice of the expiratory pressure valve and the set resistance of the expiratory valve of the ventilator. The CPAP pressure varies with the infant’s respiration. With threshold resistors, the CPAP level is determined by the force applied to the surface area of the valve. During bubble CPAP, the expiratory limb is vented through an underwater seal. The pressure generated varies with the depth of submersion of expiratory tubing and is independent of the infant’s respiration.
Bubble CPAP is appealing because of its simplicity and low cost and has remained in use since first devised in the early 1970s. It has also been suggested that the bubbling creates pressure oscillations in the circuit and helps with removal of carbon dioxide.4 Using an artificial lung and sheep models, Pillow and colleagues5 demonstrated that decreasing compliance of the lung increased the magnitude of pressure oscillations in the model lung. These data suggest that use of bubble CPAP in the poorly compliant lung may promote lung volume recruitment (stochastic resonance) and augment the efficiency of gas mixing.
In a more recent study, Pillow and colleagues12 compared differences in gas exchange physiology and lung injury resulting from treatment of RDS with either bubble or constant-pressure CPAP. In this comparison with ventilator-derived CPAP, bubble CPAP was associated with a higher pH, PaO2 (arterial partial pressure of oxygen), oxygen uptake, and area under the flow volume curve. In addition, animals placed on bubble CPAP had decreased alveolar protein (a marker of lessened injury) and a lower PaCO2 (arterial partial pressure of carbon dioxide). The better oxygenation and more efficient removal of carbon dioxide are consistent with enhanced patency of peripheral airways and a greater surface area available for exchange.
The modes of pressure generation can be varied independently of nasal interfaces. There are multiple nasal CPAP delivery systems. Not all devices are similar, and success with nasal CPAP may be device specific. Further studies need to focus on the most effective nasal CPAP interface and the best mode of pressure generation for the delivery of nasal CPAP. Unfortunately, most of the CPAP studies do not show the device used. The short binasal curved prongs (Hudson CPAP prongs by Hudson-RCI, Temecula, CA) shown in Figures 88-1 and 88-2 were created for simplicity and minimizing trauma and have been successfully used at Columbia University Medical Center1 since 1973 in over 20,000 infants.
FIGURE 88-1
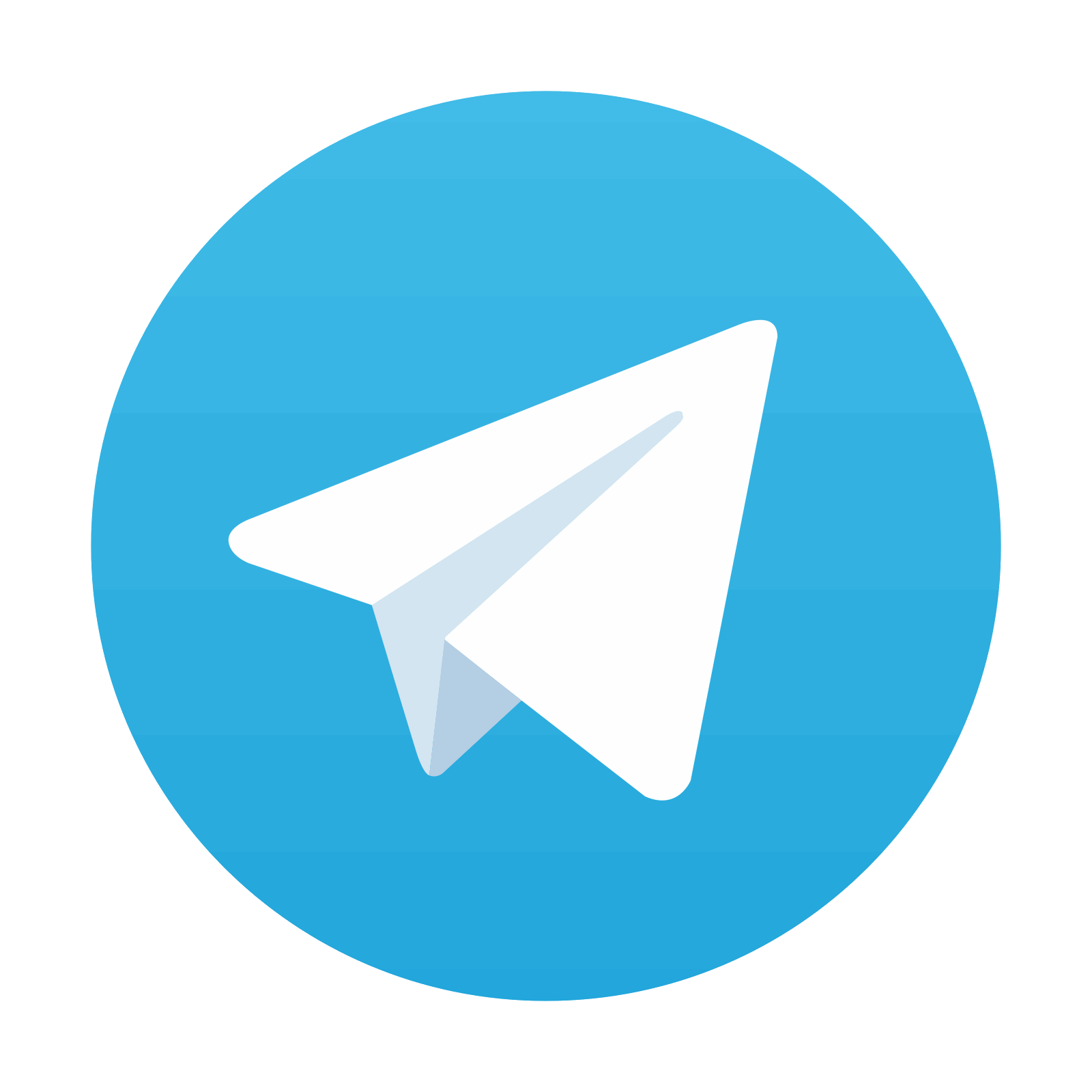
Stay updated, free articles. Join our Telegram channel

Full access? Get Clinical Tree
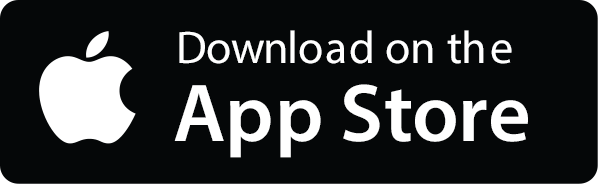
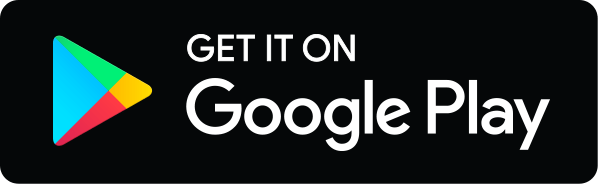