Head Injury and Intracranial Pressure Management
Cheryl A. Muszynski
Bruce A. Kaufman
Medical College of Wisconsin, Pediatric Neurosurgery, Children’s Hospital of Wisconsin, Milwaukee, Wisconsin 53226.
Medical College of Wisconsin, Pediatric Neurosurgery, Children’s Hospital of Wisconsin, Milwaukee, Wisconsin 53226.
EPIDEMIOLOGY
Head trauma is a significant cause of death and disability among children in the United States. The annual incidence of head injury in the United States has been estimated at 200 per 100,000 pediatric population (1). Nearly 10% of these patients will die from their head injury, and up to three-fourths of all pediatric trauma deaths are due to cranial injuries.
The morbidity in those who survive varies greatly. Minor injuries can be associated with reversible deficits, whereas more serious injuries often result in lifelong disability. The magnitude of morbidity in children with head injuries clearly overshadows the mortality and represents the greatest expense to the patients’ families and society.
The mechanisms of injury in children are quite distinct from those seen in adults, and often relate to a child’s age and developmental stage (2). Infants suffer disproportionately from falls, such as accidentally dropping from a table or a person’s arms. As children become more mobile, recreational activities and vehicle-related accidents predominate. Older children are more often victims in higher-speed accidents involving bicycles or motor vehicles. Overall, motor vehicles are the leading cause of accidental head injuries in children resulting in death.
Criminal assault is the most common cause of head injury in children younger than 2 years old (3). Abuse and neglect accounts for the higher mortality rate documented in infants compared with older children. Because a history of a fall is usually given, abuse is likely to be an underreported cause of head injury.
The mortality rate in children with head injuries is significantly less than that in adults. This mortality rate decreases just after infancy (when the incidence of abuse and neglect declines) and then rises slowly until early adolescence (4). The rate then rises very quickly due to the larger number of patients involved in high-speed motor vehicle accidents.
Better survival rates from head trauma in children than in adults do not necessarily mean better outcomes. Children have a neuronal plasticity related to the degree of myelination and the establishment of neuronal interconnections. This plasticity may allow a given focal injury to produce a less severe deficit than the same focal injury in a mature brain. However, this same lack of maturity can also make the infant more susceptible to a diffuse injury, resulting in far greater cognitive deficits than in adults (5).
PATHOPHYSIOLOGY
Normal Anatomy/Physiology
A number of anatomic differences between children and adults can affect their responses to head trauma. The head of a young infant or child is a large mass relative to the whole body (15% of an infant’s mass versus 3% of an adult’s). The accelerations and decelerations encountered in pediatric trauma, therefore, result in a greater momentum of force applied to the brain. The neck musculature is underdeveloped and poorly controlled, and offers no significant diminution of these forces.
The skull of the infant is thin, soft, and pliable, becoming progressively thicker and more brittle in the first few years of life. The fontanelles and sutures begin to close in the first year and are effectively closed within 3 years. These changes will affect the types of injury received from blunt trauma.
The character of the intracranial contents of children also varies significantly from that in adults. The cerebrospinal fluid (CSF) spaces, both intraventricular and subarachnoid, are present in children but the relative
volumes are much smaller than in adults. The infant’s brain tissue has greater water content. Myelination occurs during months 6 through 24, and most of the synaptic and dendritic arborization also occurs in the first few years. These factors directly affect the consistency of the brain tissue—the infant’s brain is soft and easily disrupted.
volumes are much smaller than in adults. The infant’s brain tissue has greater water content. Myelination occurs during months 6 through 24, and most of the synaptic and dendritic arborization also occurs in the first few years. These factors directly affect the consistency of the brain tissue—the infant’s brain is soft and easily disrupted.
Intracranial pressure (ICP) is derived from the inherent pressure of the components within the cranial and spinal compartments—the brain, blood vessels, and CSF spaces. Any increase in volume of one component decreases the volume of another and raises the ICP, if compensatory changes are not possible. Brain or intracranial blood volume changes are initially modulated by displacement of CSF from the intracranial space to the intraspinal space. The ICP shows normal variations with both respiration and cardiac pulsation. Marked but transient increases in ICP with activity are normal, particularly with straining and coughing, which increase the venous blood pressure and distend the veins in the spinal and intracranial spaces.
Autoregulation closely regulates cerebral blood flow through a tight coupling of cerebral metabolism with regional blood flow (Fig. 22-1). Mean arterial pressure (MAP) has little effect on blood flow over the normal ranges of pressure. Arterial hypoxia does not usually affect cerebral blood flow until the PaO2 drops below 50 mm Hg. However, a 1 mm Hg change in the PaCO2 can cause a rapid drop in the blood flow of 1.5 to 2 mL per 100 g of brain, which can be regional.
The brain depends on constant blood flow so oxygen and glucose can maintain the brain’s metabolic state. The brain has no significant anaerobic metabolic capability or energy reserves. If blood flow is insufficient, ischemia and disruption of function results. If the ischemia is severe or prolonged, irreversible injury (infarction) results. Cerebral perfusion pressure (CPP) is the difference between MAP and ICP. The accepted lower limit of normal CPP in adults is 40 to 50 mm Hg. Infants have a lower normal systemic blood pressure, and thus a lower normal CPP. Although the minimal normal CPP for infants and children is not well defined, more recently published guidelines recommend that, for children with traumatic brain injury (TBI), the CPP should be maintained above 40 mm Hg (6,7).
Pathophysiology
As an aid to diagnosis and treatment, it is useful to classify head injuries as primary or secondary. Primary injuries are those inflicted immediately by the trauma, such as skull fractures, subarachnoid hemorrhage, cerebral contusions or lacerations, intracranial or intracerebral hematomas, and axonal injuries. Ischemia, hypoxia, hypotension, infection, hydrocephalus, seizures, or increased ICP subsequent to the trauma can cause secondary injuries to brain tissue. Secondary injuries are potentially preventable and thus are the focus of most treatment plans.
Compared with adults, children have relatively few subdural or epidural hematomas. Children with an epidural hematoma usually present in better neurologic condition than adults and rarely present in coma after a lucid interval. Children are more likely to have low-pressure venous bleeding from an overlying skull fracture than to have dural arterial lacerations causing epidural hematomas. However, young children may be at greater risk for dural lacerations and shear and parenchymal injury with subsequent edema because of a greater transmission of force through the thin bone of the skull and the open cranial sutures. Unique to the young infant whose intracranial volume is relatively large compared with body size, a large intracranial hemorrhage can actually drop the hematocrit or hemoglobin.
Diffuse axonal injury is a primary injury that results from shear stresses on the brain imparted by acceleration-deceleration forces, particularly when combined with angular or rotational motion. The typical sites of this injury include the internal capsule, corpus callosum, cerebellar peduncle, and brain stem. Even minimal shearing injury in these regions can result in severe neurologic deficits. Shearing is more common in infants and small children than in older children because of the greater rotational forces from the larger momentum of force.
Microscopically, the spectrum of injury can vary from a functional disturbance of the neuron that is quickly reversible to a complete disruption of the axons that is an irreversible injury with permanent loss of function (8).
Secondary injuries are frequently due to increased ICP. Increased ICP results from the expansion of one or several components of the intracranial space: the cerebral blood volume (both venous and arterial), brain tissue volume, hematomas (intracerebral, subdural, epidural), and CSF spaces. Increased ICP can result in direct compression and injury of brain structures, such as herniation under the falx cerebri or through the tentorium cerebelli (Fig. 22-2). Indirect injury from increased ICP can occur through decreased cerebral blood flow (as a result of decreased CPP) with resulting focal or diffuse secondary ischemic injury.
The normal CSF spaces can be displaced in response to the expansion of other components, acting as a buffer for increasing ICP. CSF spaces that are trapped or obstructed, as may happen with subarachnoid or intraventricular hemorrhage, act as additional masses and increase ICP. Despite open fontanelles and sutures, children tend to have less CSF buffering capacity, probably related to a smaller spinal CSF volume. This puts them at risk for more sudden decompensation from smaller changes in ICP (9).
ICP can also rise as a result of interstitial edema of the brain tissue. A focal area of edema can be particularly deleterious if it impinges directly on the brainstem or obstructs the CSF flow. If an area of brain loses autoregulation, vasodilation unrelated to hypercarbia or metabolic demands (hyperemia) can cause a significant increase in tissue volume and thus in ICP (9). Some children with relatively minor head injuries can suffer from global hyperemia with rapid deterioration and death (10).
EVALUATION AND DIAGNOSIS
The clinical history in the child with a head injury should include determination of the mechanism of injury and of whether any changes in the patient’s clinical examination have occurred from the time of injury. A high-speed, high-force mechanism of injury raises a greater level of concern for a significant injury regardless of the clinical appearance. A decline in the child’s level of function, even if subtle, may be the only clue to an underlying significant injury.
During the physical examination, emphasis should be placed on the trend of serial examinations and the presence of any focal neurologic findings, including lethargy and irritability. The patient with a deteriorating neurologic examination requires more urgent and aggressive treatment than the patient who is initially comatose and then improves. Systemic hypotension or hypoxia should be promptly corrected because the brain is dependent on blood flow for normal metabolism. It is axiomatic that an intracranial injury is not the cause of hypotension. If systemic hypotension is present, a cause other than the head injury should be identified. One of the only possible exceptions to this is a young child who may lose sufficient blood from a scalp laceration to cause hypotension.
The typical signs of increased ICP are frequently absent or incomplete in children, even with a significantly elevated ICP. Pupillary changes can develop long after significant ICP changes have occurred because third-nerve palsies are the result of nerve compression by displaced cerebral tissue. Papilledema requires days to develop and does not present in acute trauma. The Cushing response to increased ICP (bradycardia with systemic hypertension) is not as predictable in children and may be a preterminal event.
The Glasgow coma scale (GCS) is the standardized tool for assessing and reporting the neurologic status of a trauma victim. It is based on the patient’s best response in
three categories: motor activity, verbal response, and eye opening. The GCS score has been validated as an easily applied measurement that accurately portrays the severity of the injury and allows for a temporal comparison of the patient’s condition. It has been modified for pediatric patients, allowing its use with the nonverbal infant or child (11) (Table 22-1). The usual definitions of severity used in the literature are defined in the GCS as follows: severe is a GCS score of 8 or lower; moderate, 9 to 12; and mild, 13 to 15. Based on evaluations of outcome, it is clear that all mild head injuries, as defined by GCS score of 13 or higher, are not the same. Patients with a GCS of 13 or 14 may have significant intracranial injury or morbidity after recovery.
three categories: motor activity, verbal response, and eye opening. The GCS score has been validated as an easily applied measurement that accurately portrays the severity of the injury and allows for a temporal comparison of the patient’s condition. It has been modified for pediatric patients, allowing its use with the nonverbal infant or child (11) (Table 22-1). The usual definitions of severity used in the literature are defined in the GCS as follows: severe is a GCS score of 8 or lower; moderate, 9 to 12; and mild, 13 to 15. Based on evaluations of outcome, it is clear that all mild head injuries, as defined by GCS score of 13 or higher, are not the same. Patients with a GCS of 13 or 14 may have significant intracranial injury or morbidity after recovery.
Radiologic evaluation has routinely used plain skull radiographs. Although inexpensive, they only define skull fractures. Only depressed or open fractures are clinically important in themselves, and they are usually well defined by clinical examination combined with computed tomography (CT). The presence of a skull fractures is associated with an increased risk of intracranial injury (12,13). However, the absence of a skull fracture does not reliably exclude a significant intracranial injury, such as an epidural or subdural hematoma (14,15).
CT is the procedure of choice in evaluating head trauma (16). CT allows for the prompt identification and treatment of those injuries that are potentially reversible, such as the intracranial hemorrhagic masses and the sequelae of depressed fractures. The newest scanners are fast, with slice acquisition times of several seconds, which negate most patient motion. Cerebral contusions and shifting of the brain structures can be seen directly, and increased ICP can be inferred from obliteration of normal CSF spaces. When the images are adjusted to evaluate bone density, depressed fractures and most clinically important linear skull fractures are identified.
Radiographic evaluation using CT is clearly indicated for patients who present with GCS scores of 13 or below; immediate scanning is necessary for severely injured patients (GCS up to 8). Plain radiographs have no acute diagnostic role in these patients unless indicated for surgical planning. Debate is ongoing regarding the proper radiographic evaluation of minor head injury (i.e., GCS score of 15). Significant abnormalities have been detected by CT in 10% of patients with GCS of 15, often with no other findings beside a history of loss of consciousness or amnesia (17,18,19).
CT scanning is recommended for any child with a head injury who has any neurologic deficit or a GCS score of 14 or lower. Regardless of the GCS, CT scanning is warranted when factors such as a high-force mechanism of injury, changes in the level of consciousness, or planned interventions with a loss of the clinical examination are present. The threshold for scanning is also reduced for children younger than 2 years of age.
TABLE 22-1 Modified Glasgow Coma Scorea for Children | ||||||||||||||||||||||||||||||||||||||||||||||||||||||||||||||||||||||||||||||||||||||||
---|---|---|---|---|---|---|---|---|---|---|---|---|---|---|---|---|---|---|---|---|---|---|---|---|---|---|---|---|---|---|---|---|---|---|---|---|---|---|---|---|---|---|---|---|---|---|---|---|---|---|---|---|---|---|---|---|---|---|---|---|---|---|---|---|---|---|---|---|---|---|---|---|---|---|---|---|---|---|---|---|---|---|---|---|---|---|---|---|
|
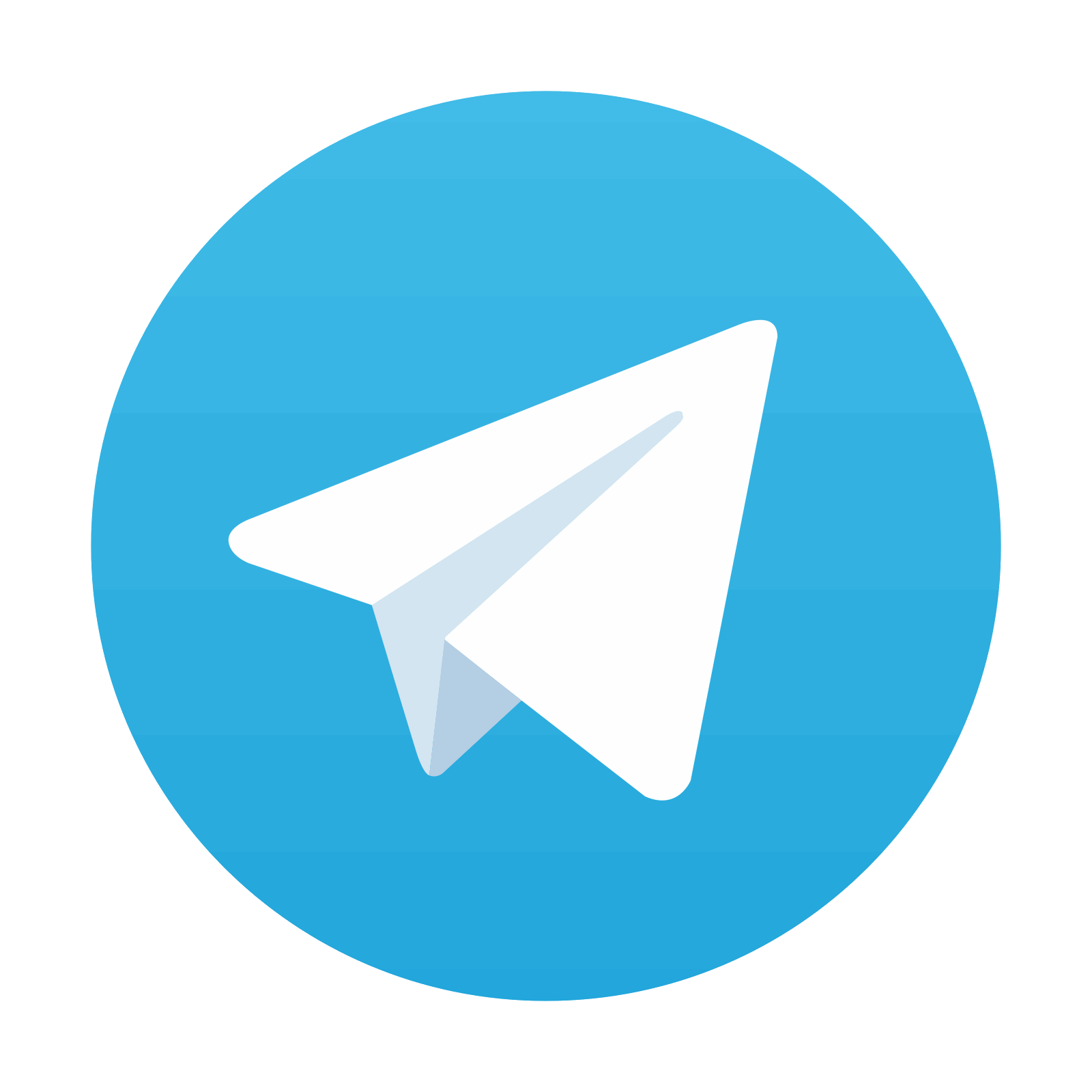
Stay updated, free articles. Join our Telegram channel

Full access? Get Clinical Tree
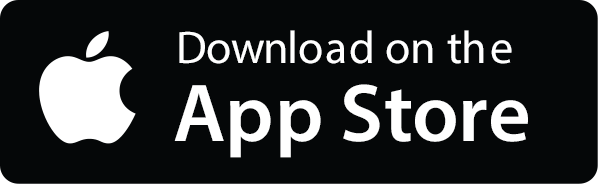
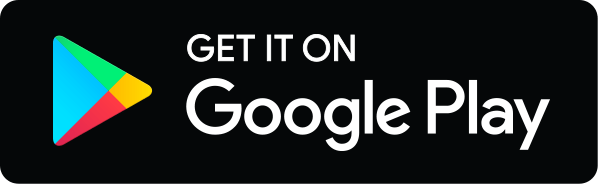