Introduction to Conventional Mechanical Ventilation
Joseph W. Luria
Introduction
The appropriate use of mechanical ventilation is a requisite skill for all physicians caring for children who are critically ill. The first widespread use of ventilators in a nonoperative setting occurred during the polio epidemic of the 1950s. Since that time, ventilators and ventilation strategies have become more sophisticated and, for some, more challenging. Although ventilators may seem to be complex machines, a few basic principles govern their function. Once these principles are understood and applied correctly, ventilator management can proceed in an effective manner (1,2,3,4,5,6,7,8,9,10,11,12,13,14).
Anatomy and Physiology
The respiratory cycle consists of inspiration and exhalation. Inspiration is an active process initiated by contraction of the intercostal musculature and diaphragm. The actions of these muscles cause an expansion of the chest cavity, which generates a negative transpleural pressure. Air flows along the resultant pressure gradient (between the atmosphere and pleural space), through the airways, and into the lungs. Exhalation, which eliminates air after alveolar gas exchange, is largely a passive process due to the elastic recoil of the lung.
The two forces that affect airflow through the respiratory tree are compliance and resistance. Compliance represents the elasticity of the respiratory system and is defined as the unit change in lung volume per unit change in pressure. When respiratory compliance decreases, the lung is often described as being “stiff.” The lung and chest wall have separate compliances, and each contributes to the total compliance of the respiratory system. Adult respiratory distress syndrome (ARDS), neonatal RDS, and the presence of pulmonary edema are examples of clinical scenarios in which respiratory compliance is decreased.
Resistance is the impedance of airflow due to friction. It is defined as the unit change in pressure per unit change in gas flow. Respiratory resistance is greatest in the airways. Processes that cause a narrowing of the airways, such as asthma, will increase respiratory resistance.
Work of breathing is the amount of effort required to overcome both the respiratory compliance and resistance. Processes that decrease compliance or increase resistance will increase the total work of breathing. In these settings, patients will compensate by adopting a respiratory pattern that minimizes their work of breathing. Generally, acute lung processes that decrease compliance are associated with rapid, shallow breathing. In contrast, processes that increase respiratory resistance are associated with slow, deep breathing. These breathing patterns can be useful clinical clues when determining a patient’s underlying pathophysiology.
The volume of air that is moved into the lungs during a normal inspiration is termed the tidal volume. Normal tidal volume at all ages is 6 to 8 mL/kg. Functional residual capacity (FRC), or resting volume, is the amount of air remaining in the lungs at the end of a normal, quiet exhalation. FRC is determined by two opposing forces that are equal at the end of exhalation. These are the elastic properties of the lung and chest wall. At FRC, the elasticity of the lung exerts a force that favors a reduction in lung volume. The elastic force of the chest wall favors expansion. Processes that alter the relationship between these two forces will alter the FRC. Closing capacity is the volume of air in the lung below which small airways begin to collapse. Any disease state that increases the closing capacity or decreases the FRC can result in airway collapse and atelectasis. One objective of mechanical ventilation
is to optimize the relationship between closing capacity and FRC. For example, closing capacity occurs at greater lung volumes in premature infants with surfactant deficiency (RDS). The goal of positive pressure ventilation in this setting is to increase the FRC above closing capacity by using positive end-expiratory pressure (PEEP). This will prevent further atelectasis and assist in the re-expansion of collapsed segments of the lung. These lung volumes are illustrated in the spirogram in Figure 82.1.
is to optimize the relationship between closing capacity and FRC. For example, closing capacity occurs at greater lung volumes in premature infants with surfactant deficiency (RDS). The goal of positive pressure ventilation in this setting is to increase the FRC above closing capacity by using positive end-expiratory pressure (PEEP). This will prevent further atelectasis and assist in the re-expansion of collapsed segments of the lung. These lung volumes are illustrated in the spirogram in Figure 82.1.
The central nervous system (CNS) coordinates the actions of the respiratory muscles. The respiratory muscles are responsible for the bellows-like action that brings oxygen into the lungs and expels carbon dioxide. The lungs provide the interface for the transfer of oxygen into the blood and the removal of carbon dioxide. The circulatory system is responsible for delivering oxygen to the tissues and bringing carbon dioxide back to the lung for removal. In a simplistic manner, this describes the big picture of normal respiration. Processes that depress the CNS (e.g., traumatic brain injury, narcotic intoxication), weaken the respiratory musculature (e.g., Guillain-Barré syndrome, poliomyelitis, myopathies), or damage the lung or heart can lead to respiratory failure.
A large number of pulmonary and cardiac processes can result in respiratory failure. The end result of these processes is impaired pulmonary gas exchange with or without increased work of breathing. Impaired gas exchange results in the inability of the circulation to deliver adequate amounts of oxygen to peripheral tissues. To meet the oxygen demands of the body, minute ventilation and/or cardiac output must be increased. To accomplish this, the respiratory musculature and cardiac musculature are forced to work harder. This additional work of breathing increases the oxygen consumption of the cardiopulmonary system. To meet the higher oxygen demand, minute ventilation and/or cardiac output must be further increased. If the underlying insult is severe or is not corrected, this cycle will continue until demand cannot be met. Then it will not be possible to meet the oxygen requirements of the body, the respiratory musculature will fatigue, and respiratory failure will ensue. This concept as it pertains to specific pathophysiologic processes is discussed in the “Procedure” section later in this chapter.
Indications
Respiratory failure is the primary indication for initiating mechanical ventilation. Respiratory failure is characterized as the inability to maintain adequate oxygenation and/or ventilation despite using more conservative respiratory therapies. Traditionally, hypoxemic respiratory failure is defined as a PaO2 of less than 55 to 60 torr in the face of inspired oxygen concentrations of greater than 60%. Inadequate ventilation is defined as the presence of respiratory acidosis, an elevated PaO2, and an arterial pH of less than 7.2 to 7.25. These numbers are guidelines and should not be used as absolute values. The clinician, in deciding whether to begin mechanical ventilation, should consider the disease process present and its anticipated progression, the patient’s clinical assessment and response to other therapies, and the risks associated with mechanical ventilation.
Impending respiratory failure with or without increased work of breathing constitutes a relative indication for mechanical ventilation. It is always preferable to initiate mechanical ventilation under controlled conditions rather than to wait for worsening acidosis, exhaustion, or cardiorespiratory failure.
In some medical and surgical conditions, it may be best for a patient to assume a specific respiratory pattern. When this occurs, mechanical ventilation can be used to achieve this goal. The most common example of this is the need for hyperventilation in a patient with intracranial hypertension and lateralizing signs or impending herniation. Table 82.1 reviews these indications for initiating mechanical ventilation.
Mechanical ventilation may be an indicated treatment in a number of clinical diseases. Specific details of ventilator management are highly dependent on the disease state present
and its severity; therefore, specific pathophysiologic processes and how they relate to ventilator management are discussed in the “Procedure” section later in this chapter.
and its severity; therefore, specific pathophysiologic processes and how they relate to ventilator management are discussed in the “Procedure” section later in this chapter.
TABLE 82.1 Indications for initiating mechanical ventilation | ||
---|---|---|
|
Equipment
To use a ventilator effectively, it is important to have a basic understanding of how it works and a common vocabulary for describing its function. Similar to spontaneous respiration, mechanical breaths may be divided into four phases: transition from exhalation to inspiration, inspiration, transition from inspiration to exhalation, and exhalation. The goal of the operator is to select ventilator settings to perform these phases of respiration in a manner that is optimal for the patient.
Transition from exhalation to inhalation refers to the way in which a ventilator initiates a mechanical breath. For practical purposes, initiation of a mechanical breath can be controlled by either time or pressure. When a breath is initiated because a preset time has elapsed, the ventilation is said to be time triggered. A pressure-triggered breath is initiated in response to a spontaneous respiratory effort. Contraction of the respiratory musculature from a spontaneous breath results in a negative transpleural pressure. This negative pressure is transmitted through the respiratory tree to the endotracheal tube and into the ventilator circuit. The ventilator will sense this decrease in the circuit pressure, and a positive pressure breath will be delivered.
Once a mechanical breath has been initiated, the ventilator must deliver an effective tidal volume, which is accomplished by preselecting limits for the inspiration. For instance, if the inspiratory pressure reaches a constant value before inspiration ends, it is a pressure-limited breath. If a constant volume or flow rate is reached before the end of inspiration, the breath is volume or flow limited, respectively. Some ventilators also have the capability to select an inspiratory flow pattern (constant, sinusoidal, accelerating, or decelerating). Despite a number of studies, the data fail to demonstrate any advantage of one gas flow pattern over the others in all circumstances.
Cycling refers to the manner by which a ventilator transitions from inspiration to exhalation. If inspiration ceases after a preset time, the breath is said to be time cycled. Likewise, if inspiration ceases after a preset volume or inspiratory pressure is reached, the breath is volume or pressure cycled, respectively.
Exhalation proceeds after a valve is opened within the exhalatory limb of the ventilator circuit. As with normal respiration, exhalation is a passive process. During mechanical ventilation, the patient will remain in the exhalation phase until another breath is triggered.
Ventilators are described by the manner in which they operate during the first three phases of respiration. For example, an infant ventilator may be described as providing time-triggered, pressure-limited, and time-cycled breaths. Most clinicians, however, do not routinely refer to ventilator function in this manner. Rather, they refer to a particular mode of ventilation. Referring to a mode of ventilation is a shorthand method for describing how a ventilator performs the phases of the respiration. Modes of ventilation are selected based on the clinical situation.
Control Mode Ventilation
Control mode ventilation (CMV) is time triggered. Each breath is initiated at a preset time interval. By definition, the ventilator will not respond to the patient’s spontaneous respiratory efforts. CMV should be reserved for patients who are apneic. A patient who is breathing spontaneously would be uncomfortable on this mode of ventilation. CMV is not responsive to patient respiratory effort and gives breaths to the alert patient asynchronously with his or her spontaneous respiration.
Assist Mode Ventilation
In assist mode ventilation, mechanical breaths are initiated by the patient’s spontaneous respiratory effort. The ventilator accomplishes this by sensing a change in circuit pressure or gas flow (pressure or flow triggered). This mode allows the patient to “interact” with the ventilator. That is, the ventilator is responsive to the patient’s needs in a synchronous fashion. It may be used for fully awake intubated patients. The assist mode should not be used with apneic patients because no respiratory support would be afforded.
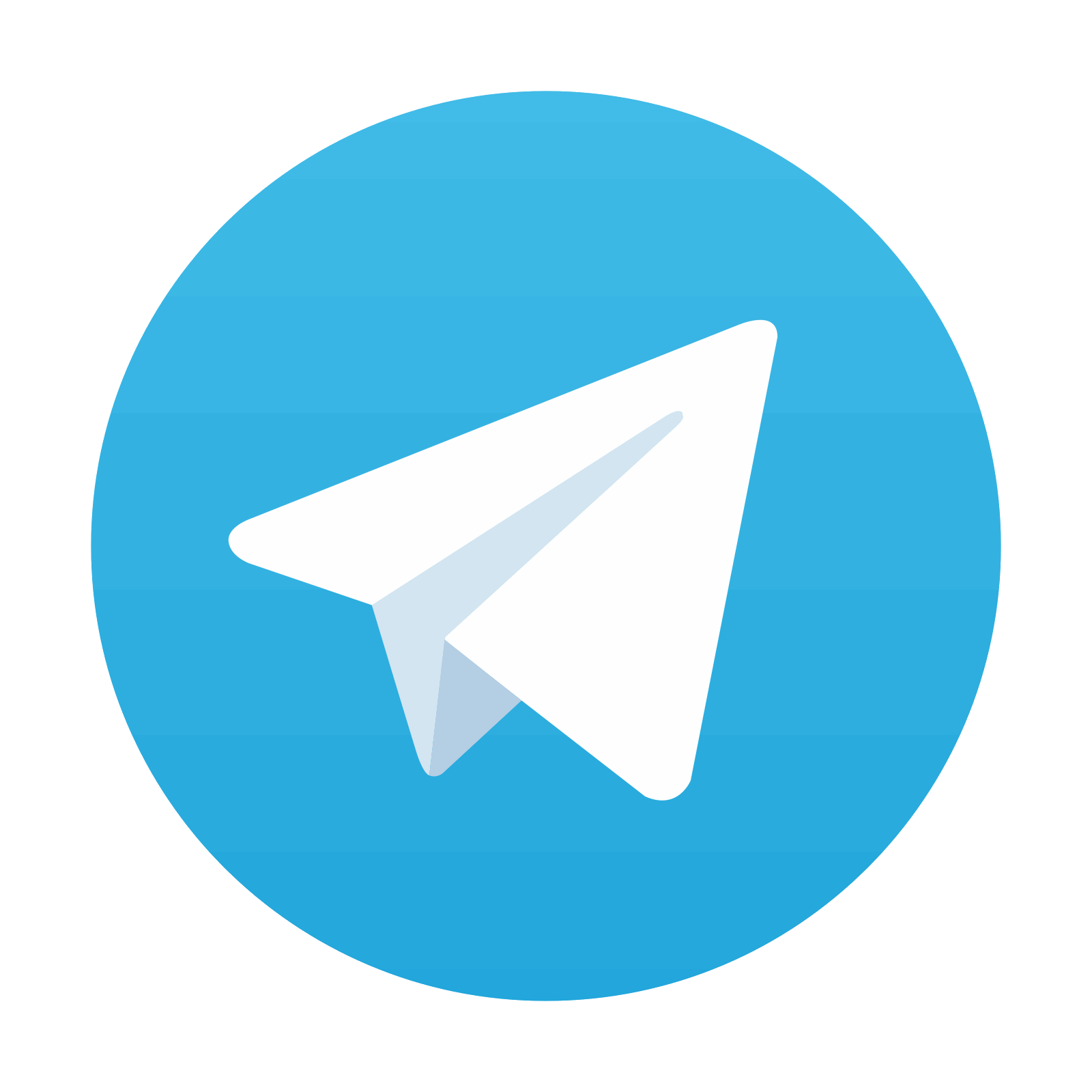
Stay updated, free articles. Join our Telegram channel

Full access? Get Clinical Tree
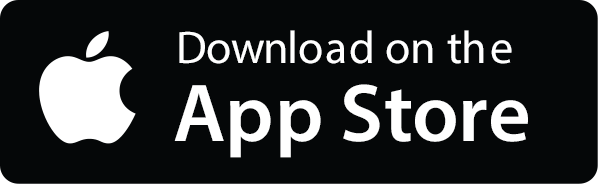
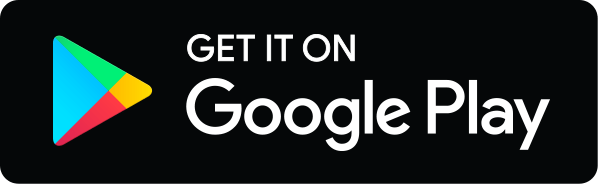