Heat Stress
Michael Apkon
Hyperthermia is a state in which body core temperature rises as a result of heat generation and absorption exceeding heat loss. Given that body temperature reflects the balance between heat gain and heat loss, hyperthermia is found most frequently under conditions such as exercise in which heat production is increased or in subjects such as infants or patients with dehydration who lack the ability to increase their heat loss in response to environmental heat. Thus individuals who engage in athletic activities and infants are at particular risk for heat-related illness. Heat stress has been recognized as a cause of illness for more than 2000 years. It is responsible for increases in population death rates during hot times of the year in areas exposed to particularly high environmental temperatures,1 and increases morbidity related to other diseases. Heatstroke, the most serious form of heat-related injury, is the second most common cause of athletics-related death after head injury.
PHYSIOLOGY OF TEMPERATURE REGULATION
It is important to differentiate fever, where the body temperature set point is elevated as a result of the influence of pyrogenic cytokines (see Chapters 198 and 227), from hyperthermia, where the temperature is elevated above normal because the heat-dissipating homeostatic mechanisms activated at temperatures above the set point are overwhelmed. In febrile patients, homeostatic mechanisms including vasoconstriction and shivering are activated to raise body temperature by increasing heat production and decreasing heat dissipation until a new set point is reached whereas in hyperthermic patients, homeostatic mechanisms attempt to lower body temperature.
Metabolic activity leads to heat production. If no heat were lost to the environment, resting heat production would raise body temperature by approximately 1°C/hr. This increase would be even greater during exercise, when heat production may increase as much as 4-fold. Heat may also be absorbed from the environment by radiation from the sun or the ground, or by convection when the air temperature exceeds body temperature.
Heat production and absorption are balanced by heat loss via four mechanisms. Conduction carries heat between a body and a contacting surface along a temperature gradient. This is the primary mechanism of cooling during ice or water immersion. Convection transfers heat from the body surface to or from a gas or fluid circulating around the body. With conduction, heat transfer stops when the contacting surface temperature reaches body temperature, whereas with convection, circulation of fresh gas or fluid around the body preserves the temperature gradient between the body and the circulating gas or fluid thus increasing the efficiency of heat transfer. Radiation transfers heat from a warmer to a colder body via electromagnetic waves. Evaporation removes heat by promoting a phase transition from liquid to a gas. Of these four mechanisms, radiation is the principal mechanism of heat elimination in temperate environments. Heat loss by convection and radiation are increased by cutaneous vasodilation, a neurally mediated response to hyperthermia. As either heat production or environmental temperature increases, evaporation of sweat becomes the principal mechanism for heat elimination. It is important to recognize that sweating is the only mechanism for heat loss at environmental temperatures above body temperature because for heat to be lost by conduction, convection, or irradiation, body temperature must be warmer than the gas, fluid, or objects to which heat is dissipated. In fact, conduction, convection, and irradiation lead to heat gain when environmental temperatures exceed body temperature. Under these conditions, the adaptation to heat stress relies on both a greater rate of sweating at a lower degree of exercise and a lower electrolyte content of sweat. Sweating and subsequent evaporation are very effective mechanisms of temperature control, provided that the environment’s humidity is low enough to permit evaporation. This does not occur when the air becomes saturated with water vapor at humidity levels of 90% to 95% and is reduced at humidity levels greater than 75%. In addition, sweating can cause a substantial water and electrolyte loss occurring at the rate of 1 L/hr/m2 of body surface area. This may contribute to the clinical manifestations found in a child exposed to excessive heat.
CAUSES OF HYPERTHERMIA
Under most circumstances, hyperthermia in children is the result of decreased heat elimination, either because the subject has a limited ability to vasodilate (dehydration, shock, autonomic dysfunction, medications that impair thermal control) or sweat (dehydration, ectodermic dysplasia, medications that inhibit sweating), or because the normal mechanisms of heat dissipation are precluded by the circumstances of the environment (high ambient temperature, lack of convective flow, high humidity). Because in the presence of shock or dehydration, vasoconstriction (designed to maintain blood pressure) usually predominates over thermoregulation, patients may present with an increased core temperature and cool extremities.2
Occasionally, however, hyperthermia can occur if the amount of heat generated by the body overwhelms the normal mechanisms of heat dissipation (strenuous exercise, thyrotoxicosis). Hyperthermia resulting from increased heat production is exacerbated when ambient conditions make heat dissipation less efficient. Hyperthermia can also be caused by certain medications. Drug-induced hyperthermia can be found in malignant hyperthermia associated with anesthetics, neuroleptic malignant syndrome, anticholinergic syndrome, serotonin syndrome associated with serotonin reuptake blockers, and sympathomimetic poisoning. Drug-induced hyperthermia can result from interference with heat-loss triggering mechanisms in the anterior hypothalamus or through increased heat generation as a result of muscle rigidity produced by direct muscle stimulation or through extrapyramidal pathway stimulation.
CLASSIFICATION OF HEAT-RELATED ILLNESS
Heat-related illnesses are a spectrum of disorders varying in the severity of their manifestations from heat cramps, heat syncope, and heat exhaustion, to the life-threatening condition usually described as heatstroke. Heat cramps are thought to be caused by electrolyte disturbances resulting from thirst-driven replacement of large sweat losses with hypotonic fluid. They affect large muscle groups and are more common after exercise. Heat syncope occurs as a result of decreased cerebral blood flow secondary to vasodilation-induced peripheral blood pooling, dehydration, and gravitational redistribution of blood in upright individuals.
Heat exhaustion and heatstroke refer to the more severe forms of heat-related illness and represent a continuum with poorly defined boundaries. With both conditions, illness is in part a consequence of homeostatic attempts at temperature regulation. With heatstroke, those homeostatic mechanisms become overwhelmed and temperature rises above normal. Heat exhaustion, the most common form of heat-related illness, is characterized by intravascular volume depletion under conditions of heat stress and encompasses a poorly defined array of nonspecific symptoms and signs that include weakness, malaise, headache, dizziness, orthostatic or nonorthostatic hypotension, dehydration, tachycardia, nausea, and vomiting. Typically, mental function is preserved, sweating persists and may be profuse, and body temperature is normal or mildly elevated.
In heatstroke, body temperature is elevated (rectal temperature > 40°C), there are deficits in mental function (ranging from disorientation to coma), and there is a history of heat exposure. Classically, there is cessation of sweating, although some individuals may continue to sweat, even profusely. Heatstroke may be characterized as exertional or nonexertional. Individuals succumbing to exertional heatstroke tend to be young, healthy individuals engaged in vigorous activity. The impact of heat stress on these individuals may be exacerbated by limited access to water. Even when there is free access to water, heat-stressed individuals frequently voluntarily replace only a fraction (∼ 2/3) of the fluids that they lose. Nonexertional heatstroke develops more slowly in individuals without the capacity to regulate their environment or to replace ongoing losses of fluid. Infants and individuals with chronic disease are, therefore, at highest risk.
EFFECTS OF ELEVATED BODY TEMPERATURE
Hyperthermia has negative effects on both cellular and organ functions. At a cellular level, it raises metabolic activity, increasing heat production as well as the demand for oxygen delivery. At an organ or system level, the characteristic response of the organism to heat stress is cutaneous vasodilation. This results in a shift in the blood volume from the central venous circulation to the skin and a decrease in systemic vascular resistance. These effects are partially compensated by splanchnic vasoconstriction. Cardiac output initially rises to compensate for both the increased metabolic activity and the vasodilation. The rise in cardiac output results from an increase in heart rate and myocardial contractility as well as from the reduction in afterload. Redistribution of blood volume from the central veins and reduction in intravascular volume by dehydration limit this rise in cardiac output. When intravascular volume depletion and redistribution become severe, cardiac output will fall. When temperature elevation is more severe, cardiac output may be reduced further because of myocardial injury and conduction disturbances. Cardiovascular collapse may ensue when the circulatory disturbances become sufficiently severe that homeostatic processes defending against hypotension (ie, peripheral vasoconstriction) compete with those defending against hyperthermia (ie, cutaneous vasodilation). Even before cardiovascular collapse occurs, when cardiac output and oxygen delivery are insufficient to meet the demands of an accelerated metabolic rate, cell function may be disrupted by depletion of their energy stores. Some authors have suggested that there is a critical maximal temperature above which cellular injury is likely to occur. However, it is perhaps more accurate to consider injury to represent the integrated effect of the extent and duration of hyperthermia.
All organ systems may be affected by severe hyperthermia. Alterations in mental function are universal in heatstroke and range from delirium, convulsions, and opisthotonus to coma. Cerebellar injury leading to ataxia is common and its manifestation may be delayed and progressive. Despite cooling the liver may be particularly sensitive to the effects of hyperthermia because of its high metabolic rate, which leads to a normal temperature above body-core temperature. A consumptive coagulopathy and edema are both common, indicating activation of systemic inflammation of magnitude sufficient to cause an endothelial injury. Muscle injury may be manifest as rhabdomyolysis. The myoglobinuria that results from muscle injury may precipitate acute renal failure and acute tubular necrosis. Diminished cardiac output, hypotension, and direct heat injury may also cause or contribute to renal failure. Impaired respiration usually results from pulmonary edema that may develop as a result of myocardial failure (ie, cardiogenic pulmonary edema) or as a result of increased pulmonary capillary permeability. Hyperventilation is common, leading to development of a respiratory alkalosis, which may be manifest as tetany from the reduction of ionized calcium concentrations that accompanies alkalosis.
Although many of the consequences of hyperthermia may be ascribed to direct effects of temperature elevation, it is likely that some effects are secondary to the generation of inflammatory cytokines, particularly interleukin-1 (IL-1). In animals, immunization against bacterial endotoxin before heat stress markedly reduces heat-induced elevations in interleukin levels and mortality. Moreover, treatment of animal subjects with an IL-1 receptor antagonist at the time of heat exposure reduces the cardiovascular dysfunction caused by hyperthermia. It is not known to what extent cytokines play a role in human heat-related disease.
TREATMENT OF HYPERTHERMIA
Therapy for heat-related illness is aimed at supporting cardiorespiratory function, repleting intravascular volume, and reducing temperature. For milder forms of heat injury, reducing the level of physical activity, transport to a cooler environment, and oral rehydration are typically sufficient. When heat cramps are present, salt as well as water may be replaced using a 0.1% to 0.2% salt solution (∼ 1/4 tsp table salt per 8 oz water).
Heatstroke is a medical emergency and temperature reduction is a central priority. Cooling is the mainstay of first aid and can be initiated by removing clothing and drenching with cool water. Cooling should continue during and following transport to a hospital but should be terminated when the core temperature is less than 38.5°C to 39°C to prevent hypothermia. A number of methods3 are effective at reducing body temperature (Fig. 121-1). Water immersion produces the greatest rate of temperature decrease (∼ 0.15−0.2°C/min) using noninvasive means. Although ice-water immersion can theoretically accelerate heat loss compared to warmer water, some authors have suggested that this effect is limited by intense shivering that increases heat production and by cutaneous vasoconstriction. The literature shows mixed results in demonstrating that colder water is more effective. Evaporative cooling may be nearly as effective as water immersion if optimized with atomized spray of lukewarm water (to minimize cutaneous vasoconstriction) combined with fanning to increase convection. More invasive methods of cooling directly seek to cool the body’s core and may be accomplished by ice-water gastric lavage, cold saline peritoneal, or pleural lavage or by cardiopulmo-nary bypass. Antipyretics are not indicated in the management of heatstroke. Acetaminophen may potentiate hepatic injury and other nonsteroidal anti-inflammatory drugs may potentiate renal injury and coagulopathy.
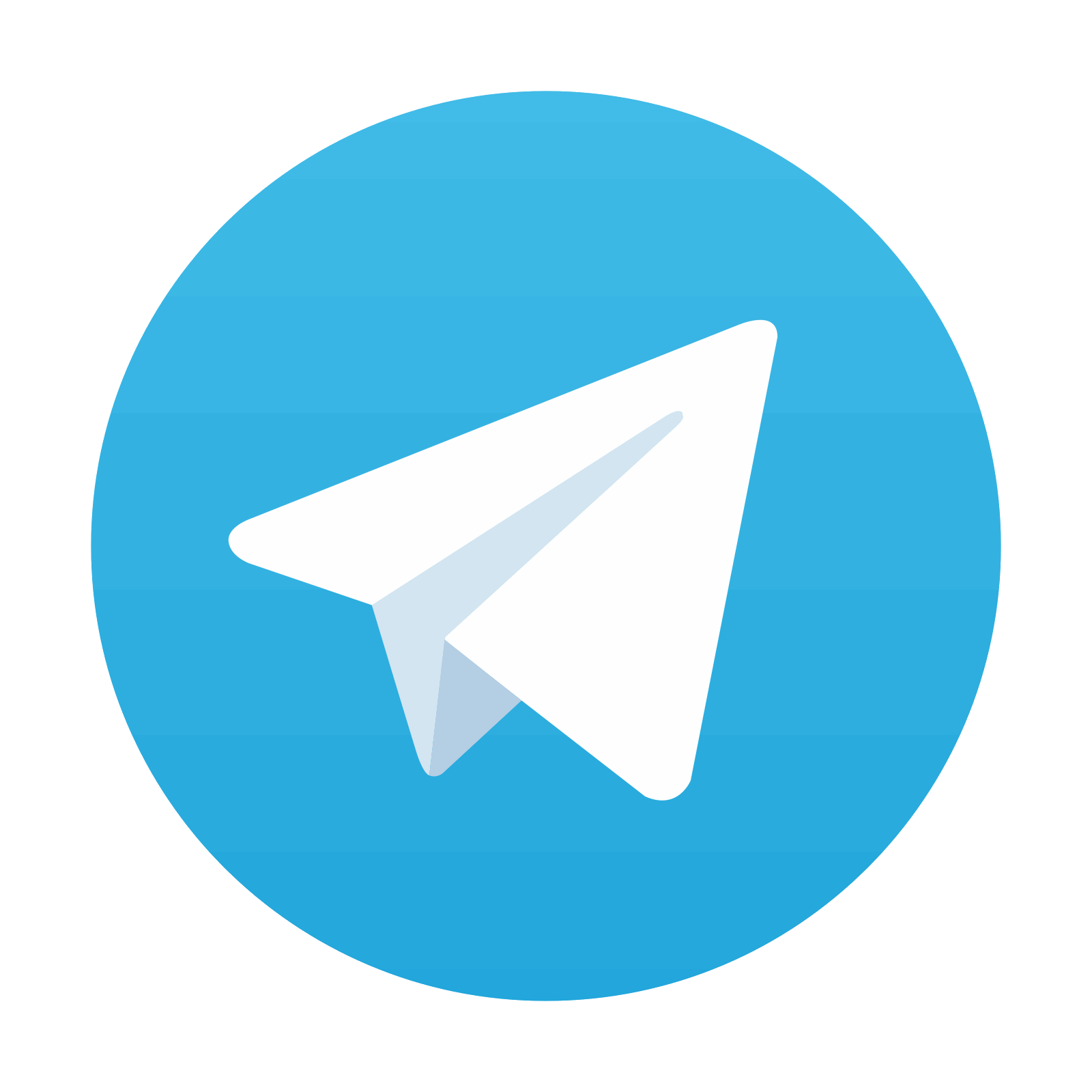
Stay updated, free articles. Join our Telegram channel

Full access? Get Clinical Tree
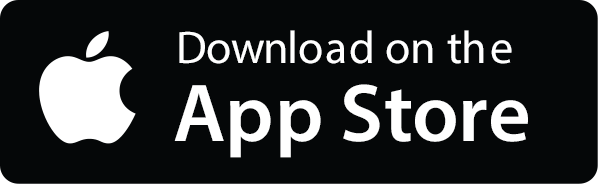
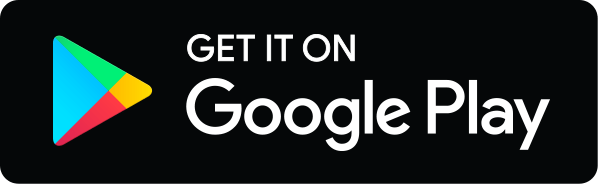