Background
Prenatal alcohol exposure leads to impaired fetal growth, brain development, and stillbirth. Placental impairment likely contributes to these adverse outcomes, but the mechanisms and specific vasoactive effects of alcohol that links altered placental function to impaired fetal development remain areas of active research.
Objective
Recently, we developed magnetic resonance imaging techniques in nonhuman primates to characterize placental blood oxygenation through measurements of T 2 * and perfusion using dynamic contrast-enhanced magnetic resonance imaging. The objective of this study was to evaluate the effects of first-trimester alcohol exposure on macaque placental function and to characterize fetal brain development in vivo.
Study Design
Timed-pregnant Rhesus macaques (n=12) were divided into 2 groups: control (n=6) and ethanol exposed (n=6). Animals were trained to self-administer orally either 1.5 g/kg/d of a 4% ethanol solution (equivalent to 6 drinks/d) or an isocaloric control fluid from preconception until gestational day 60 (term is G168). All animals underwent Doppler ultrasound scanning followed by magnetic resonance imaging that consisted of T 2 * and dynamic contrast-enhanced measurements. Doppler ultrasound scanning was used to measure uterine artery and umbilical vein velocimetry and diameter to calculate uterine artery volume blood flow and placental volume blood flow. After noninvasive imaging, animals underwent cesarean delivery for placenta collection and fetal necropsy at gestational day 110 (n=6) or 135 (n=6).
Results
Fetal weight and biparietal diameter were significantly smaller in ethanol-exposed animals compared with control animals at gestational day 110. By Doppler ultrasound scanning, placental volume blood flow was significantly lower ( P =.04) at gestational day 110 in ethanol-exposed vs control animals. A significant reduction in placental blood flow was evident by dynamic contrast-enhanced magnetic resonance imaging. As we demonstrated recently, T 2 * values vary throughout the placenta and reveal gradients in blood deoxyhemoglobin concentration that range from highly oxygenated blood (long T 2 * ) proximal to spiral arteries to highly deoxygenated blood (short T 2 * ). Distributions of T 2 *throughout the placenta show significant global reduction in T 2 * (and hence high blood deoxyhemoglobin concentration) in ethanol-exposed vs control animals at gestational day 110 ( P =.02). Fetal brain measurements indicated impaired growth and development at gestational day 110, but less so at gestational day 135 in ethanol-exposed vs control animals.
Conclusion
Chronic first-trimester ethanol exposure significantly reduces placental perfusion and oxygen supply to the fetal vasculature later in pregnancy. These perturbations of placental function are associated with fetal growth impairments. However, differences between ethanol-exposed and control animals in placental function and fetal developmental outcomes were smaller at gestational day 135 than at gestational day 110. These findings are consistent with placental adaptation to early perturbations that allow for compensated placental function and maintenance of fetal growth.
In the United States, approximately 40% of women consume alcohol in pregnancy, and >3 million women are at risk of exposing their fetus to alcohol because more than one-half of all pregnancies are unplanned and most women do not recognize they are pregnant until 4–6 weeks after conception. Of significance, ethanol readily crosses the placenta and accumulates in the fetus at concentrations proportionate to maternal blood levels within an hour. Previous pregnant ovine, baboon, and perfused human placenta models have shown that uterine blood flow decreases after acute exposure to ethanol. In addition, other studies have demonstrated that prenatal ethanol exposure negatively affects fetal growth and increases the risk of stillbirth, fatty hepatic degeneration, and fetal alcohol syndrome. However, the mechanisms and specific vasoactive effects of alcohol exposure early in gestation that link placental perfusion and oxygenation to impaired fetal development are not known.
Alcohol exposure has been shown in vitro to produce dose-dependent placental vasoconstriction that increases fetal-placental vascular resistance and placental perfusion pressure that results in impaired oxygen transport. The existing literature is limited and consists mostly of in vitro studies of the placenta. Furthermore, human studies are limited by current imaging capabilities to assess placental function in vivo in human subjects. Traditionally, Doppler ultrasound (Doppler-US) has been used to query major maternal vessels that support the placenta for clinical antenatal surveillance. However, this method cannot be used to assess blood flow within the placental intervillous space, which is the site of maternal-fetal oxygen and nutrient exchange. Recent work with pregnant nonhuman primates (NHP) has identified magnetic resonance imaging (MRI)–based methods to characterize maternal blood flow and oxygen exchange in the placenta with the fetal vasculature. Maternal perfusion of the placenta can be quantified with the use of dynamic contrast-enhanced MRI (DCE-MRI), which requires intravenous administration of an MRI contrast reagent. Although we have shown that maternal gadolinium (Prohance) administration in Rhesus macaques results in minimal fetal exposure, methods for antenatal in vivo hemodynamic assessment that does not require gadolinium-based contrast reagents are clinically desirable. Another method, which involves the analysis of endogenous MRI contrast provided by water T 2 * values, can quantify maternal blood oxygenation through the blood oxygen level-dependent effect. This provides a safe alternative to standard MRI contrast agents for future clinical use in human subjects.
The NHP model provides a powerful translational model for human pregnancy studies; NHPs have a gestational term and developmental ontogeny similar to humans, which includes its placental structure and function. Further benefits to studying NHPs are the similar rates of absorption and metabolism of ethanol to humans and the ability to induce oral self-administration of preset doses of ethanol. Maternal smoking, nutritional inadequacy, medication or illicit drug use, which are all factors that can have a synergistic effect on alcohol exposure, often confound human studies of placental impairment. Modeling human drinking behavior with NHP models provides the added advantage of a precise alcohol history while retaining the normal route of alcohol administration as opposed to strategies that are used to study fetal ethanol exposure in rodents. Further, voluntary ethanol self-administration also obviates the need for procedures such as gavage, which introduce confounders that are associated with increased maternal stress. This study focuses on the effects of chronic early alcohol exposure on placental perfusion and fetal oxygen availability and development in a relevant translational model. We hypothesized that alcohol exposure early in pregnancy would impair maternal perfusion of the placenta and would result in decreased tissue oxygenation.
Materials and Methods
Experimental design
A cohort of time-mated pregnant control Rhesus macaques (n=12) were divided into 2 groups: control (n=6) and ethanol exposed (n=6). Dams were trained to orally self-administer either 1.5 g/kg/d of 4% ethanol solution (equivalent to 6 drinks/day) or an isocaloric control fluid after a previously described 4-month schedule induced polydipsia procedure. After drinking induction, plasma estradiol and progesterone levels were sampled daily until ovulation was suspected, at which point dams were paired with male animals. Plasma estradiol was determined daily; once a decline from peak value was observed, the pairing phase was concluded. The day of peak plasma estradiol was defined to be gestational day 0 (G0). Each pregnant animal continued drinking 1.5 g/kg/d ethanol until G60 (term is G168), at which point access to ethanol, or isocaloric solution, was removed. A weekly maternal weight was collected for all animals throughout pregnancy. All animals completed an unsedated prenatal ultrasound examination at G50 to measure the fetal biparietal diameter. Subsequently, all animals underwent Doppler-US followed by MRI that consisted of T 2 * and DCE measurements. After noninvasive imaging, on the same day, animals underwent immediate cesarean delivery for placenta collection and fetal necropsy at G110 (n=6) or G135 (n=6). Placental and fetal brain tissue was collected, weighed, and measured after delivery.
Imaging
Doppler-US
All G50 prenatal ultrasound imagings were performed on awake animals by a single sonographer (J.O.L). Before terminal Doppler-US, animals were sedated by intramuscular administration of 10 mg/kg ketamine (Henry Schein Animal Health, Dublin, OH). Sedation was maintained on a portable anesthesia delivery system that provided O 2 with 1.5% isoflurane. Doppler-US measurements were collected by a sonographer (J.O.L) who used image-directed pulsed and color Doppler equipment (GE Voluson 730; GE, Vienna, Austria) with a 5- to 9-MHz sector probe. The lowest high-pass filter level was used (100 Hz), and an angle of ≤15 degrees between the vessel and the Doppler beam was deemed acceptable. Blood flow velocity waveforms were obtained from the proximal portion of the uterine artery (Uta), as previously described. Doppler waveform measurements for the Uta and the umbilical artery were performed and averaged over 3 cycles with the use of machine-specific software; the following measurements were obtained: pulsatility index, velocity time integral (VTI), and fetal heart rate (HR). The diameter of the Uta was measured with the use of power angiography, as previously described. The cross-sectional area (CSA) of the vessel was calculated: CSA = π(diameter/2) 2 . Uta volume blood flow (cQ Uta ) was calculated with the following formula and corrected by maternal weight: cQ Uta = VTI×CSA×HR. For the placental volume blood flow (cQ UV ), the Doppler waveforms were obtained from the straight portion of the intraabdominal umbilical vein, as previously described. The mean velocity (V mean ) was calculated as 0.5 of the maximum velocity. cQ UV was calculated with the following formula: V mean ×CSA×60.
MRI
Immediately after the ultrasound procedures, MRI studies were performed on a nonhuman primate-dedicated 3T Siemens TIM-Trio scanner (Erlangen, Germany) with a circularly polarized transmit, 15-channel receive radiofrequency “extremity” coil (QED, Cleveland, OH) to acquire T 2 -weighted images of the fetal brain, as described previously. For the analyses of the cerebral cortex, surface models of the interface between cerebral spinal fluid and cortical gray matter (the pial cortical surface) was generated with the use of the CARET software package ( http://brainvis.wustl.edu ). Surface area of each hemisphere was measured with the use of standard functionalities of the CARET program. Placenta MRI followed previously described procedures. Anatomic and multi echo imaging was performed during expiratory breath holding, which was achieved by temporarily suspending ventilation; DCE-MRI data were acquired during ventilated breathing. Physiologic monitoring of pulse rate, arterial blood oxygen saturation, and end-tidal CO 2 partial pressure was performed throughout the imaging study no deviations from normal ranges were observed in these parameters. Spiral artery flow from DCE-MRI data was measured as described previously in Frias et al, and the flow permeability ratio, F /( νPS ), was determined as described in Schabel et al.
Statistical analysis
Data are expressed as mean±standard deviation. All animals (n=12) were analyzed; differences between ethanol-exposed and control animals at G110 and G135 were tested by a nonpaired t -test. The T 2 * results were evaluated with the use of a 2-sample Kolmogorov-Smirnov test.
Results
Growth parameters
At G50, the fetal biparietal diameter that was measured on average at 1.24±0.02 cm on ultrasound scanning of all the animals in both treatment groups was appropriate for gestational age. Subsequent ultrasound scans that were performed at G110 were notable for a significantly smaller fetal biparietal diameter ( P <.05) and femur length ( P <.01) in ethanol-exposed animals compared with control animals ( Table 1 ). Such differences were not observed at G135. Biometry results were reflected in the fetal birth weight; ethanol-exposed fetuses weighed significantly less than control animals at both G110 and G135 ( P <.05; Table 2 ). Maternal and placental weights were not significantly different across treatment groups ( Table 2 ). Fetal gender ratio was also not significantly different ( Table 2 ).
Parameter | Gestational day 110, mean±standard deviation | Gestational day 135, mean±standard deviation | ||
---|---|---|---|---|
Control (n=3) | Ethanol (n=3) | Control (n=3) | Ethanol (n=3) | |
Biparietal diameter, mm | 39±0.9 | 35±1.1 a | 45±0.6 | 44±1.4 |
Abdominal circumference, cm | 12±0.2 | 11±0.8 | 14±0.4 | 13±1.9 |
Femur length, mm | 29±0.4 | 26±0.9 b | 37±1.9 | 36±0.5 |
Parameter | Gestational day 110 | Gestational day 135 | ||
---|---|---|---|---|
Control (n=3) | Ethanol (n=3) | Control (n=3) | Ethanol (n=3) | |
Maternal weight, kg a | 7.5±1.7 | 8.3±1.4 | 7.5±0.9 | 8.5±1.1 |
Fetal weight, g a | 217±21 | 175±8.4 b | 333±6.5 | 318±3.6 b |
Placental weight, g a | 75±17 | 65±4.1 | 78±2.3 | 83±10.3 |
Fetal sex (male:female) | 1:2 | 0:3 | 1:2 | 1:2 |
Placental perfusion and oxygenation
By Doppler-US, we demonstrated a significant reduction in cQ uv in the ethanol-exposed group compared with controls at G110 that was not observed at G135 ( Table 3 ). There was no significant difference in cQ u ta and Uta and umbilical artery pulsatility indices in either treatment group at both gestational ages ( Table 3 ). Maternal perfusion of the placental intervillous space was evaluated with the use of DCE-MRI and T 2 * measurements, and this revealed differences between ethanol-exposed animals and controls. Through the analysis of DCE-MRI measurements, it is possible to identify individual spiral arteries and to quantify maternal volume blood flow ( F ) into the placenta through each of them. The total volumetric blood flow to all placental cotyledons was decreased in the ethanol-exposed group vs controls ( Table 3 ), which was suggested by the semiquantitative measures of the cQ u ta , in animals that were evaluated at G110, but not G135. Figure 1 shows images that reflect perfusion for a representative ethanol-exposed case and for a representative control case. Further, through the analysis of water T 2 * values throughout the placenta, it is possible to quantify the flow-permeability ratio, F /( νPS ), which relates to spiral artery flow and oxygen delivery to the fetal blood (through the volume fraction of the intervillous space [ν] and the oxygen permeability surface area product [ PS ]) on an individual spiral artery basis. In control placentas at both G110 and G135, magnetic resonance image voxels that are proximal to spiral artery sources of oxygenated maternal blood are characterized by relatively long T 2 * values, as previously reported. At greater distances from the spiral arteries, the concentration of deoxyhemoglobin is higher, because of fetal oxygen uptake, and results in shorter T 2 * values. The histograms shown in Figure 2 summarize placental T 2 *by demonstrating a statistically significant ( P =.02) reduction in values at G110 in the ethanol-exposed cases compared with control animals. Similar to the DCE-MRI data, the difference in T 2 * that were observed at G135 was not statistically significant ( P =.39). Median F /( νPS ) ratios are smaller in ethanol-exposed animals than in controls at both G110 and G135; however, because of the influence of additional factors in the analysis of T 2 * that contribute to interanimal variation in F /( νPS ), such as the number of spiral arteries, significant differences in F /( νPS ) were not observed ( Table 3 ).
Parameter | Gestational day 110, mean±standard deviation | Gestational day 135, mean±standard deviation | ||
---|---|---|---|---|
Control (n=3) | Ethanol (n=3) | Control (n=3) | Ethanol (n=3) | |
Uterine artery pulsatility index | 0.65±0.1 | 0.82±0.1 | 0.86±0.3 | 0.8±0.01 |
Umbilical artery pulsatility index | 1.22±0.1 | 1.7±0.3 | 1.15±0.1 | 1.35±0.5 |
Uterine artery blood flow, mL/min/kg | 48±23 | 19±11 | 34±22 | 27±0.1 |
Total placental blood flow, mL/min a | 681±40 | 284±142 b | 558±189 | 501±166 |
Median F /( νPS ), cm 3 | (4.4±0.5)×10 −6 | (0.69±0.08)×10 −6 | (1.7±0.2)×10 −6 | (1.0±0.4)×10 −6 |
a Obtained by dynamic contrast-enhanced magnetic resonance imaging
b P <.01. F/(vPS) = flow permeability ratio, where F = maternal volume blood flow, v = volume fraction of the intervillous space, PS = oxygen permeability surface area.
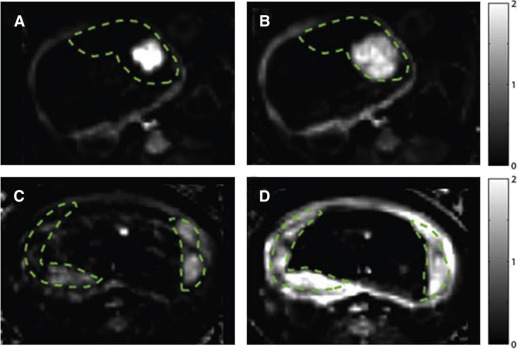
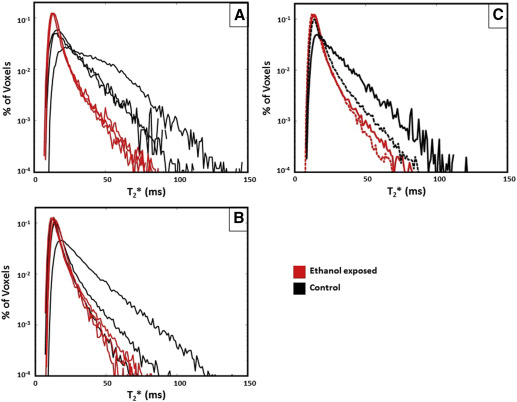
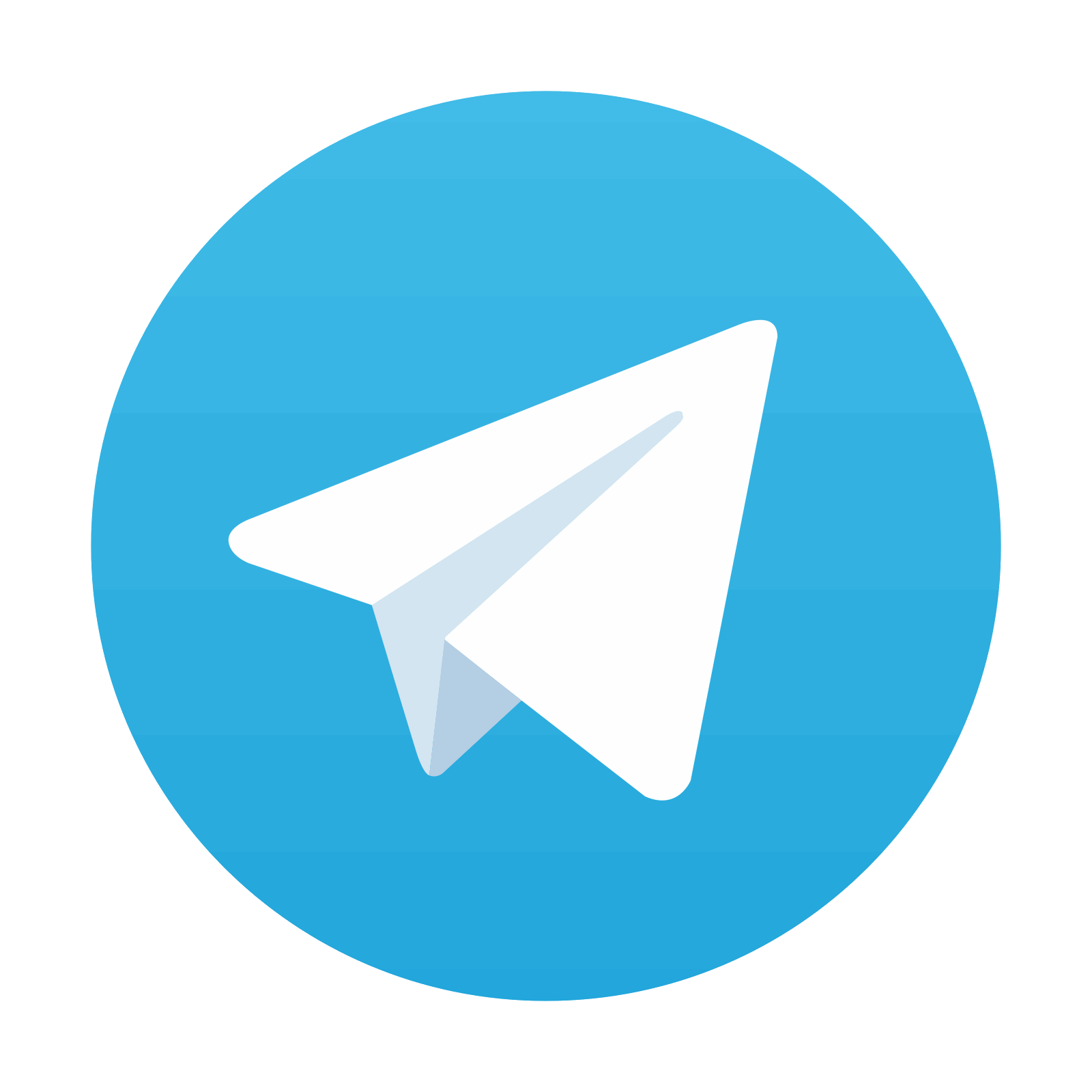
Stay updated, free articles. Join our Telegram channel

Full access? Get Clinical Tree
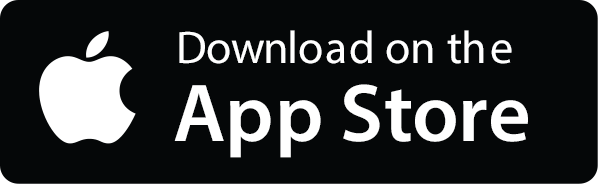
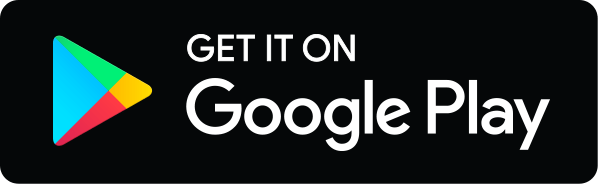