Inflammation
Jeffrey S. Upperman
Henri R. Ford
Department of Pediatric Surgery, Children’s Hospital of Pittsburgh, University of Pittsburgh, Pittsburgh, Pennsylvania 15260.
Department of Pediatric Surgery, Children’s Hospital of Pittsburgh, University of Pittsburgh, Pittsburgh, Pennsylvania 15260.
Inflammation is a highly regulated physiologic process that purports to restore host homeostasis. The inflammatory cascade is essential for effective host defense. It can be likened to a military engagement where “foreign” microbes invade the host, while the latter’s immune system responds or counteracts by launching a cadre of cellular and soluble factors consisting of neutrophils, macrophages, lymphocytes, fibroblasts, immunoglobulins, complement proteins, cytokines, oxidants, and other pro- and antiinflammatory molecules. Thus, an intact immune system is a prerequisite for survival of the species. Inflammation also plays a critical role in the normal healing process following tissue injury. In conjunction with anatomic or physical barriers such as skin, for instance, the immune system normally helps to prevent foreign organisms from breaching the epithelial barrier to reach the bloodstream or lymphatics. In addition, the immune system customarily aids in the removal of senescent cells, tumor cells, and devitalized host tissues. Acute inflammation can often result in collateral tissue injury; however, in most cases, the damage is minimal because of the complex and highly efficient immune system that has evolved.
Inflammatory Process.
In general, the initial stages of acute inflammation evoke little, if any, systemic response. Foreign antigen is recognized as nonself by the host immune system through specific and nonspecific sensing mechanisms. A specific response occurs when distinct T-cell receptors or preformed immunoglobulins interact with a particular foreign antigen. Nonspecific recognition and destruction of foreign antigen is mediated by natural killer (NK) or phagocytic cells and by the alternative pathway of complement activation. By necessity, these processes are highly conserved and tightly regulated because dysregulated inflammation could destroy the host.
Dysregulated Inflammation.
The distinction between an efficient host defense and pathological injury to host tissues can be extremely narrow. Unchecked, exuberant, or misdirected inflammatory responses can lead or contribute to systemic diseases. Indeed, dysregulated inflammatory responses play important roles in many contemporary pediatric surgical diseases, including sepsis, necrotizing enterocolitis, and meningitis, to name a few. The potential for endogenous inflammatory injury was first recognized when investigators discovered that serum sickness resulted from antigen-antibody–mediated tissue injury in the host. Subsequently, the fundamental importance of acute inflammation and its role in diverse diseases such as atherosclerosis, arthritis, acute respiratory distress syndrome (ARDS), multiorgan failure syndrome, compensatory antiinflammatory response syndrome, chronic lung disease, trauma, thermal injury, acute pancreatitis, ischemia-reperfusion injury, sepsis, and transplant rejection was demonstrated. More recently, the widespread use of antimicrobial agents has helped demonstrate that stimuli other than microbes can evoke a systemic inflammatory response. For instance, ARDS may result from a systemic inflammatory response to tissue injury without overt evidence of infection. The inflammatory response leads to leukocyte- or cytokine-mediated injury to the pulmonary microvasculature, resulting in interstitial edema, alveolar flooding, impaired gas exchange, and respiratory failure. As a result of this dysregulated inflammatory response, the host often suffers significant morbidity and mortality. Because the steps that govern these processes remain ill defined, therapeutic interventions are largely supportive. For example, in ARDS, the mortality rate remains 50% or higher in most reports and has not changed appreciably since its original description. Furthermore, specific therapy for ARDS has not been forthcoming, despite a substantial increase in our understanding of the mechanistic processes involved. Therefore, defining the aberrant molecular switches or networks that result in uncontrolled inflammation will be essential for
designing therapies that attenuate or reverse dysregulated inflammation.
designing therapies that attenuate or reverse dysregulated inflammation.
This chapter begins by providing an overview of the various components (cellular and soluble) of the immune system, with particular emphasis on nonspecific immunity. It concludes by examining how these components, in conjunction with other intrinsic host-defense mechanisms, interact to orchestrate the inflammatory response to microbial invasion or tissue injury. Other relevant aspects of the immune system, including transplantation, tumor immunology, specific infections, and autoimmune disease, are detailed elsewhere in this book.
INDIVIDUAL COMPONENTS OF THE IMMUNE SYSTEM
In the early twentieth century, Ehrlich and Metchnikoff were awarded Nobel prizes for recognizing the two basic components of the immune system: the humoral and cellular elements. In subsequent years, only incremental progress was made in understanding their complex and intricate interactions. During the last decade, however, modern molecular and cell biology techniques have yielded an enormous amount of data detailing the specific components of the immune system and the regulation of the immune or inflammatory response. For instance, redness (rubor), edema (tumor), heat (calor), and pain (dolor) were recognized as the cardinal signs of inflammation more than 2,000 years ago. We now understand that these clinical manifestations are the result of vasodilation with enhanced local blood flow, which causes redness and heat, and increased microvascular permeability, which leads to edema. Extravasation of plasma proteins and leukocyte recruitment to the site of injury generate pain.
Fundamental characteristics of the immune system include the ability to differentiate between self and nonself antigens using antibodies (immunoglobulins) and T-cell receptors. The immune response depends on fixed and circulating elements derived from the lymphoreticular system (Fig. 14-1). Fixed elements, which consist of the bone marrow, spleen, liver, lymph nodes, and thymus as well as specialized cells contained in the skin, gastrointestinal, respiratory, and urogenital tracts, supply and amplify the immune response. For example, neutrophils and platelets originate from the bone marrow and circulate to injured organs to support the local inflammatory response to trauma or to an antigenic insult. Circulating elements consist of cells that originate from fixed elements of the immune system. These include lymphocytes, granulocytes, monocytes, macrophages, erythrocytes, platelets, and mast cells.
Circulating cellular elements (Fig. 14-2) are derived from common progenitor cells that originate in the embryonic yolk sac. During the sixth week of human gestation, the fetal liver is populated with yolk sac-derived stem cells and becomes a site of hematopoietic activity. By the twelth week of gestation, the spleen begins to produce blood cells, and by the twentieth week of gestation, the thymus, lymph nodes, and bone marrow also become active
sites of hematopoiesis. It is at this time that the two major lymphocyte subsets, T and B cells, first become differentiated in the fetal spleen and lymph nodes. In addition to T or B cells, another subset of lymphocytes that lack both T- and B-cell markers appears; they are known as NK cells. (Table 14-1). At term, the bone marrow is normally the exclusive source of hematopoiesis.
sites of hematopoiesis. It is at this time that the two major lymphocyte subsets, T and B cells, first become differentiated in the fetal spleen and lymph nodes. In addition to T or B cells, another subset of lymphocytes that lack both T- and B-cell markers appears; they are known as NK cells. (Table 14-1). At term, the bone marrow is normally the exclusive source of hematopoiesis.
TABLE 14-1 Lymphocyte Subsets. | ||||||||||||||||
---|---|---|---|---|---|---|---|---|---|---|---|---|---|---|---|---|
|
Cellular Elements
T Lymphocytes
Bone marrow-derived lymphoid progenitor cells differentiate into T or B lymphocytes, depending on their site of maturation. A subset of these progenitor cells mature in the thymus where they acquire certain markers, known as clusters of differentiation or CD antigens, that comprise the entire T-cell receptor complex, CD3. T cells are further “educated” in the thymus by acquiring additional subsets of membrane receptors. Most notable are the CD8 cytotoxic/suppressor antigens, and the CD4 helper/inducer antigens, which functionally differentiate T-cell subsets. After maturation, T cells emigrate from the thymus to populate other “fixed” elements or peripheral lymphoid organs, such as the spleen, lymph nodes, intestinal Peyer’s patches, for instance, where they survey and combat foreign antigens (1). The process is illustrated schematically in Fig. 14-3.
B Lymphocytes
Lymphoid progenitor cells that undergo maturation in the bone marrow or the fetal liver are known as B lymphocytes. These cells undergo discrete stages of differentiation in the bone marrow and eventually complete the final stages of maturation in the periphery, where they specialize in immunoglobulin synthesis. The maturation stages include (1) stem cells; (2) pre-B cells; (3) immature B cell; (4) mature B cell; and (5) activated B cell. Stem cells are primitive B-cell precursors that reside in the bone marrow but do not produce immunoglobulins. They function solely as precursors. They give rise to antigen-unresponsive pre-B cells that produce cytoplasmic μ heavy chains. Pre-B cells in turn differentiate into immature B cells that produce membrane-bound IgM. Although immature B cells are sensitive to tolerance induction, they do not proliferate or differentiate in response to antigens. Tolerance to self-antigens is critical in the process of maturation of immature B cells in the bone marrow. The first truly antigen-sensitive B cell is the mature B cell. Mature B cells produce complete heavy or light chains after they come in contact with antigen. Once mature B cells commit to a particular set of heavy and light chains, they are unable to produce any different heavy or light chains during their lifetime. Consequently, the mature B cells containing distinct membrane-bound immunoglobulins such as IgM or IgD emigrate from the bone marrow to the peripheral lymphoid tissues, where they are poised to interact with a specific foreign antigen. Once the mature B cell comes in contact with a foreign antigen, it becomes activated. These cells proliferate and differentiate into plasma cells that secrete specific immunoglobulins. Some activated B cells do not secrete immunoglobulin in response to antigenic stimulation. They continue to have membrane-bound antibody and become memory cells. Memory B cells exposed to a specific antigen elicit a secondary antibody response that results in secretion of large quantities of highly specific immunoglobulins. As a population, B cells possess a large repertoire of antibody responses. Their extraordinary potential for clonal expansion is a fundamental aspect of the immune response and acquired immunity. These issues are summarized schematically in Fig. 14-3.
Natural Killer Cells
NK cells are lymphocytes that lack B- or T-cell markers. NK cells play an important role in the innate immune response to foreign antigens because they possess the ability to produce cytokines or chemokines and to kill specific target cells (2). The maturation of NK cells occurs in a fashion similar to that of T and B lymphocytes. NK cells are derived from lymphoid progenitor cells in the bone marrow. They migrate through the peripheral bloodstream and to specialized lymphoid components where they serve an
important role as effector cells. NK cells express the CD2 and FcγRIII (CD16) molecules on their surface. Cross-linking of CD2 or CD16 causes NK cells to proliferate or secrete cytokines. NK cells have the ability to kill tumor- and viral-infected cells; they do not require antigen recognition in order to kill. NK cells kill target cells by releasing cytotoxins, serine esterases, and proteoglycans. However, killing by NK cells is not a random process because they do not lyse all tumor cells or virally infected cells.
important role as effector cells. NK cells express the CD2 and FcγRIII (CD16) molecules on their surface. Cross-linking of CD2 or CD16 causes NK cells to proliferate or secrete cytokines. NK cells have the ability to kill tumor- and viral-infected cells; they do not require antigen recognition in order to kill. NK cells kill target cells by releasing cytotoxins, serine esterases, and proteoglycans. However, killing by NK cells is not a random process because they do not lyse all tumor cells or virally infected cells.
Monocytes/Macrophages
Monocytes and macrophages are essential effector cells for host defense and wound healing. The monocyte is derived from a granulocytic progenitor stem cell in the bone marrow. The latter gives rise to the monoblast, which becomes a promonocyte upon acquisition of specific granules. The promonocyte undergoes further differentiation in the bone marrow to become a monocyte. Monocytes enter the peripheral circulation under the regulation of several growth factors and cytokines, notably interleukin (IL)-1, IL-3, granulocyte-macrophage colony-stimulating factor (GM-CSF), and macrophage colony-stimulating factor (M-CSF) (3,4). Monocytes are characterized by the presence of immunoglobulin receptors and both class I and class II major histocompatibility complex (MHC) antigens on their cell surface. Their cytoplasm contains lysosomal granules that aid in the digestion of engulfed bacteria. Monocytes adhere to and traverse the microvascular endothelium to reach specific peripheral tissues, where they exert their specialized functions. This homing process may be part of the terminal differentiation pathway whereby circulating monocytes become tissue macrophages capable of antigen presentation and phagocytosis. Organ-specific differentiated macrophages include the Kupffer cells within the liver, microglial cells in the central nervous system (CNS), and Langerhans cells in the skin.
In response to foreign protein such as bacteria and viruses, the monocyte/macrophage secretes IL-1, tumor necrosis factor-α (TNF-α), and IL-6 (5). These cytokines
activate a variety of cells and induce acute-phase protein synthesis. In addition, the activated monocyte/macrophage secretes IL-12, which can help amplify the immune response and promote T-cell differentiation, and chemokines such as IL-8 and macrophage inflammatory peptides 1 and 2, which aid in the recruitment of additional inflammatory cells. The monocyte/macrophage also secretes IL-10, transforming growth factor-β (TGF-β), and soluble IL-1 receptor, which serve to attenuate the inflammatory process. Finally, it secretes platelet-derived growth factor, which, along with TGF-β, plays an important early role in the wound repair process (Fig. 14-4).
activate a variety of cells and induce acute-phase protein synthesis. In addition, the activated monocyte/macrophage secretes IL-12, which can help amplify the immune response and promote T-cell differentiation, and chemokines such as IL-8 and macrophage inflammatory peptides 1 and 2, which aid in the recruitment of additional inflammatory cells. The monocyte/macrophage also secretes IL-10, transforming growth factor-β (TGF-β), and soluble IL-1 receptor, which serve to attenuate the inflammatory process. Finally, it secretes platelet-derived growth factor, which, along with TGF-β, plays an important early role in the wound repair process (Fig. 14-4).
Neutrophils
Neutrophils are derived from pluripotent granulocytic stem cells in the bone marrow. More than 90% of the mature neutrophil population resides within the bone marrow. Their maturation and proliferation are under the control of several cytokines and growth factors (6,7); this process is detailed in Fig. 14-2. Their release from the bone marrow into the circulation is complex and incompletely understood.
Neutrophils are the first inflammatory cells to arrive at the site of injury. They are the primary cells responsible for phagocytosis in acute inflammation. As such, they play a fundamental role in the inflammatory process. They are equipped with a variety of surface ligands and receptors that are conduits for the regulatory signals that govern activation, chemotaxis, endothelial adherence, phagocytosis, and cell killing (Fig. 14-5 and Table 14-2) (6,7,8,9,10,11). Neutrophils sense increasing concentrations of chemotactic factors, such as complement, leukotrienes, and chemokines, in the wound microenvironment. These factors interact with receptors on the surface of neutrophils and promote the adherence of neutrophils to the endothelium. Neutrophils then migrate between the endothelial cells—a process called diapedesis. Once the neutrophils are in the extravascular space, they continue along the chemoattractant concentration gradient toward the inflammatory stimulus.
Neutrophils contain a variety of secretory products that react with pathogens and host tissues; proteases and cationic proteins are among the most important of these molecules. These products are stored in specific cytoplasmic granules (lysosomes) that do not take up
hematoxylin or eosin stains, hence, the neutrophil designation. When the neutrophil is activated, the lysosomes fuse with phagosomes containing the ingested particle or microbe to become a phagolysosome, or they are exported to the cell surface. Granule contents are targeted to intracellular or extracellular locations and destruction of the pathogen follows (Fig. 14-5A). Table 14-3 provides a partial inventory of neutrophil granule contents (12,13,14).
hematoxylin or eosin stains, hence, the neutrophil designation. When the neutrophil is activated, the lysosomes fuse with phagosomes containing the ingested particle or microbe to become a phagolysosome, or they are exported to the cell surface. Granule contents are targeted to intracellular or extracellular locations and destruction of the pathogen follows (Fig. 14-5A). Table 14-3 provides a partial inventory of neutrophil granule contents (12,13,14).
One of the key functional consequences of neutrophil activation is the generation of reactive oxygen products (Fig. 14-5B). These products include the superoxide anion (O2–), hydrogen peroxide (H2O2), hypochlorite (HOCl), and the hydroxyl radical (OH.). All are nascent, highly reactive free radicals. In addition, neutrophils produce chloramines, oxidants with relatively long biologic half-lives. Oxidants participate in numerous important biochemical interactions (15) (Fig. 14-5C), which include protein oxidation, DNA strand breakage, and membrane lipid peroxidation. These reactions contribute to the death of an invading pathogen or a targeted host cell. Oxidant injury by free radicals is a major mechanism for neutrophil-mediated cell killing. Significant synergy has been demonstrated between neutrophil-derived oxidants and proteinases (12). Oxidant- and proteinase-mediated killing of invading pathogens can also result in damage to host cells (16). Fortunately, however, regulation of this process is precise, and a variety of endogenous antiproteinases and antioxidants have evolved that are highly conserved
phylogenetically. Among the most important of these regulatory enzymes are α1-antiproteinase, α2-macroglobulin, superoxide dismutase, and catalase.
phylogenetically. Among the most important of these regulatory enzymes are α1-antiproteinase, α2-macroglobulin, superoxide dismutase, and catalase.
TABLE 14-2 Some Important Polymorphonuclear Neutrophil Receptors and Ligands. | |||||||||||||||||||||||||||||||||||||||||||||||||||||||||||||||||||||||||||||||||||||||
---|---|---|---|---|---|---|---|---|---|---|---|---|---|---|---|---|---|---|---|---|---|---|---|---|---|---|---|---|---|---|---|---|---|---|---|---|---|---|---|---|---|---|---|---|---|---|---|---|---|---|---|---|---|---|---|---|---|---|---|---|---|---|---|---|---|---|---|---|---|---|---|---|---|---|---|---|---|---|---|---|---|---|---|---|---|---|---|
|
Basophils/Eosinophils
Basophils and eosinophils are granulocytes derived from a common hematopoietic stem cell. IL-3 is the principal cytokine responsible for basophil growth and differentiation. Although early workers believed that basophils were circulating mast cells because of their histamine content, more recent evidence suggests that basophils and eosinophils are functionally similar.
Basophils constitute less than 1% of the circulating leukocyte population. They usually remain in the circulation. However, they may be found in the skin or airway after local antigen stimulation. Patients with active allergies or asthma have increased numbers of circulating basophils. Basophils have cell surface receptors for IgE, IgG, complement (C3aR, C5aR, CR1, CR3 p150,95), cytokines [IL-2, IL-8, monocyte chemoattractant protein, IL-3, IL-4, IL-5, GM-CSF, interferon (IFN)-γ, TNF-α], platelet-activating factor (PAF), and histamine (17). Histamine and proteoglycans reside within basophils as preformed mediators that are promptly released upon cell stimulation. In addition, basophils have an important capacity to synthesize and release leukotriene C4.
Eosinophil differentiation within the bone marrow is regulated principally by GM-CSF, IL-3, and IL-5. Once released from the bone marrow, eosinophils circulate briefly (13 to 18 hours) before their transvascular migration into peripheral tissues. Eosinophils are usually found in tissues exposed to the external environment, such as the skin, respiratory, gastrointestinal, and genitourinary tracts. Eosinophils have many receptors on their cell surfaces, including some for IgG (Fc), C1q, C3b/C4b (CR1),
C3bi (CR3), C5a, IgE, IL-3, IL-5, and GM-CSF. In addition, MHC class II proteins and CD4 are expressed on the surface of eosinophils. The presence of the CD4 receptor suggests that eosinophils can not only respond to lymphocyte chemoattractants, but also present antigen to T cells. Similar to other granulocytes, eosinophils possess a variety of proinflammatory proteins within cytoplasmic granules. Granule constituents include lysophospholipase, collagenase, and peroxidase. Eosinophils also produce a wide range of cytokines, including GM-CSF, IL-1, IL-3, IL-5, IL-6, and IL-8. In addition, eosinophils produce PAF, leukotriene C4, and leukotriene D4; all potent mediators of inflammation. Eosinophil activation may be induced by GM-CSF, IL-3, IL-5, TNF-α, IFN-γ, IFN-α, and PAF (18).
C3bi (CR3), C5a, IgE, IL-3, IL-5, and GM-CSF. In addition, MHC class II proteins and CD4 are expressed on the surface of eosinophils. The presence of the CD4 receptor suggests that eosinophils can not only respond to lymphocyte chemoattractants, but also present antigen to T cells. Similar to other granulocytes, eosinophils possess a variety of proinflammatory proteins within cytoplasmic granules. Granule constituents include lysophospholipase, collagenase, and peroxidase. Eosinophils also produce a wide range of cytokines, including GM-CSF, IL-1, IL-3, IL-5, IL-6, and IL-8. In addition, eosinophils produce PAF, leukotriene C4, and leukotriene D4; all potent mediators of inflammation. Eosinophil activation may be induced by GM-CSF, IL-3, IL-5, TNF-α, IFN-γ, IFN-α, and PAF (18).
TABLE 14-3 Partial Inventory of Neutrophil Granule Constituents.a | ||||||||||||||||||||||||||||||||||||||||||||||||||||||||||
---|---|---|---|---|---|---|---|---|---|---|---|---|---|---|---|---|---|---|---|---|---|---|---|---|---|---|---|---|---|---|---|---|---|---|---|---|---|---|---|---|---|---|---|---|---|---|---|---|---|---|---|---|---|---|---|---|---|---|
|
Mast Cells
Mast cells are derived from CD34+ pluripotent progenitor cells within the bone marrow. Their growth and maturation are regulated by several soluble factors. Mast cells usually comprise two distinct populations, depending on their location. Connective tissue mast cells are found in the skin and peritoneal cavity, whereas mucosal mast cells reside within the lamina propria of hollow viscera. Both subsets reach functional maturity in the peripheral tissues, but they differ in the types of surface proteoglycans they express and the types of proteinases found in their cytoplasmic granules. When activated, mast cells release preformed inflammatory mediators from intracellular granules, but also synthesize eicosanoids (notably prostaglandin D2, leukotriene B4, and leukotriene C4) and PAF. These mediators promote microvascular permeability, peripheral vasodilation, platelet aggregation, and leukocyte adherence. Pulmonary vasoconstriction, bronchoconstriction, and alveolar edema formation are important sequelae of mast cell degranulation in the lung. Mast cell products include histamine, proteoglycans, serine proteinases, and carboxypeptidase A. In addition, a wide range of cytokines, including TNF-α, IL-1, IL-2, IL-3, IL-4, IL-5, IL-6, GM-CSF, IFN-γ, and macrophage inflammatory proteins 1α and 1β are also elaborated by mast
cells. Tissue mast cells release serotonin when activated, which recruits other leukocytes (19).
cells. Tissue mast cells release serotonin when activated, which recruits other leukocytes (19).
TABLE 14-4 Platelet Granule Contents and Function. | ||||||||||||||||||||||||||||||||||||
---|---|---|---|---|---|---|---|---|---|---|---|---|---|---|---|---|---|---|---|---|---|---|---|---|---|---|---|---|---|---|---|---|---|---|---|---|
|
Unlike neutrophils, mast cells are capable of de novo protein synthesis and persist in the local microenvironment after degranulation. It is probable that they contribute to chronic inflammation in this manner. In addition, mast cells synthesize collagen, laminin, and heparan sulfate proteoglycans, which are essential structural components of the basement membrane. These observations suggest that mast cells play an important role in wound healing and tissue repair. The initial signal for fibroblast migration into a wound may be collagen synthesis and secretion by the mast cell.
Platelets
Platelets are anucleated, membrane-bound circulating elements that play an essential role in hemostasis, coagulation, and the inflammatory response. They are derived from megakaryocytes in the bone marrow and have a half-life of 8 to 10 days in the circulation. Like granulocytes, platelets store a variety of proteins in granules that regulate critical events such as leukocyte adherence, complement activation, microvascular tone and permeability, eicosanoid production, and chemotaxis and growth factor production (20) (Table 14-4).
Soluble Components of Inflammation
The previous section described the cellular components that play a crucial role in the complex immune response or inflammatory process. However, soluble components such as immunoglobulins, complement, and cytokines are responsible for initiating and optimizing the highly orchestrated cellular response.
Immunoglobulins
Immunoglobulins or antibodies represent a distinct set of proteins that are produced by mature B or plasma cells as a result of interaction between a T-helper cell and an antigen-presenting cell bearing an MHC and peptide complex (21). This communication between cells may lead to cytokine synthesis, B-cell proliferation and maturation and, most important, the production of a distinct class of immunoglobulin. The chief function of antibodies in host defense is to defend the host against microbes by preventing bacterial adherence to and invasion of vulnerable host cells. The mechanisms crucial to this process include opsonization of the microbe to enhance phagocytosis, complement activation with deposition of complement fragments on bacterial membranes to further facilitate phagocytosis, and subsequent bacterial killing and neutralization of microbial toxins or virulence factors to interrupt bacterial attachment to cell surfaces. There are five principal isotypes: IgG, IgA, IgM, IgD, and IgE. Each immunoglobulin subclass is composed of two identical light and two identical heavy polypeptide chains. There are two light chain isotypes, κ and γ, and five heavy chain isotypes: α, δ, σ, γ, and μ. Immunoglobulins are classified according to their heavy chains because the latter determine antibody function. Figure 14-6 shows a schematic illustration of immunoglobulin structure.
The NH2-terminal, Fab portion, of the immunoglobulin molecule is a complex three-dimensional structure with hypervariable regions that represent specific antigen-binding sites. Most antibody effector function is mediated through the constant Fc (carboxy terminus) region. The formation of antigen-antibody complexes results in complement activation or immunoglobulin binding to Fc receptors that are found on monocytes, macrophages, neutrophils, and NK cells. When complement binds to the antigen-antibody complex, phagocytosis is enhanced in a process referred to as opsonization.
Antibody Isotypes
IgM has the largest immunoglobulin structure. It is the chief component of the initial response to infection or antigenic stimulus. The half-life of IgM is relatively short lived compared with that of other immunoglobulins because it stays around for only 5 to 6 days. Due to its large size, it is found predominantly in the intravascular space where it serves as a bacterial agglutinin and robust activator of the complement system.
The most abundant antibody is IgG, which comprises approximately 85% of serum immunoglobulins. IgG is found in the intravascular and extravascular tissue compartments; it is the only immunoglobulin to cross the placenta from mother to fetus. IgG is the major type of antibody that responds to bacteria and viruses. The key biologic feature of IgG is its ability to bind antigen to the Fab portion while concurrently binding the Fc receptor on the neutrophil, monocyte, or macrophage with its Fc element. Another key feature of IgG is its ability to aggregate and activate the complement system.
Antibodies of the IgA isotype play a central role in local mucosal immunity. IgA is found predominantly in saliva, tears, bile, respiratory, and enteric secretions. IgA is produced by plasma cells in lymphoid tissue located adjacent to epithelial surfaces. IgA is released as a dimer that acquires a secretory component as it moves through the epithelial cells to the mucosal surface in the form of secretory IgA. A primary function of secretory IgA is to serve as an antiseptic paint that coats pathogenic microbes and therefore prevents microbial attachment, colonization, and tissue invasion. Whereas IgA synthesis is demonstrable in the small intestine and lung by the twentysixth week of gestation, most IgA synthesis occurs after birth. However, IgA is not solely responsible for mucosal immunity; IgG, IgM, and IgE can also play a role in mucosal immunity.
IgD and IgE have relatively low serum levels when compared with the other major circulating immunoglobulins. IgD is coexpressed with IgM on the surface of B lymphocytes and appears to aid in antigen recognition and subsequent lymphocyte activation. In humans, IgE is normally present in low concentrations. It is an important component of the acute allergic response. Following antigen binding, the Fc portion of the IgE molecule binds to mast cells and basophils, triggering histamine and serine proteinase release, as well as prostaglandin and cytokine (TNF-α, IL-1, IL-3, and IL-4) synthesis. IgE plays an important role in the host response to certain parasites.
Complement
Recognition of foreign antigen by antibody is normally the first step in the process of eliminating a pathogen. Whereas the mature, immunocompetent individual has an extensive repertoire of antibodies, the naive or immature host requires a unique mechanism to recognize and remove newly presented novel antigen. The phylogenetically primitive complement system serves this essential function. This system is composed of about 25 plasma proteins,
several membrane-bound glycoproteins, and their regulatory enzymes. It is presented in detail in Chapter 12 and in several published reviews (22,23,24). Figure 14-7 summarizes the complement system and discusses its physiologic role. The various protein products that result from complement activation interact to opsonize, or in some cases, directly kill pathogens. In addition, certain complement products modulate important aspects of the immune system, such as local blood flow and leukocyte trafficking. Complement activation is initiated by the classic pathway, through antigen-antibody complex formation, or by the alternative pathway after exposure to a variety of noxious stimuli, such as endotoxin, chemical toxins, or mechanical tissue injury. This latter pathway is of particular interest to surgeons because acute pancreatitis, burns, and other forms of trauma, for instance, are known to activate this pathway. Both pathways are illustrated in Fig. 14-7.
several membrane-bound glycoproteins, and their regulatory enzymes. It is presented in detail in Chapter 12 and in several published reviews (22,23,24). Figure 14-7 summarizes the complement system and discusses its physiologic role. The various protein products that result from complement activation interact to opsonize, or in some cases, directly kill pathogens. In addition, certain complement products modulate important aspects of the immune system, such as local blood flow and leukocyte trafficking. Complement activation is initiated by the classic pathway, through antigen-antibody complex formation, or by the alternative pathway after exposure to a variety of noxious stimuli, such as endotoxin, chemical toxins, or mechanical tissue injury. This latter pathway is of particular interest to surgeons because acute pancreatitis, burns, and other forms of trauma, for instance, are known to activate this pathway. Both pathways are illustrated in Fig. 14-7.
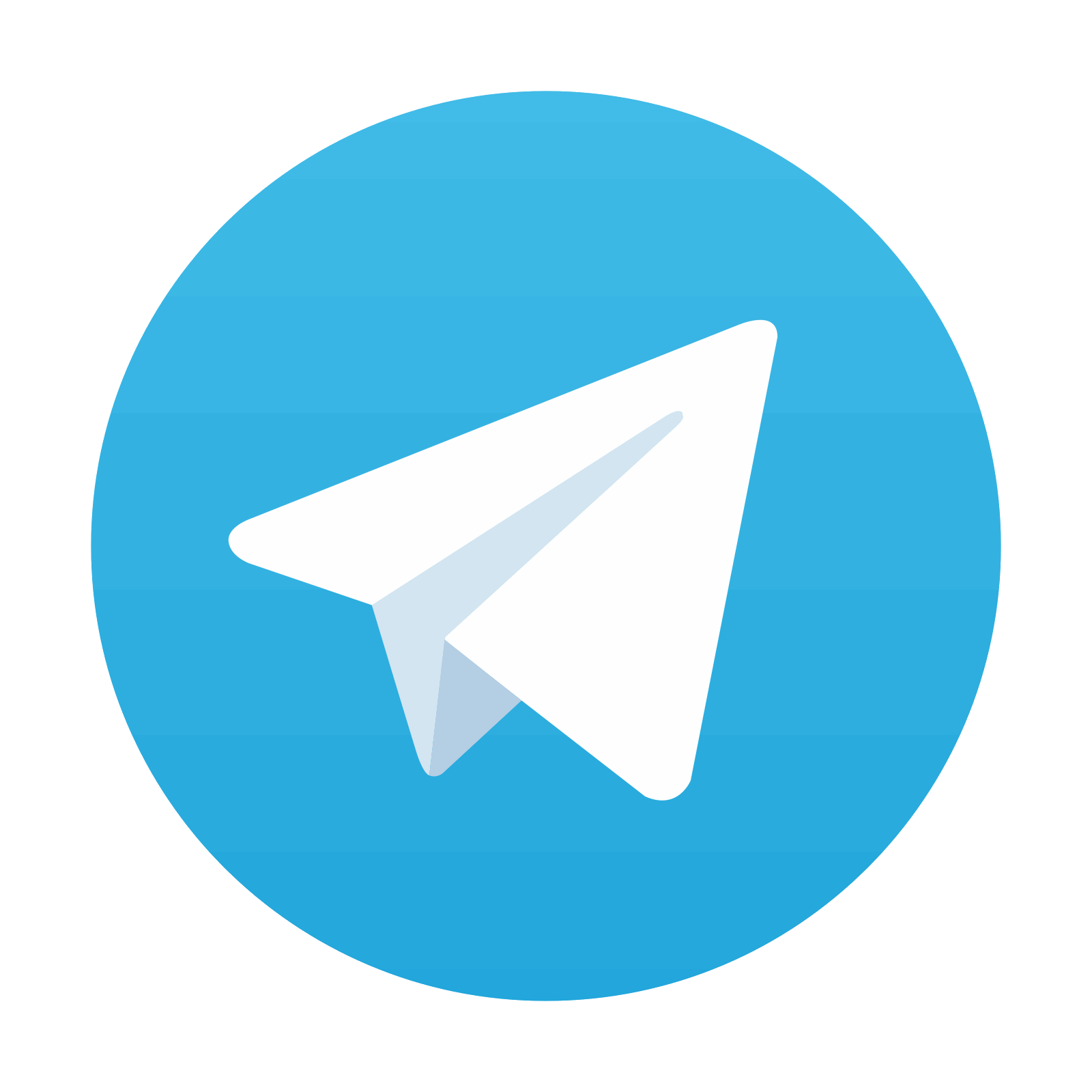
Stay updated, free articles. Join our Telegram channel

Full access? Get Clinical Tree
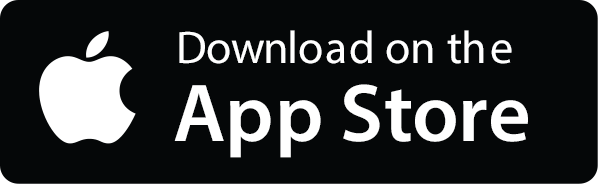
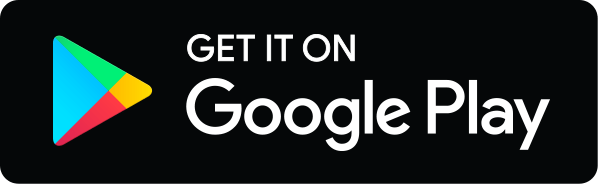
