Background
Large-for-gestational-age fetuses are at increased risk of perinatal morbidity and mortality. Magnetic resonance imaging seems to be more accurate than ultrasound in the prediction of macrosomia; however, there is no well-powered study comparing magnetic resonance imaging with ultrasound in routine pregnancies.
Objective
This study aimed to prospectively compare estimates of fetal weight based on 2-dimensional ultrasound and magnetic resonance imaging with actual birthweights in routine pregnancies.
Study Design
From May 2016 to February 2019, women received counseling at the 36-week clinic. Written informed consent was obtained for this Ethics Committee-approved study. In this prospective, single-center, blinded study, pregnant women with singleton pregnancies between 36 0/7 and 36 6/7 weeks’ gestation underwent both standard evaluation of estimated fetal weight with ultrasound according to Hadlock et al and magnetic resonance imaging according to the formula developed by Baker et al, based on the measurement of the fetal body volume. Participants and clinicians were aware of the results of the ultrasound but blinded to the magnetic resonance imaging estimates. Birthweight percentile was considered as the gold standard for the ultrasound and magnetic resonance imaging–derived percentiles. The primary outcome was the area under the receiver operating characteristic curve for the prediction of large-for-gestation-age neonates with birthweights of ≥95th percentile. Secondary outcomes included the comparative prediction of large-for-gestation-age neonates with birthweights of ≥90th, 97th, and 99th percentiles and small-for-gestational-age neonates with birthweights of ≤10th, 5th, and 3rd percentiles for gestational age and maternal and perinatal complications.
Results
Of 2914 women who were initially approached, results from 2378 were available for analysis. Total fetal body volume measurements were possible for all fetuses, and the time required to perform the planimetric measurements by magnetic resonance imaging was 3.0 minutes (range, 1.3–5.6). The area under the receiver operating characteristic curve for the prediction of a birthweight of ≥95th percentile was 0.985 using prenatal magnetic resonance imaging and 0.900 using ultrasound (difference=0.085, P <.001; standard error, 0.020). For a fixed false-positive rate of 5%, magnetic resonance imaging for the estimation of fetal weight detected 80.0% (71.1–87.2) of birthweight of ≥95th percentile, whereas ultrasound for the estimation of fetal weight detected 59.1% (49.0–68.5) of birthweight of ≥95th percentile. The positive predictive value was 42.6% (37.8–47.7) for the estimation of fetal weight using magnetic resonance imaging and 35.4% (30.1–41.1) for the estimation of fetal weight using ultrasound, and the negative predictive value was 99.0% (98.6–99.3) for the estimation of fetal weight using magnetic resonance imaging and 98.0% (97.6–98.4) for the estimation of fetal weight using ultrasound. For a fixed false-positive rate of 10%, magnetic resonance imaging for the estimation of fetal weight detected 92.4% (85.5–96.7) of birthweight of ≥95th percentile, whereas ultrasound for the estimation of fetal weight detected 76.2% (66.9–84.0) of birthweight of ≥95th percentile. The positive predictive value was 29.9% (27.2–32.8) for the estimation of fetal weight using magnetic resonance imaging and 26.2% (23.2–29.4) for the estimation of fetal weight using ultrasound, and the negative predictive value was 99.6 (99.2–99.8) for the estimation of fetal weight using magnetic resonance imaging and 98.8 (98.4–99.2) for the estimation of fetal weight using ultrasound. The area under the receiver operating characteristic curves for the prediction of large-for-gestational-age neonates with birthweights of ≥90th, 97th, and 99th percentiles and small-for-gestational-age neonates with birthweights of ≤10th, 5th, and 3rd percentiles was significantly larger in prenatal magnetic resonance imaging than in ultrasound ( P <.05 for all).
Conclusion
At 36 weeks’ gestation, magnetic resonance imaging for the estimation of fetal weight performed significantly better than ultrasound for the estimation of fetal weight in the prediction of large-for-gestational-age neonates with birthweights of ≥95th percentile for gestational age and all other recognized cutoffs for large-for-gestational-age and small-for-gestational-age neonates ( P <.05 for all).
Introduction
Prenatal estimation of fetal weight (EFW) began in the 1960s, during the very early days of obstetrical ultrasound. The estimations were initially based on abdominal circumference with biparietal diameter being added some years later. , In 1984 and 1985 Hadlock et al , introduced an algorithm for the prediction based on the measurement of biparietal diameter, head circumference, abdominal circumference, and femur length. More than 3 decades later and despite all efforts to develop new models for the EFW, the algorithm described by Hadlock et al , remains the most accurate method of birthweight prediction. Unfortunately, this algorithm is still relatively imprecise, identifying only 73% of large-for-gestational-age (LGA) neonates with birthweights (BWs) of >95th percentile for a fixed false-positive rate (FPR) of 10%.
Why was this study conducted?
This study aimed to determine whether prenatal magnetic resonance imaging performs better than ultrasound in the prediction of large-for-gestational-age (LGA) and small-for-gestational-age neonates.
Key findings
In pregnant women presenting at 36 0/7 to 36 6/7 weeks’ gestation and for a fixed false-positive rate of 5%, magnetic resonance imaging detected 80.0% of women with a birthweight (BW) of ≥95th percentile, whereas ultrasound detected 59.1% of women with a BW of ≥95th percentile (primary outcome). Moreover, magnetic resonance imaging detected 91.6% of women with a BW of ≤5th percentile, whereas ultrasound detected 57.8% women with a BW of ≤5th percentile (secondary outcome).
What does this add to what is known?
This study showed strong evidence that prenatal magnetic resonance imaging performs significantly better than ultrasound in the prediction of LGA neonates with BWs of ≥95th percentile and all other recognized cutoffs for LGA and small-for-gestational-age neonates at 36 weeks’ gestation ( P <.05 for all).
The purpose of the EFW is to follow serial growth and identify fetuses at the extremes, either small-for-gestational-age (SGA) or LGA neonates. Both these groups have higher rates of morbidity and mortality; therefore, once identified, managing clinicians can modify the timing and/or mode of birth to optimize clinical outcomes and minimize perinatal risks.
Magnetic resonance imaging (MRI)-derived EFW was first introduced in 1984 by Baker et al, but the method was both complex and time-consuming, which explains why not many papers have been published on the topic. Over the last decade, we have managed to introduce and validate a semiautomated method that allows a reproducible, rapid, and precise method of EFW, which accurately predicts SGA and LGA, whether the estimations are performed close to or remote from delivery. However, to date, there is no publication of large prospective studies for routine pregnancies.
In this blinded prospective study, Predict Neonatal Macrosomia (PREMACRO) study, we tested the hypothesis that MRI-EFW performs better than standard ultrasound EFW (US-EFW), based on Hadlock et al, , in the prediction for LGA with a BW of ≥95th percentile in a large, unselected population of women at 36 weeks’ gestation. Moreover, we evaluated the performance of MRI-EFW and US-EFW in the prediction of LGA and SGA at various cutoffs.
Materials and Methods
Study design and population
This was a prospective, noninterventional, single-center, blinded study, which compared the prediction of singleton LGA neonates (defined as neonates with a BW of ≥95th percentile for gestational age) using prenatal US and MRI at between 36 0/7 and 36 6/7 weeks’ gestation. We enrolled pregnant women who were attending a 36-week growth clinic at the Department of Obstetrics and Gynecology of the University Hospital Brugmann, Brussels, Belgium.
The inclusion criteria for the study were age of 18 years or older, singleton pregnancy, live fetus at 36 0/7 to 36 6/7 weeks’ gestation, planning for a delivery in our maternity ward at the University Hospital Brugmann, Brussels, Belgium. The exclusion criteria were as follows: unconscious or severely ill women, those with learning difficulties or mental handicap, multiple pregnancy, major fetal abnormality identified at routine first-, second-, or third-trimester scans, no first-trimester scan (risk of imprecise dating), presentation outside the target gestational age window, known contraindication to MRI, painful regular uterine contractions or history of ruptured membranes, patients already recruited in a previous pregnancy, women delivering before MRI and US evaluation, neonatal weight measured >6 hours after the birth for any reason, patients with history of stillborns.
Potential study candidates were given a participant information sheet concerning the research, and written informed consent was obtained from all those who agreed to recruitment. Ethical approval for the study was obtained from the local research ethics committee (CE 2016/44), University Hospital Brugmann, Brussels, Belgium. The study is registered on ClinicalTrials.gov (number NCT02713568 ).
Prenatal ultrasound examination
All women underwent prenatal US examinations using transabdominal sonography by 1 of 4 experienced consultants in maternal-fetal medicine. US-EFW was obtained according to Hadlock et al, , based on measurements of biparietal diameter, head circumference, abdominal circumference, and femoral length. US was performed on Voluson E8 machines (GE Medical Systems, Zipf, Austria). A percentile for the US-EFW was obtained after plotting the weight estimation on the curves, as described by Yudkin et al. Ultrasound data were entered into the astraia software gmbh (Munich, Germany). The participants, general practitioners, obstetricians, and midwives of the patients had access to the results of US-EFW, which was used for clinical management.
Magnetic resonance imaging examination
Within 15 minutes of US, the women underwent an MRI examination to measure fetal body volume (FBV). Details of the MRI-EFW method have been described previously. Briefly, MRI was performed using a clinical 1.5 T whole-body unit with a gradient field strength of 45 mT/m. No maternal sedation was administered, and the mother was not asked to breath-hold. Women were scanned in the supine position, with a combination of a 6-channel phased-array body and 6 elements of the spine coil positioned in the lower pelvic area.
The MRI protocol consists of a “scout” scan to gather information about the orientation of the fetus. Subsequently, we recorded T2-weighted imaging using fast imaging with steady-state free procession (TrueFISP) sequences in the fetal sagittal plane: 9 to 15 adjacent slices (average adjusted) according to fetal size with a 4-mm slice thickness, an intersection gap of 20, a field of view of 380×309 mm 2 , a matrix of 166×256, repetition time/echo time=4.65 ms/2.33 ms, resulting voxel resolution of 1.9×1.5×4.0 mm 3 , and a bandwidth of 399 Hz/pixel.
For further research, other sequences were acquired in the following order: TrueFISP sequences in the fetal sagittal plane with a 4-mm slice thickness and an intersection gap of 4 mm, followed by sequences for pelvimetric measurements.
MRI was performed using 1 of 2 magnets: Magnetom Avanto with a bore diameter of 60 cm or Aera with a bore diameter of 70 cm (Siemens Healthcare GmbH, Erlangen, Germany). Sequences degraded by fetal motion or following maternal movements were repeated using the same parameters. For the PREMACRO study, total examination time was a maximum of 5 minutes.
MRI-EFW was defined as successful if the women did not feel any discomfort during the MRI or felt discomfort but the acquisition of the main sequence was successful and an MRI planimetric measurement was possible. Conversely, MRI-EFW was defined as a failure in any of the following circumstances: women who could not be accommodated in the machine because of a high body mass index (BMI), MRI impossible because of claustrophobia or discomfort resulting in interruption of the examination before the main sequence could be performed, any absolute contraindication to MRI, and examination was discontinued because of a complication related to the magnet.
Total FBV planimetric measurements were made by 1 of 5 previously trained operators. FBV was measured either on the same day as the MRI examination or within 48 hours by the available operator, who used a semiautomated software on a picture archiving and communication system (PACS) (Impax, Agfa-Gevaert, Mortsel, Belgium) ( Figure 1 ).

The operators performing FBV measurements were blinded to the US-EFW results. FBV measurements were entered in the astraia database with access limited to the operators performing FBV measurements. MRI-EFW was calculated using the equation 0.12+1.031×FBV (L)=MRI-EFW (kg) as developed by Baker et al. MRI-EFW was not entered in the astraia database but kept in a secure database by the principal investigator and transmitted to the independent data monitoring committee on a monthly basis. In contrast to the US-EFW, which was available for clinical use, the participants, general practitioners, obstetricians, and midwives of the patients were blinded to the results of the MRI-EFW. A percentile for the MRI-EFW was obtained after plotting the weight estimation on the curves as described by Yudkin et al.
Pregnancy and newborn outcomes
We aimed to measure neonatal weight immediately after birth or within 6 hours of delivery. A percentile for the birthweight was obtained after plotting the actual weight on the curves as described by Yudkin et al, and this was considered the gold standard comparator for the US-EFW and MRI-EFW–derived percentiles.
Data on pregnancy and neonatal outcomes were collected from our obstetrical electronic database MOSOS (BMA B.V., Houten, the Netherlands) and hospital maternity records. For neonates admitted to the neonatal intensive care unit (NICU), additional neonatal outcomes were collected from the discharge summaries.
Study outcomes
The primary outcome was the area under the receiver operating characteristic (ROC) curve (AUC) for the detection of LGA neonates with a BW of ≥95th percentile for gestational age based on MRI-EFW vs US-EFW in women with complete results for the 2 sets. Secondary outcomes included the comparison of MRI-EFW with US-EFW for the prediction of LGA neonates with a BW of ≥90th, 97th, and 99th percentile for gestational age and SGA neonates with a BW of ≤10th, 5th, and 3rd percentile for gestational age. Moreover, we evaluated the performance of MRI-EFW vs US-EFW to predict shoulder dystocia, fracture of the clavicle or a long bone, brachial plexus injury, intracranial hemorrhage, or death; neonatal morbidity (defined as arterial cord blood pH of <7.10, Apgar score of <7 at 5 minutes, admission to the NICU); or maternal morbidity (defined as cesarean delivery, operative vaginal delivery [vacuum or forceps], postpartum hemorrhage [>500 mL], blood transfusion, and anal sphincter tear).
Statistical analysis
The sample size estimation was based on the results of a pilot study previously conducted in our department before the PREMACRO study, assumptions concerning the performance of MRI-EFW (AUC, 0.981) and US-EFW (AUC, 0.921) in the prediction of neonatal macrosomia, and information regarding the prevalence of neonates born in our department with a BW of ≥95th percentile (available data from 5920 deliveries between 2011 and 2016). We determined that a sample size of 90 cases of macrosomic fetuses (≥95th percentile) and 2250 negative controls would provide a power of 90% to determine the primary outcome of detecting a difference between the AUC of MRI-EFW and US-EFW at a significance level of 5%.
MRI-EFW and US-EFW each produce a measured value and therefore classify the fetus as being either macrosomic or not. The ROC curve is generated by computing sensitivity and specificity for each technique (MRI and US) compared with the actual classification of a neonate being macrosomic or not at birth. The differences between the ROC curves are calculated taking into account the paired nature of the data. AUC values were compared with the use of a z test according to the method of DeLong et al. A P value of <.05 was considered to indicate statistical significance. Confidence intervals were computed with the use of the Clopper-Pearson method. The exact binomial test for paired comparisons was used in sensitivity and specificity and the generalized score statistic to analyze the positive predictive value (PPV) and negative predictive value (NPV). We compared sensitivity, specificity, and PPV and NPV of MRI-EFW and US-EFW for the detection of neonatal macrosomia for fixed FPRs of 5% and 10%. The differences between the morbidity outcomes according to MRI-EFW and US-EFW were performed using the chi-squared test.
The statistical software packages R (R Project for Statistical Computing, https://www.r-project.org/ ) and MedCalc (version 15.11.4; MedCalc Software Ltd, Mariakerke, Belgium) were used for data analyses.
Results
Study participants
From May 2016 to February 2019, a total of 2914 women received counseling at the 36-week clinic. Of these women, 242 (8.3%) were excluded because they did not meet the eligibility criteria: 15 (0.5%) were <18 years old, 149 (5.1%) had imprecise pregnancy dating because of an absence of a first-trimester scan, 36 (1.2%) presented outside the gestational age window, 41 (1.4%) had already been included in the study in a previous pregnancy, and 1 presented with an in utero fetal death (IUFD). Of the 2672 eligible women, 2466 (92.2%) agreed to participate in the study. In addition, MRI-EFW failed in 2 women because of a high BMI—too large to enter the machine (48.6 and 46.4 kg/m 2 ), 67 women did not undergo MRI because of claustrophobia or discomfort resulting in interruption of the examination, 2 women had contraindications to MRI: 1 with metallic fragments inside a large tattoo and 1 carrying an integrated insulin reading device, and 2 underwent MRI but there was a problem with transmission of the image to the PACS and the examination was lost. Of the women who participated in the study, 13 were lost to follow-up and 2 ended with a stillborn (1 at 38.2 and 1 at 38.6 weeks’ gestation). After all exclusions, the primary analysis cohort included 2378 women ( Figure 2 ).

Baseline characteristics of the primary analysis cohort are outlined in Table 1 . Imaging was performed at a median gestational age of 36.3 weeks (range, 36.0–36.6), delivery occurred at a gestational age of 39.3 weeks (range, 37.0–42.1), and delivery weight was 3380 g (range, 1935–5020). Of the 2378 newborns, 200 (8.4%) had birthweights of ≥90th percentile, 105 (4.4%) ≥95th percentile, 62 (2.6%) ≥97th percentile, and 25 (1.1%) ≥99th percentile. In addition, 201 (8.5%) had BWs of ≤10th percentile, 83 (3.5%) ≤5th percentile, and 47 (2.0%) ≤3rd percentile.
Characteristic | Value |
---|---|
Number of patients | 2378 |
Maternal age (y) | |
Maternal weight before pregnancy (kg) | 68.0 (37.0–139.0) |
Body mass index before pregnancy (kg/m 2 ) | 25.5 (15.1–53.0) |
Maternal weight at the 36-wk scan (kg) | 79.0 (46.0–140.0) |
Weight gain in pregnancy (kg) | 10.0 (−9.0 to 29.0) |
Body mass index at the 36-wk scan (kg/m 2 ) | 29.4 (17.5–55.2) |
Nulliparity | 754 (31.70) |
Ethnicity | |
White or North African | 1940 (81.60) |
Central African | 397 (16.70) |
South Asian | 30 (1.30) |
East Asian | 6 (0.30) |
Mixed | 5 (0.20) |
Conception | |
Natural | 2335 (98.20) |
Assisted by use of ovulation drugs | 16 (0.70) |
In vitro fertilization | 27 (1.10) |
Cigarette smoker | 234 (9.80) |
Medical history | |
Diabetes mellitus type 1 | 7 (0.30) |
Diabetes mellitus type 2 | 18 (0.80) |
Gestational diabetes mellitus treated with diet only | 419 (17.60) |
Gestational diabetes mellitus treated with insulin | 161 (6.80) |
Untreated gestational diabetes mellitus | 4 (0.20) |
Any type of diabetes mellitus | 609 (25.60) |
Male fetus | 1210 (50.90) |
Mode of delivery | |
Planned cesarean delivery | 217 (9.13) |
Emergency cesarean delivery | 208 (8.75) |
Vaginal birth with instrumental delivery | 176 (7.40) |
Vaginal birth without instrumental delivery | 1777 (74.73) |
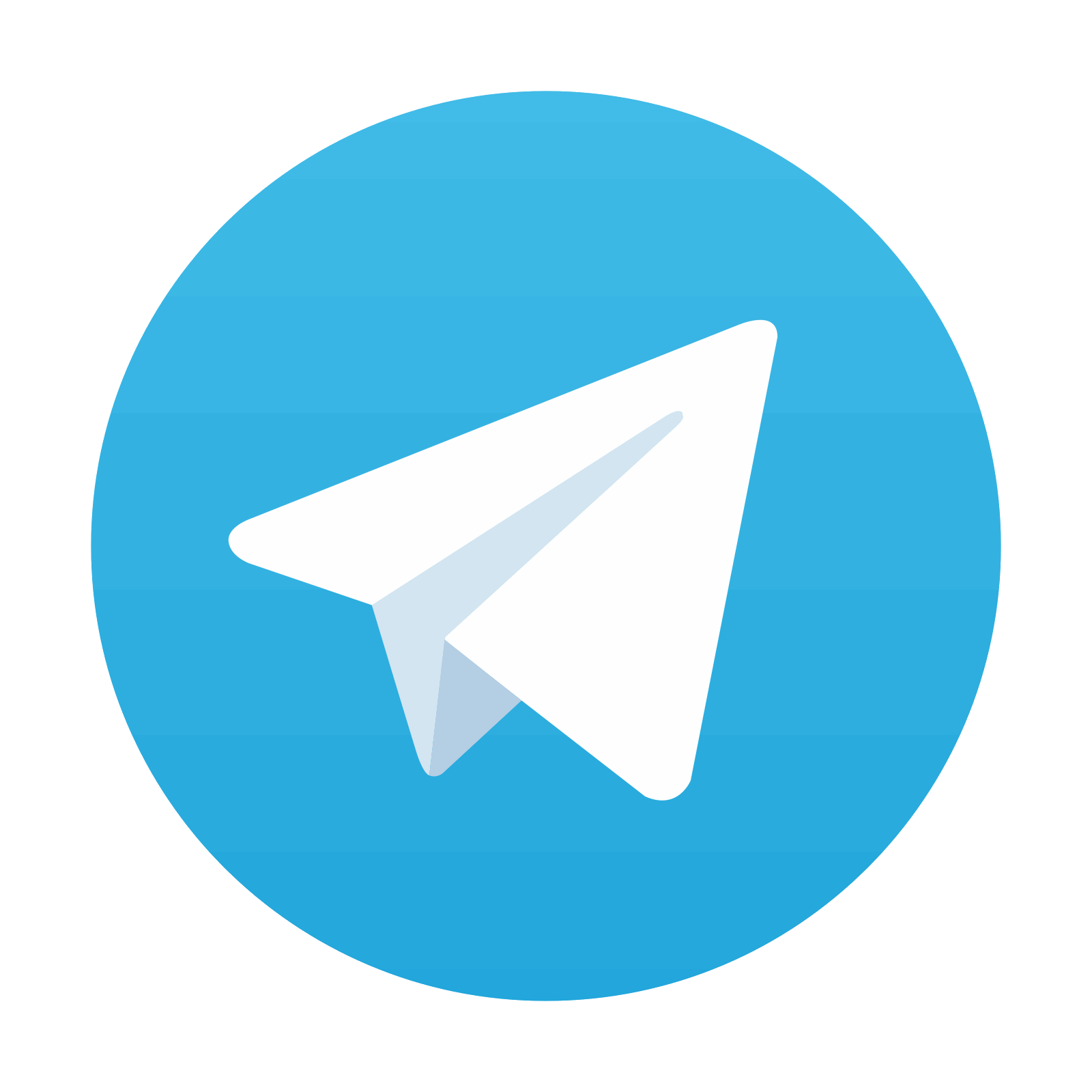
Stay updated, free articles. Join our Telegram channel

Full access? Get Clinical Tree
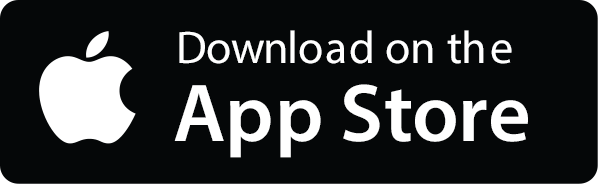
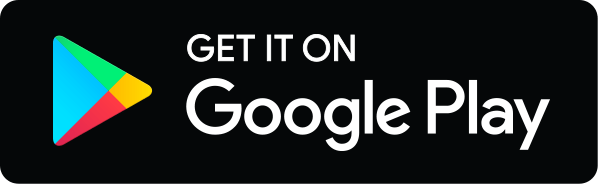