Local and Regional Anesthesia
Audra McCreight
Maria Stephan
Introduction
Local anesthesia is a useful adjunct in the treatment of pediatric patients with a variety of injuries and illnesses. Local anesthetics can be administered by tissue infiltration, by topical application, and/or for regional (nerve block) anesthesia. The unique properties of these agents allow physicians and other health care providers to safely and effectively reduce the patient’s discomfort, often without the complications associated with the more time-consuming process of sedation. However, successful administration of local anesthetics is dependent on a basic understanding of their pharmacology and, in the case of regional anesthesia, familiarity with the local anatomy of the nerve and its associated landmarks (1). For the sake of clarity, certain types of nerve blocks are discussed elsewhere in this text. The auricular block of the external ear is described in Chapter 55, and nerve blocks of the oral and facial areas are described in Chapter 62.
General Concepts
Anesthetic Structure
Local anesthetics are classified by their chemical structure and linkage. This basic chemical structure is: aromatic segment–intermediate chain–hydrophilic segment (2,3,4,5).
The most commonly used anesthetics are aminoesters and aminoamides (2,3,5,6). In common parlance, these agents are often referred to as simply “esters” and “amides,” and these terms are used to denote aminoesters and aminoamides throughout the remainder of this chapter. Procaine, cocaine, chloroprocaine, and tetracaine are examples of esters, and lidocaine, bupivacaine, prilocaine, mepivacaine, and etidocaine are amides. Drugs from the amide group are used more frequently for pediatric patients. The ester and amide local anesthetics differ in their chemical stability, locus of biotransformation, and antigenic potential. Amides are extremely stable, whereas esters are relatively unstable in solution.
The aromatic moiety of the anesthetic molecule contributes to its lipid solubility: 2-6-dimethylanaline for amides and p-aminobenzoic acid for esters. Changes in the structure of the aromatic portion have effects on lipid solubility and pKa (5,7). Combining them with hydrogen chloride to form the salt of a weak acid solubilizes local anesthetics. The proportion of local anesthetic in the ionized form is determined by its pKa and the pH of the solution. Neutral or alkaline pH favors the nonionized form, which is more lipid soluble and therefore better able to penetrate nerve membranes. Differences in the intermediate chain of the local anesthetic molecule determine its metabolism and clearance (2,3,5,7).
Ester and amide local anesthetics also differ in their allergic potential. Ester-linked local anesthetics (e.g., cocaine, tetracaine) are quickly hydrolyzed by plasma pseudocholinesterase and are excreted in the urine. Patients with pseudocholinesterase deficiency—those sensitive to succinylcholine, those taking cholinesterase inhibitors, and those with myasthenia gravis—are therefore more susceptible to toxic overdose of ester anesthetics (8). Cocaine, an ester, is an exception in that it is also metabolized by hepatic carboxylesterase (9). Esters also undergo degradation to the metabolite para-aminobenzoic acid, which is often responsible for producing allergic reactions (10). Amide-linked local anesthetics (e.g., lidocaine, mepivacaine, bupivacaine, ropivacaine) are conjugated in the liver by microsomal enzymes and are excreted in the urine. Unlike ester agents, the amide anesthetics rarely are associated with allergic reactions (11).
Molecular Action
When placed in proximity to nerve membranes, local anesthetic agents produce a transient and reversible blockade of
neural impulses (12). Recent data show that local anesthetics act to displace calcium ions at an internal receptor site of the sodium channel in the nerve cell membrane. This displacement of calcium ions stabilizes cell membrane permeability to the flow of sodium ions into the cell, slowing the rate of depolarization such that the threshold potential is not reached (13). By inhibiting the influx of sodium ions through selective ion channels, local anesthetics prevent the initiation and propagation of an action potential (10). Inhibition of nerve conduction in the region of local anesthetic injection causes a temporary loss of sensation that dissipates with time as the local anesthetic molecules are released from the sodium channel (14).
neural impulses (12). Recent data show that local anesthetics act to displace calcium ions at an internal receptor site of the sodium channel in the nerve cell membrane. This displacement of calcium ions stabilizes cell membrane permeability to the flow of sodium ions into the cell, slowing the rate of depolarization such that the threshold potential is not reached (13). By inhibiting the influx of sodium ions through selective ion channels, local anesthetics prevent the initiation and propagation of an action potential (10). Inhibition of nerve conduction in the region of local anesthetic injection causes a temporary loss of sensation that dissipates with time as the local anesthetic molecules are released from the sodium channel (14).
The sodium channel plays a central role in the mechanism by which local anesthetics block action potentials along the nerve axons. The nerve axon is surrounded by a membrane consisting of lipid and protein molecules (15), which control the passage of small ions such as potassium and sodium. An electrostatic gradient (16,17) is generated by the sodium-potassium ATPase pump, with high concentrations of intracellular potassium and extracellular sodium and chloride. A resting potential of between ∼70 to ∼90 mV is thereby maintained.
When a nerve impulse is generated, depolarization occurs through a complex process in which membrane proteins (“gates”) become permeable to sodium and potassium. As sodium enters the cell, the transmembrane potential reaches a threshold, and an action potential is propagated (2,15,18,19). Repolarization occurs as sodium and potassium ions are actively transported by the sodium ATPase pump, re-establishing the resting potential. Local anesthetics interact with the membrane protein gates and prevent sodium entry into the cell, although the precise mechanism of this interaction is not currently known. Without an influx of sodium, the electrochemical gradient does not reach the threshold potential, and no action potential is generated. This effectively blocks transmission of impulses from sensory receptors to the central nervous system (CNS).
Nerve Structure
A nerve fiber is composed of the axon and its surrounding Schwann cell sheath. A single Schwann cell may surround several unmyelinated nerve fibers (15). Myelinated nerve fibers often have one Schwann cell wrapped around a single axon. Myelin greatly increases the speed of nerve conduction by insulating the axolemma from the surrounding conducting salt medium and forcing the action current to flow through the axoplasm to the nodes of Ranvier, which are junctions between the sheaths along the axon (20). These myelin-deficient junctions are the sites where sodium channels necessary for depolarization are located. The nodes are more excitable than the rest of the membrane, allowing the impulse to skip from node to node and increasing conduction velocity. As the diameter of the axon increases, the nodes of Ranvier are spaced farther apart.
Nerve fibers can be classified into three types: A, B, and C fibers (3,7,15,17,21). The diameter and degree of myelination of the nerve predict its sensitivity to local anesthetics (21). With subcutaneous infiltration of local anesthetic, the smallest, unmyelinated fibers are affected first, and the larger, myelinated fibers are affected last. Consequently, thick, myelinated type A nerve fibers (motor, proprioception, reflex, pressure, and touch) are less readily blocked than thin, unmyelinated type C fibers (pain and temperature) (3,4,22). Because pain sensation typically is conveyed on smaller, unmyelinated nerves, and motor impulses are carried on larger, myelinated nerves, a predictable pattern of loss of function occurs. The sensation of pain disappears first, followed by the ability to sense temperature, touch, proprioception, and, finally, skeletal muscle tone (12). By using an appropriate dose of anesthetic, it is therefore possible to completely block pain sensation while preserving full motor function. This can be advantageous, as with the repair of facial lacerations, because the patient can follow motor commands despite anesthesia to sensory stimuli. Anxious patients may interpret any sensation as failure of the anesthetic; therefore, the physician should prepare the patient by explaining that the sensation of pressure may remain but not pain (8). The recovery of function occurs in reverse as the local anesthetic dissipates (i.e., motor function returns before pain sensation). A variety of factors determine the final concentration of anesthetic presented to the nerve membrane, including dilution of the anesthetic by tissue fluid, fibrous tissue barriers, uptake by surrounding fatty areas, and systemic absorption (2,3,17).
Anesthetic Effectiveness
The effectiveness of a local anesthetic is influenced by various aspects of the physiologic environment and by its inherent chemical activity, which largely determines its onset of action, potency, and duration. Diffusion of local anesthetics (and therefore, their potency) depends primarily on lipid solubility. Local anesthetics with greater inherent lipid solubility are generally more potent. Hydrophobic effects are also important, because the anesthetic molecule must penetrate the nerve membrane and bind at a partially hydrophobic site on the Na+ channel (23,24). The nonionized form is more lipid soluble. The ratio of ionized to nonionized forms depends on both the pKa of the parent molecule and the pH of the solution, as described by the Henderson-Hasselbach equation (1):
pH = pKa log (ionized form/nonionized form)
Local anesthetics are commercially available as water-soluble salts in an acidic solution, which primarily contains the cationic form of the drug (21,25,26). Raising the pH by adding sodium bicarbonate before administration increases the proportion of molecules in the nonionized, more lipid-soluble form, which speeds the onset of action (2,21,27). The rate and extent of diffusion also depend on molecular weight and concentration (2,4,5). Additionally, the site of administration plays an important role. As the amount of surrounding tissue or the size of the nerve sheath increases, the onset is delayed because of the greater distance the drug must travel to reach its receptor (2,4,7).
Protein binding of the anesthetic is an important factor in determining the duration of action. Binding reduces the amount of free drug available to diffuse into nerves directly (2,5,7,16,21). Agents that bind more tightly to the protein receptor remain in the sodium channel longer (18). Vasodilation produced by local anesthetics inversely influences the potency and duration of action (4,5). Local blood flow can remove the drug from the area before it is bound in nerve tissue and other binding sites. Vasoconstriction decreases this “washout” effect, allowing more molecules to remain at the peripheral nerve. In this way, epinephrine added to the local anesthetic solution can significantly increase the potency and duration of anesthesia (4,8,21,28,29). The duration also varies with the mode of administration and the dose. Topical application usually produces a shorter period of anesthesia than tissue infiltration. Increasing the concentration of anesthetic, while being cautious to avoid any toxic effects, can prolong the duration of action.
Important differences exist in the distribution and metabolism of local anesthetics in pediatric patients compared with adults. Infants have a larger volume of distribution, more rapid absorption, and lower plasma levels of albumin and α 1-glycoprotein (which binds lidocaine) (2,30). The activity of plasma pseudocholinesterase and microsomal enzyme systems in the liver is also low in this age group, resulting in a longer half-life of local anesthetics (30,31). Furthermore, the relatively increased cardiac output in young children leads to a more rapid peak plasma concentration. Because of the differences in pharmacokinetics, the toxic threshold in infants and toddlers is approximately one half that of children older than 5 years of age and adults (32). Special caution and reduced dosages are therefore warranted when using local anesthetics for these younger patients.
After the first few months of life, children have a greater clearance of local anesthetics than adults (30). Myelination is not complete until age 8 to 12 years. In laboratory studies, young animals are more sensitive to lidocaine, because they possess fewer myelinated type A fibers (17). The difference in the myelination of nerve fibers may account for the effectiveness of lower concentrations of local anesthetics in children.
Adverse Reactions
Systemic toxicity may occur by inadvertent intravascular injection or by using excessively large doses of local agents. After injection, peak blood levels are reached in 10 to 60 minutes. The more potent agents also are more toxic. Those with high lipid solubility and protein binding tend to become sequestered in tissue and have a slower rate of absorption, which produces a lower blood concentration. Esters are difficult to measure in blood due to their rapid hydrolysis by pseudocholinesterase. Toxic effects usually involve the CNS or the cardiovascular system. Local anesthetics are administered to inhibit conduction in the peripheral nerves, but any excitable nerve membrane (e.g., heart, brain, neuromuscular junction) may be affected if a higher drug concentration is reached (33).
Local anesthetics are lipophilic and cross the blood-brain barrier. As serum concentrations increase, patients initially complain of numbness of the lips and a metallic taste in the mouth. Nystagmus may follow, progressing to muscle twitching, tremors, seizures, and ultimately CNS depression and respiratory arrest at excessive concentrations (2,3,24,33,34,35,36). The blood-brain barrier is more permeable in infants than in adults, resulting in greater CNS concentrations of local anesthetic and therefore increased potential for complications (2). The cardiovascular system seems to be more resistant to toxic effects than the CNS. A decrease in blood pressure, however, may be observed secondary to the negative inotropic action of these agents, which can lead to a diminished cardiac output and stroke volume (2,3,21,33,34,37). Cocaine, which is rarely used now for topical anesthesia, produces its toxic effects on the cardiovascular system by inhibiting reuptake of catecholamines (epinephrine and norepinephrine) at adrenergic nerve endings, leading to increased blood pressure and/or ventricular dysrhythmias.
Allergic reactions to local anesthetics are uncommon. Most reports involve the esters which have a para-aminobenzoic acid nucleus (33,34). Reactions to amide anesthetics are rare. More often, methylparaben, a common preservative found in commercial preparations, causes allergic reactions that are subsequently (and often inappropriately) attributed the anesthetic agents themselves (33,38). Local anesthetics are available in a preservative-free preparation that avoids the aforementioned problems. Allergic reactions manifest as dermatitis, urticaria, anaphylaxis, pruritus, and bronchospasm. Small lacerations in allergic individuals may be repaired without local anesthesia or with normal saline infiltration. Diphenhydramine has also been used as a local anesthetic in a 1% solution (50 mg/mL diluted with 4 mL of normal saline to produce a 1% solution).
Tissue injury can result from the injection of anesthetic into a nerve or from passage of the needle. Neuropathy has been reported, especially with direct intraneuronal injection (39). In addition, inflammatory changes in muscle have been observed, but wound healing does not seem to be impaired (40,41). Tissue ischemia may result when epinephrine-containing solutions are injected in areas perfused by end arteries. Epinephrine administration is therefore contraindicated for use in digits, penis, nasal alae, and pinna of the ear. Local anesthetics do not affect the incidence of wound infection (42,43). Lidocaine has some antiseptic properties, but its presence has little effect on wound infection rates. If a wound is to be cultured, however, the swab should be obtained before local infiltration with lidocaine. Vasoconstrictors such as epinephrine have been associated with increased potential for infection in animal studies, but clinical studies in humans show no difference in actual rates of infection. Methemoglobinemia has been described with large doses of prilocaine and benzocaine (2). Toxicity is decreased by using an appropriate dose and route of administration as well as by administering vasoconstrictor-containing solutions (to prevent bolus absorption of large quantities of anesthetic) when not contraindicated. Contraindications to the use of regional anesthesia include (a) allergy to anesthetic agent, (b) infection
at injection site, (c) poor patient acceptance or cooperation, (d) coagulopathy, and (e) inadequate skill or knowledge on the part of the clinician (1).
at injection site, (c) poor patient acceptance or cooperation, (d) coagulopathy, and (e) inadequate skill or knowledge on the part of the clinician (1).
TABLE 35.1 Characteristics of Commonly Used Local Anesthetics | ||||||||||||||||||||||||||||||||||||||||||||||||||||||||||||||||||||||
---|---|---|---|---|---|---|---|---|---|---|---|---|---|---|---|---|---|---|---|---|---|---|---|---|---|---|---|---|---|---|---|---|---|---|---|---|---|---|---|---|---|---|---|---|---|---|---|---|---|---|---|---|---|---|---|---|---|---|---|---|---|---|---|---|---|---|---|---|---|---|
|
Selecting a Local Anesthetic
Although no local anesthetic is perfect in all respects, ideally a local anesthetic should (a) have a rapid onset of action and a long duration of action, (b) be nonirritating and nontoxic to tissues, (c) be effective both topically and by injection, (d) have a high therapeutic index and minimal undesirable side effects, (e) be stable in solution, (f) be water soluble, and (g) be heat stable for sterilization (1). The agents used for children that most closely approximate this optimal profile are lidocaine (Xylocaine) and procaine (Novocain), which account for approximately 90% of the use of local anesthetics. Plain lidocaine may be administered in doses up to 5 mg/kg, whereas the addition of epinephrine allows for a maximum dose of 7 mg/kg. Bupivacaine, a long-acting local anesthetic, is not approved by the U.S. Food and Drug Administration (FDA) for children under 12 years of age. Table 35.1 summarizes information on the most commonly used local anesthetics (3,21,34,38).
For increased safety in the use of local anesthetics for younger patients (less than 8 years of age), the following guidelines are recommended (44): (a) the dosage should be reduced to 80% of the maximal allowable dose; (b) the anesthetic should be administered slowly, in divided doses when possible; and (c) epinephrine (1:100,000 to 1:400,000) should be added if not contraindicated. As mentioned previously, the addition of epinephrine delays absorption but is contraindicated in areas where end arteries exist. For most procedures, it is unnecessary to choose a local anesthetic based on its onset of action. Simply waiting 5 to 10 minutes will generally obviate the need for additional local anesthetic.
Minimizing Pain with Injection
Rapid infiltration of an acidic solution of local anesthetic is painful. Decreasing the speed of infiltration can significantly reduce pain during injection (45,46,47). In addition, warming the local anesthetic to 40°C and using sodium bicarbonate as a buffer not only improves potency but also reduces the pain associated with infiltration (47,48,49). To buffer a local anesthetic solution, 1 part sodium bicarbonate is mixed with 10 parts anesthetic (by volume) in a syringe. Buffering an entire bottle reduces the shelf life and is not generally recommended (38). Likewise, because a precipitate is created, buffering of bupivacaine with sodium bicarbonate is contraindicated.
Infiltration Anesthesia
Wound infiltration is a safe, rapid, and easy method for providing anesthesia and hemostasis to a localized area. It is used for the majority of minor procedures (e.g., laceration repair, wound débridement, foreign body removal, abscess drainage). The two primary methods of infiltration of anesthetics are direct infiltration and parallel margin infiltration.
Direct infiltration involves the injection of an anesthetic agent directly into the tissues, and it results in the production of localized analgesia at the site of infiltration. Individual nerves are not specifically blocked but become anesthetized in the tissue planes surrounding the wound. The region is rendered painless by altering the perception of pain, although the sensation of touch may persist. Direct infiltration is the most commonly used technique for the administration of local anesthetic for laceration repair.
Parallel margin infiltration (field block) involves injecting adjacent tracts of anesthesia parallel to the wounded edge or surgical field. This results in the interruption of nerve impulses from the site to the CNS. As with direct infiltration, individual nerves are not sought specifically; they are anesthetized in the tissue plane in which they lie. The technique for this procedure is essentially the same as the technique for local infiltration except that the local anesthetic is injected circumferentially at a distance from the wound rather than into the wound margins. Field blocks are preferred for procedures in areas of inflammation or gross contamination. This method allows for the instillation of anesthetic away from acidic, infected areas, where local anesthetic is likely to have decreased effectiveness. Parallel margin infiltration also prevents the needle from carrying debris or bacteria from the wound into uncontaminated surrounding tissues. In addition, this method does not distort tissue planes that must be accurately
reapproximated to optimize the cosmetic result of laceration repair. It is therefore preferred over direct wound infiltration when preservation of the wound architecture is desired.
reapproximated to optimize the cosmetic result of laceration repair. It is therefore preferred over direct wound infiltration when preservation of the wound architecture is desired.
Anatomy and Physiology
The skin is composed of the epidermis, dermis, and superficial fascia. Free nerve endings that generate impulses from painful stimuli are plentiful in the dermis and epidermis. The preferred plane of injection is into the superficial fascia, immediately below the dermis, where nerve fibers transmitting painful stimuli are easily blocked by infiltration of local anesthetic. Insertion of the needle through the margin of the wound beneath the majority of free nerve endings is less painful than insertion through intact, densely innervated epidermis. Tissue resistance and pain from the instillation of anesthetic are also less in the subdermal tissue plane than in the epidermis or dermis (50).
As in direct wound infiltration, the plane of injection for a field block is the superficial fascia, just beneath the dermis. Proximal to the laceration, nerves (bundles of nerve fibers) can be blocked, producing a field of anesthesia distally. With parallel margin infiltration, the superficial fascial layer is approached through intact skin parallel to the wound or field edge. As a result, needle insertion with this technique is more painful than direct wound infiltration, because it passes through intact skin.
Indications
Direct wound infiltration is indicated for most wounds and lacerations. In highly contaminated wounds, injection through intact skin may be preferred as a means of preventing the introduction of organisms from the wound margin into the surrounding tissues.
Some tissues, such as the sole of the foot, are difficult to infiltrate because of the fibrous, septated subcutaneous tissue structure. In other areas, swelling and distortion of the anatomy with infiltration make it more difficult to accurately reapproximate tissue planes and optimize the cosmetic result. Nerve blocks described later in this chapter are often superior to infiltration anesthesia in such cases.
Indications for field blocks are (a) local anesthesia for procedures in areas of inflammation or infection (grossly contaminated lacerations, incision and drainage of abscesses, etc.) or (b) local anesthesia with preservation of the wound architecture.
Equipment
10% povidone-iodine solution
Sterile gauze
Sterile gloves
1% lidocaine (with or without epinephrine, depending on location) and 8.4% sodium bicarbonate (1 mEq/mL)
3-, 5-, or 10-mL syringe
27- or 30-gauge, 1.5-inch hypodermic needle
Procedure
Direct Infiltration
The region is checked for perfusion, sensation, motor function, and associated injuries before anesthetic injection. To minimize patient anxiety, the syringe is filled with warmed, buffered lidocaine out of view of the child or before the child enters the treatment room. Whenever possible, the child should not be allowed to see the needle. All materials should be ready to use before the start of the procedure. After an appropriate explanation of the procedure, infiltration should begin without delay. A sensitive, calm, and soothing approach that engages the child in conversation or distracts the child may avoid the need for sedation. The young child should be immobilized by either an assistant, a papoose restraint, or both (see Chapter 3).
The area surrounding the wound is cleansed with povidone-iodine solution and dried with sterile gauze. A few drops of anesthetic are instilled directly into the wound. A 27- or 30-gauge, 1.5-inch needle is inserted through the wounding opening into the subcutaneous tissue exposed by the laceration. Anesthetic solution is slowly injected as the needle is
advanced. Alternatively, the needle may be fully inserted and slow injection performed on withdrawal. Aspiration is generally necessary only in the vicinity of major vessels, as only a small amount of anesthetic is injected at any given point while the needle is continually advancing. The needle is removed and then reinserted into adjacent tissue through the previously anesthetized subdermis. Reinsertion and slow injection of anesthetic continues sequentially until the entire perimeter of the wound has been infiltrated. Optimal anesthetic effect is reached in less than 5 minutes. The needle can be lightly applied to the skin around the wound site to test for adequate wound anesthesia.
advanced. Alternatively, the needle may be fully inserted and slow injection performed on withdrawal. Aspiration is generally necessary only in the vicinity of major vessels, as only a small amount of anesthetic is injected at any given point while the needle is continually advancing. The needle is removed and then reinserted into adjacent tissue through the previously anesthetized subdermis. Reinsertion and slow injection of anesthetic continues sequentially until the entire perimeter of the wound has been infiltrated. Optimal anesthetic effect is reached in less than 5 minutes. The needle can be lightly applied to the skin around the wound site to test for adequate wound anesthesia.

Check region for perfusion, sensation, motor function, and associated injuries before anesthetic injection.
Explain procedure to parents and (as appropriate) to child, and keep needle out of view of child.
Restrain child if necessary.
Cleanse surrounding area with povidone-iodine solution and dry with sterile gauze.
If an open wound, instill a few drops of anesthetic directly into wound; then insert needle through margin of exposed subcutaneous tissue (rather than intact skin).
If not an open wound (e.g., foreign body removal) insert needle through adjacent intact skin into subcutaneous tissue.
Slowly inject anesthetic solution as needle is advanced, or insert needle completely and inject as it is withdrawn.
Remove needle and, if necessary, reinsert it into adjacent tissue through an area that has already been anesthetized.
Continue injecting tissue until entire area requiring anesthesia has been infiltrated.
After a few minutes, lightly apply needle to test for adequate anesthesia.
Parallel Margin Infiltration (Field Block)
Preparation for this technique up to the point of needle insertion is the same as for direct infiltration. Because this technique involves the injection of anesthetic through intact skin, the operator may choose to administer topical anesthesia (see below) prior to parallel margin infiltration. A 27- or 30-gauge, 1.5-inch needle is first inserted through the skin to the subcutaneous tissue 1 to 2 cm proximal to the laceration. Small amounts of anesthetic are injected as the needle is advanced to at least two thirds its entire length. Slow injection is continued as the needle is withdrawn from the insertion site. The needle is then reinserted at the end of the first wheal where the skin is anesthetized. In similar fashion, the injection is repeated until complete infiltration of the circumference has been achieved. Optimal local anesthesia is achieved in about 5 minutes.

Check region for perfusion, sensation, motor function, and associated injuries before anesthetic injection.
Explain procedure to parents and (as appropriate) to child, and keep needle out of view of child.
Restrain child if necessary.
Cleanse surrounding area with povidone-iodine solution and dry with sterile gauze.
Insert needle into skin proximal to laceration to level of subcutaneous tissue.
Inject small amounts of anesthetic as needle is advanced to at least two thirds its entire length.
Slowly inject anesthetic as needle is withdrawn.
Reinsert needle at end of first wheal where skin is anesthetized and inject anesthetic.
Continue injections until complete infiltration around circumference has been achieved.
After a few minutes, lightly apply needle to test for adequate anesthesia.

A longer needle allows for fewer needlesticks.
Slower injection minimizes pain.
Nerve blocks or general anesthesia, rather than infiltration anesthesia, prevents systemic lidocaine toxicity if lacerations are large.
Stabilizing the ends of a wound with the nondominant hand and bracing the syringe against the nondominant thumb helps control the injection with a struggling patient.
Aspiration is generally necessary only in the vicinity of major vessels, as only a small amount of anesthetic is injected while the needle is advancing.
Complications
Direct wound infiltration and parallel margin infiltration are generally safe procedures, as long as correct medication dosages are administered and careful technique is practiced. Complications of both techniques include infection, bleeding, and intravascular injection of anesthetic.
Topical Anesthesia
Topical anesthesia is an alternative to local infiltrative anesthesia. It is used to anesthetize certain small wounds and intact skin for simple procedures. Topical agents are often advantageous because they are easier and less painful to apply, they do not distort wound margins, and they can decrease the need for sedation and physical restraint of pediatric patients. There are, however, some important limitations to the use of topical anesthetics: they have a relatively slow onset of action, they will not provide adequate anesthesia in many cases, and the agents are somewhat costly. Finally, occasional local and systemic adverse reactions are associated with the use of topical medications.
Tetracaine-Adrenaline-Cocaine
Tetracaine-adrenaline-cocaine (TAC) is a clear solution of 0.5% tetracaine, 1:2000 epinephrine, and 11.8% cocaine. Its anesthetic efficacy was first described by Pryor et al. in 1980 (51). The use of TAC has decreased dramatically in recent years due to the development of other topical anesthetics that carry fewer adverse side effects, cost less, and do not have
appreciable abuse potential. Significant blood levels of cocaine have been detected and cocaine toxicity reported with its use (52). For the sake of completeness, however, its uses will be briefly described.
appreciable abuse potential. Significant blood levels of cocaine have been detected and cocaine toxicity reported with its use (52). For the sake of completeness, however, its uses will be briefly described.
TAC topical anesthesia is about as effective as lidocaine infiltration for anesthetizing pediatric facial and scalp lacerations. It is less effective on trunk and extremity lacerations and in adult patients (53,54). Topical application of TAC is advantageous because it is easy and painless and has been shown to improve patient compliance with the subsequent wound repair procedure (51,53,55). The psychological trauma associated with restraint and painful procedures is reduced because of the relative absence of pain. Additionally, topical application avoids the swelling and distortion of wound margins caused by infiltrative anesthesia. This may enhance accurate tissue approximation and cosmetic outcome. TAC does not appear to increase bacterial proliferation or wound infection rates when compared with lidocaine (56).
The major disadvantage of TAC is that its use is limited to highly vascularized, non-mucous-membrane regions. Even when applied to selected wounds, adequate anesthesia may not be achieved. However, if additional local anesthetic is required, subsequent lidocaine infiltration is often less painful after TAC administration. Another disadvantage is that TAC costs significantly more per patient dose than lidocaine and also incurs the additional indirect costs associated with using a Schedule II agent (54,57). Finally, the time required to achieve effective anesthesia is considerably longer than that for lidocaine infiltration.
Anatomy and Physiology
TAC causes local anesthesia by the diffusion of the topical anesthetic combination through the margins of lacerated tissues. Tetracaine hydrochloride is a long-acting ester group local anesthetic. It acts on the neuronal membrane, interacting with the sodium channel to prevent the generation and conduction of nerve impulses in axons of the peripheral nervous system (54). Cocaine hydrochloride, which is derived from the leaves of the Erythroxylon coca plant, is an ester group local anesthetic with vasoconstrictive properties. Cocaine induces anesthesia by preventing impulse generation and conduction in sensory nerves, and it also blocks the presynaptic reuptake of norepinephrine and other catecholamines. This process results in excess norepinephrine concentrations in the synaptic cleft and is responsible for the vasoconstrictive properties (54). Epinephrine, an endogenous catecholamine with vasoconstrictive effects, prolongs the duration of action of locally infiltrated anesthetics by retarding drug removal and limiting systemic absorption.
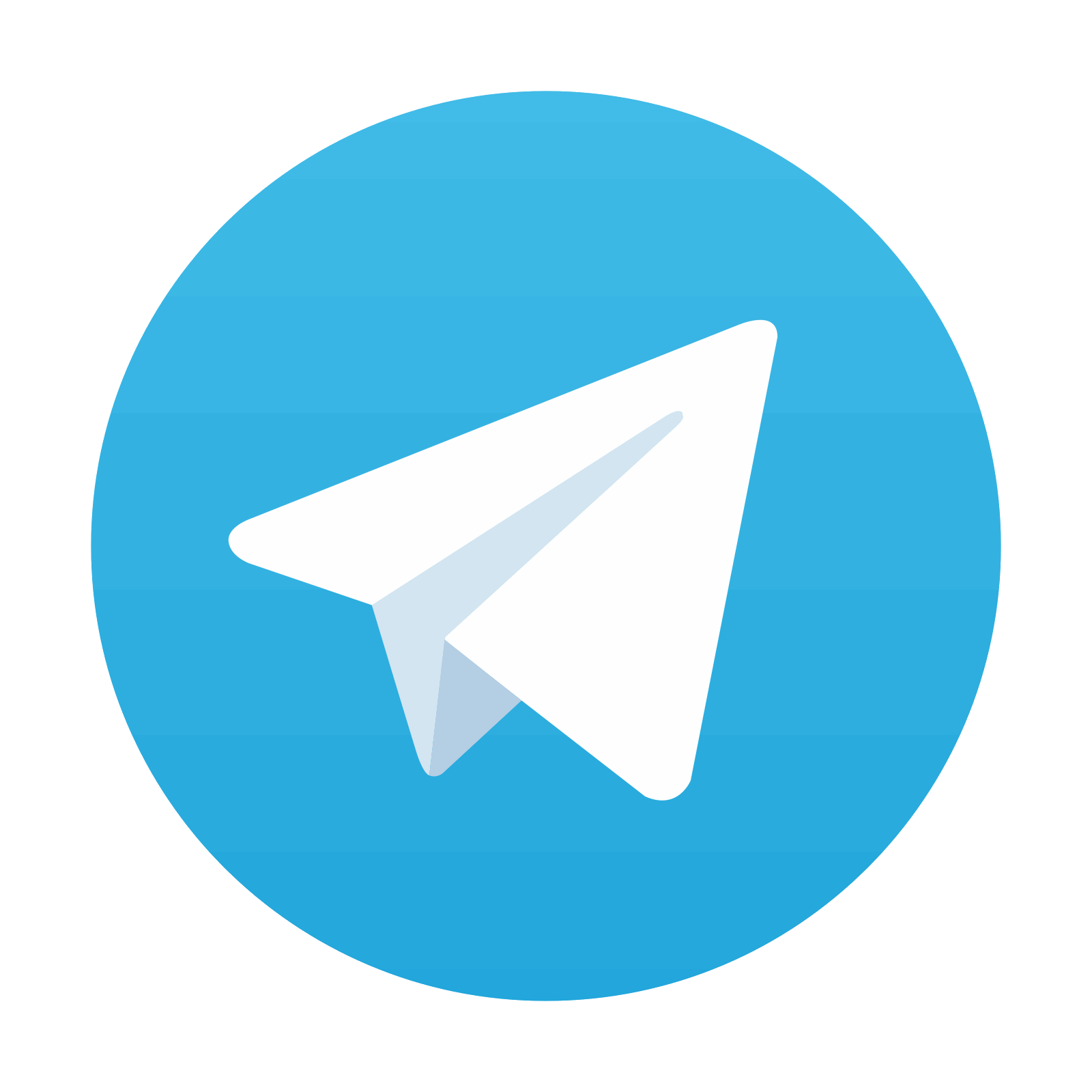
Stay updated, free articles. Join our Telegram channel

Full access? Get Clinical Tree
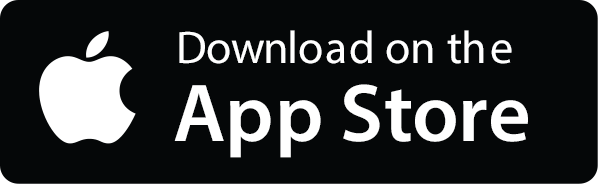
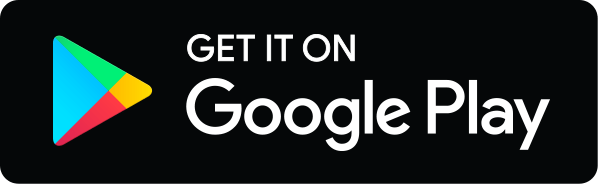
