!DOCTYPE html PUBLIC “-//W3C//DTD XHTML 1.1//EN” “http://www.w3.org/TR/xhtml11/DTD/xhtml11.dtd”>
Moira M. McCarthy Elizabeth Cody Jo A. Hannafin
|
CHAPTER |
Anterior cruciate ligament (ACL) injury is a common problem in female adolescent athletes playing cutting, pivoting, and landing sports with a greater incidence than male adolescent athletes.1 The rise in ACL injuries in adolescents is multifactorial and may reflect widespread participation in sports at younger ages and year-round competition. The gender disparity in ACL injury incidence deserves attention secondary to potential consequences, including loss of participation in athletics, potential loss of scholarship funding and educational opportunities, and chondral and meniscal damage ultimately leading to osteoarthritis.2,3 The intrinsic gender differences that have been proposed as risk factors are multifactorial and include anatomic, hormonal, neuromuscular, and biomechanical differences. Identification of the differences between males and females especially during the adolescent growth period is important to provide insight into design and implementation of injury prevention programs to decrease the risk of ACL injury.
GENDER DIFFERENCES IN BONE MATURITY
Females reach maturity at a younger age than males, which may affect the surgeon’s decision regarding technique of ACL reconstruction. More than 25% of North American males have open physes at age 16 years, whereas 75% of females have stopped growing by age 14 years and nearly all have closed physes by age 16 years.4
There are also significant differences in the characteristics of female bone compared to male bone, which begin to appear during puberty. Several imaging modalities have been used to study the changes that occur in female and male bone from childhood to adulthood. Macdonald et al.5 used peripheral quantitative computed tomography (pQCT) to assess total bone cross-sectional area and cortical area in the tibial midshaft of 128 females and males during various stages of puberty. They found no gender differences in these variables in prepubertal children. However, during puberty, males had significantly greater increases in both total cross-sectional area and cortical area as compared to females.
Hogler et al.6 found similar results: They performed magnetic resonance imaging (MRI) and dual-energy x-ray absorptiometry (DEXA) studies on the femurs of 145 males and females ranging in age from 6 to 25 years. They found that in prepubertal children, there was no gender difference in bone mineral content, cortical bone mineral density, cross-sectional bone geometry, and muscle area in the proximal third of the femur. After puberty, males had a greater total and cortical bone area, bone diameter, and muscle area than their female counterparts, even when adjusted for femur length and weight. However, relative to muscle area, postpubertal females had greater total and cortical bone area than men.
Neu et al.7 assessed volumetric bone density in addition to total and cortical cross-sectional bone area. They used pQCT to analyze the radial diaphysis of 469 subjects ranging in age from 6 to 40 years. From childhood to adulthood, females increased their total cross-sectional area by 50%, compared to 116% in males, with similar increases in cortical area. Bone mineral content increased by 111% in females and 140% in males; however, volumetric bone mineral content increased by 48% in females, more than double the 23% increase seen in males. Kontulainen et al.8 also used pQCT of the tibial mid-diaphysis to show that during puberty, females increase the density of their cortical bone to a greater extent than males. By adulthood, females have a smaller volume of bone than males, but it is more dense bone. Some authors have hypothesized that this additional bone mineral content in women functions as a reservoir of calcium and other minerals needed for reproduction.9,10
The amount and strength of bone that women have also appears to be modifiable by physical activity. Ward et al.11 used pQCT and DEXA studies to compare 86 prepubertal gymnasts with age-matched controls. The gymnasts had 5% greater cortical area and 6% greater cortical thickness than controls, corresponding to about a 5% greater bone strength. In the distal radius and tibia, sites of primarily trabecular bone, volumetric bone density was up to 17% greater in the gymnasts than in controls.11
Kontulainen et al.12 studied 64 female tennis and squash players compared to age-matched controls using pQCT and DEXA. Their findings were similar to those of Ward et al.11 in that the athletes had greater cortical bone area, higher bone mineral content, and higher volumetric trabecular bone density. Interestingly, they also compared the athletes who began playing their sport before or after menarche. Early starters, who began the sport before menarche, had higher bone mineral content, total cross-sectional area, cortical area, cortical thickness, and torsional bone strength index than the older starters. Their findings suggest that physical activity has an even greater effect on bone mineral content and bone architecture if started prior to puberty.12
Females who engage in more physical activity before puberty may increase their bone mineral content and bone size to an extent greater than that seen in males. Hasselstrom et al.13 evaluated 135 males and 108 females aged 6 to 8 years who participated in a program in which the time spent in physical education classes was doubled to 3 hours per week. DEXA evaluation after 3 years showed that the females had a 12.5% increase in distal forearm bone mineral content and a 13.2% increase in distal forearm scanned area compared to controls who participated in the standard curriculum. Among the males, however, there was no difference between the intervention group and the control group.
It is important to note for the young female athlete that bone age may be affected by sport participation. The most common example may be gymnastics. Markou et al.14 studied 169 female elite artistic gymnasts. In this group, bone age was delayed by 2 years compared to chronologic age.
Overall, major changes occur in the architecture and composition of bone in both females and males during puberty. Compared to males, females reach adulthood with less bone volume and less cortical thickness but with higher density of bone. The bone variables are more likely to be modifiable by increased activity. The young female athlete sees primarily increases in thickness at cortical bone sites and in bone density at trabecular bone sites. With high intensity of exercise, however, bone age may be delayed. The surgeon should be aware of these factors when treating an adolescent female athlete with an ACL injury.
EPIDEMIOLOGY OF ANTERIOR CRUCIATE LIGAMENT INJURIES
ACL injuries are most common in patients in their late teens and early 20s, with soccer being the most common sport implicated.15 Approximately 70% of ACL injuries occur by a noncontact mechanism. The characteristic mechanism described is at foot strike when landing or decelerating, with a valgus stress on the knee, the tibia in internal rotation, and the knee in slight flexion.16
Females have been reported to have higher rates of ACL injury than males, with some estimating up to an eight times greater incidence of ACL tears in women than in men.16 Prodromos et al.17 conducted a meta-analysis of all studies which reported ACL tear incidence in an effort to clarify the roles of gender, sport, and knee injury reduction regimens. Most significantly, they found that the gender difference varies by sport. The female-to-male ACL tear incidence ratio was highest in basketball at 3.5, decreasing to 2.67 in soccer, 1.18 in lacrosse, and 1.0 in alpine skiing. From their data, the authors deduced that women playing high-level soccer or basketball have a 5% risk each year for ACL injury. Women playing handball, rugby, and wrestling also had increased risk relative to men, although due to the low number of exposures reported, these findings were less reliable.17
Age may also play a role in the gender difference in ACL incidence. In the meta-analysis, Prodromos17 noted that in basketball, the overall incidence of ACL tears was significantly higher in collegiate female athletes than in high school female athletes (0.29 tears per 1,000 exposures vs. 0.09). However, the female-to-male incidence ratio was higher in the high school athletes (4.5 vs. 3.63).17
These findings correlate with the observation that increased risk in females appears to arise at puberty.18 ACL injury in children younger than the age of 10 years is extremely rare; incidence increases throughout adolescence to a peak incidence at age 17 years. The incidence of ACL injury in the pediatric population has been increasing, possibly due to increased sports participation at increasingly younger ages.19 Caine et al.20 conducted a review of studies reporting incidence rates of sports injuries in children. Females younger than the age of 18 years who play basketball were found to have approximately doubled the risk of sustaining any knee injury and up to nine times the risk of sustaining an ACL injury.20
Parkkari et al.21 reported on ACL tear incidence among 46,000 Finnish adolescent athletes aged 14 to 18 years over a period of 9 years. In this population, the incidence of ACL injury was 60.9 per 100,000 person-years. The incidence in male subjects was more than three times the incidence in female subjects. However, the risk of ACL injury was greatest in females who participated in organized sports at least four times per week; this risk was double that seen by males.21
Dodwell et al.19 examined New York State database records over 20 years, from 1990 to 2009, to identify the rate of ACL reconstruction per 100,000 person-years in the population aged 3 to 20 years. In this population, the rate of ACL reconstruction increased from 17.6 in 1990 to 50.9 in 2009. The youngest patient to undergo ACL reconstruction was age 9 years. At the peak age of 17 years, the rate was 176.7. Males had a 15% higher rate of ACL reconstruction compared to females. Although the rate of ACL tears is almost certainly higher than the rate of ACL reconstruction, the data still highlights the fact that ACL injury in children and adolescents is likely on the rise.19
ACL injuries are frequently associated with other injuries, including injuries to the menisci, articular cartilage, medial collateral ligament (MCL), and posterior cruciate ligament (PCL). These injuries tend to vary by mechanism: Compared to ACL injuries sustained from soccer, skiing injuries are more often associated with PCL, MCL, and multiligamentous injuries, and basketball injuries are more frequently associated with meniscus and cartilage injuries.22
Reported rates of meniscal injury in patients with ACL tears range from about 40% to 60%.15,23 Isolated injury to the medial meniscus is most common, followed by injury to the lateral meniscus. Females have a lower rate of injury to the menisci, suggesting that a less forceful mechanism may be required to cause an ACL tear in females.23–25
REASONS FOR INCREASED FEMALE ANTERIOR CRUCIATE LIGAMENT INJURY RATE—RISK FACTOR ANALYSIS
Females are more likely than males to sustain an ACL injury. Differences between the anatomy and biomechanics of males and females exist only after puberty, which corresponds with the developmental time period when the gender differences in ACL injury rates occur.26–28
Anatomic, hormonal, neuromuscular, and biomechanical risk factors in female athletes have been investigated in numerous sports, including soccer, basketball, volleyball, and handball. Specific risk factors that are often discussed and widely studied are body mass index (BMI), generalized and specific knee joint laxity, Q angle and other anatomic considerations, intercondylar notch width, ACL size and strength, pelvic anatomy, posterior tibial slope, and foot pronation.29 In addition, neuromuscular and biomechanical gender differences exist, and, together, these form the basis for ACL injury prevention programs.30
Anatomic Gender-Based Risk Factors
Anatomic risk factors such as tibia length and thigh length and height have been minimally studied because the ability to modify these factors is low. Beynnon et al.31 suggested that an increased thigh length in female skiers was related to an increased injury rate. However, these static anthropometric differences have not correlated well with dynamic injury mechanisms.32
Females have a higher BMI than males. A prospective study of cadets at the U.S. Military Academy demonstrated that a higher BMI in females was associated with an increased injury risk.33 Uhorchak et al.33 reported that females with a BMI 1 standard deviation (SD) above the mean had a 3.2 to 3.5 times greater risk of ACL injury than those with normal or below normal BMI. Height and weight were also shown to be predictors of injury in adolescent females in a longitudinal study of adolescent soccer players.34 In prepubescent athletes, there was no gender difference in knee injury, but females aged older than 11 years had an increased risk of knee injury.34 In females older than age 8 years, higher BMI was also correlated with an increase in knee injury risk.34 The authors estimated that females began to have a higher risk of knee injury at age 12 years, which correlated with the timing of increased BMI in females related to pubertal development.34 Brown et al.35 noted in college-aged recreational athletes that an increase in BMI resulted in a more extended position of the lower extremity with a decreased knee flexion velocity on landing, which was postulated to lead to an increase in ACL injury. Knapik et al.36 prospectively evaluated males and females in basic combat training and noted no increased risk of female cadets with higher BMIs. Ostenberg and Roos37 also found no correlation with BMI and injury in female soccer players. Thus, although BMI has not been shown in the literature to have a profound impact on increasing ACL injury risk, there are other risk factors associated, such as improper lower extremity positioning during landing, which may play a more prominent role in ACL injury.
Pubertal development and maturation is a distinct point when females become more at risk for ACL injuries than males. There is no evidence for a gender discrepancy in ACL injury rates in prepubescent athletes.34,38,39 Knee injuries do occur in pediatrics, and the percentage of those presenting as ACL injuries may be rising. And although ACL injuries are on the rise in both male and female pubescent athletes, females have a higher rate of ACL injuries immediately after their growth spurt.40 The growth of the tibia and femur is rapid around puberty in both genders.41 As these two bones grow rapidly, the knee is subject to greater torques.42 Males and females show differing neuromuscular activation patterns during and following puberty42 with females showing decreased adaptation after puberty.28 Males increase power, strength, and coordination with age and maturational stage which allows increased dynamic lower extremity control. Females do not follow the same postpubertal course. Thus, the growth and development during and following puberty related to neuromuscular, biomechanical, and hormonal factors are likely responsible for the differences in ACL injury risk based on age and gender.
Another widely studied risk factor for female ACL injury relates to the increased Q angle of females. The Q angle is formed by a line from the anterior superior iliac spine (ASIS) to the middle of the patella and then another line from the middle of the patella to the tibial tubercle. Some authors have reported that a higher Q angle may alter lower limb mechanics and place the knee at risk for higher static and dynamic valgus stresses.43,44 Shambaugh et al.45 reported a higher incidence of knee injuries in female basketball players with higher Q angles. Other authors, however, have suggested that a static measurement of the Q angle is not predictive of either knee valgus angles, neuromuscular patterns, or ACL injury risk during movement.32,46 Soderman et al.47 studied Q angle effect on leg injuries in female soccer players and noted no increase in leg injuries. Pantano et al.48 showed that peak knee valgus in a single-leg squat was not significantly greater in collegiate athletes with high or low Q angles. Pantano et al.48 also noted that a better ratio to predict valgus knee was pelvic width to femoral length ratio. This ratio was related to both static and dynamic knee valgus. Overall, static measurements such as Q angle do not appear to be entirely predictive of knee valgus or ACL risk, but this leaves the door open for evaluation and emphasis on dynamic neuromuscular factors and the role of muscle activation and control in the landing, pivoting, and cutting maneuvers known to induce ACL injury.32,46
Females have increased joint laxity and flexibility compared to males.47,49 Uhorchak et al.33 reported that females with generalized joint laxity had a 2.7 times greater risk of ACL injury than those without joint laxity. Generalized joint laxity and hyperextension were found to increase the risk of injury in female soccer players.47 Joint laxity is defined not only in the sagittal plane as hyperextension but also in the coronal plane as valgus which both can lead to strain in the ACL and possibly an increased risk of ACL injury.28,33,49–51
Notch width differences between males and females have been proposed as a risk factor for ACL injury. Uhorchak et al.33 reported a 16.8 times greater risk ratio for women with a smaller intercondylar notch (<13 mm) than those with a larger notch. Emerson52 hypothesized that a narrower notch width was a predisposing factor for ACL injury because it resulted in a smaller, weaker ligament. Shelbourne et al.53 agreed that notch width was indicative of a smaller ACL and that more females than males had smaller, narrower notches. Dienst et al.54 reported an in vivo MRI study that there was a correlation with smaller ACL cross-sectional area with smaller notch surface area and postulated an increase in ACL injury with smaller notches because of impingement of the ACL in the notch with tibial external rotation and abduction. Although many have accepted this theory regarding notch size as a risk factor for ACL injury, others refute that there is an association between notch width and ACL injury or that there are smaller notches in females compared to males when the notch width is normalized to bone width in both genders.1,28,55,56
Hamstring flexibility has been postulated as an additional risk factor for the increased ACL rates in females. Hamstring muscles were significantly more lax in ACL-injured athletes than matched controls.49 Hamstring flexibility is a marker for overall flexibility, with males and females showing divergent flexibility patterns after puberty. Males show decreased flexibility with age and maturation, whereas females have increased flexibility with puberty.57 After the pubertal growth spurt with significant changes in height and weight, males have decreased flexibility, whereas females do not,58 coincident with the time that ACL injury rates increase in females.
Increased hamstring flexibility in females may be responsible for the decreased dynamic control of the knee in adolescent female athletes.59,60 A potential mechanism is that lax hamstrings may lead to a delay in hamstring muscle activation. During early foot strike, hamstring and quadriceps cocontraction is required. It is postulated that with increased hamstring flexibility and laxity, hamstring activation may be delayed or absent, resulting in abnormalities in gait at foot strike.61 Further study is required to assess hamstring flexibility and laxity patterns during cutting and pivoting maneuvers in adolescent females; however, the disparity in female hamstring flexibility may be a potential risk factor for increased ACL injury in adolescent females.
The primary restraint to anterior translation of the tibia on the femur is the ACL.51
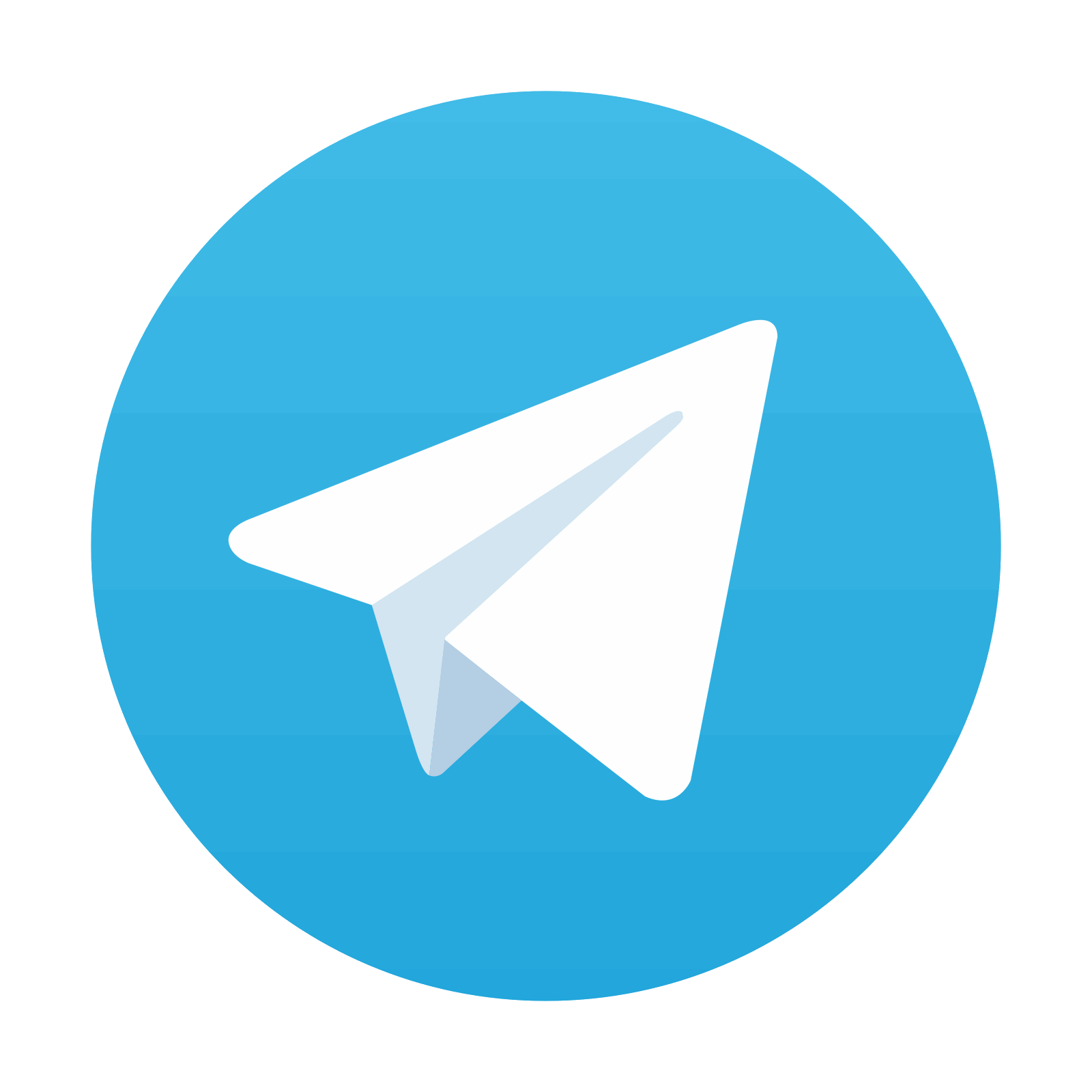
Stay updated, free articles. Join our Telegram channel

Full access? Get Clinical Tree
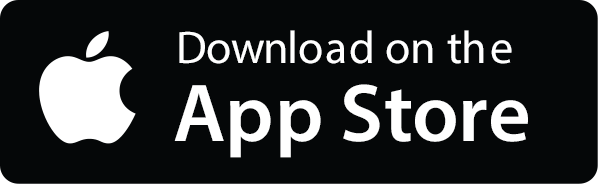
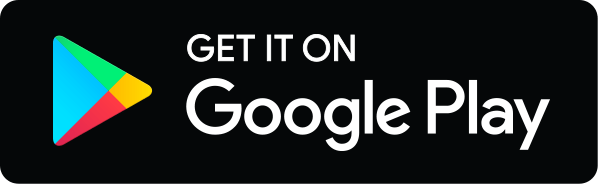