Background
Bacterial cells in the human body account for 1–3% of total body weight and are at least equal in number to human cells. Recent research has focused on understanding how the different bacterial communities in the body (eg, gut, respiratory, skin, and vaginal microbiomes) predispose to health and disease. The microbiota of the reproductive tract has been inferred from the vaginal bacterial communities, and the uterus has been classically considered a sterile cavity. However, while the vaginal microbiota has been investigated in depth, there is a paucity of consistent data regarding the existence of an endometrial microbiota and its possible impact in reproductive function.
Objective
This study sought to test the existence of an endometrial microbiota that differs from that in the vagina, assess its hormonal regulation, and analyze the impact of the endometrial microbial community on reproductive outcome in infertile patients undergoing in vitro fertilization.
Study Design
To identify the existence of an endometrial microbiota, paired samples of endometrial fluid and vaginal aspirates were obtained simultaneously from 13 fertile women in prereceptive and receptive phases within the same menstrual cycle (total samples analyzed n = 52). To investigate the hormonal regulation of the endometrial microbiota during the acquisition of endometrial receptivity, endometrial fluid was collected at prereceptive and receptive phases within the same cycle from 22 fertile women (n = 44). Finally, the reproductive impact of an altered endometrial microbiota in endometrial fluid was assessed by implantation, ongoing pregnancy, and live birth rates in 35 infertile patients undergoing in vitro fertilization (total samples n = 41) with a receptive endometrium diagnosed using the endometrial receptivity array. Genomic DNA was obtained either from endometrial fluid or vaginal aspirate and sequenced by 454 pyrosequencing of the V3–V5 region of the 16S ribosomal RNA (rRNA) gene; the resulting sequences were taxonomically assigned using QIIME. Data analysis was performed using R packages. The χ 2 test, Student t test, and analysis of variance were used for statistical analyses.
Results
When bacterial communities from paired endometrial fluid and vaginal aspirate samples within the same subjects were interrogated, different bacterial communities were detected between the uterine cavity and the vagina of some subjects. Based on its composition, the microbiota in the endometrial fluid, comprising up to 191 operational taxonomic units, was defined as a Lactobacillus -dominated microbiota (>90% Lactobacillus spp.) or a non- Lactobacillus -dominated microbiota (<90% Lactobacillus spp. with >10% of other bacteria). Although the endometrial microbiota was not hormonally regulated during the acquisition of endometrial receptivity, the presence of a non- Lactobacillus -dominated microbiota in a receptive endometrium was associated with significant decreases in implantation [60.7% vs 23.1% ( P = .02)], pregnancy [70.6% vs 33.3% ( P = .03)], ongoing pregnancy [58.8% vs 13.3% ( P = .02)], and live birth [58.8% vs 6.7% ( P = .002)] rates.
Conclusion
Our results demonstrate the existence of an endometrial microbiota that is highly stable during the acquisition of endometrial receptivity. However, pathological modification of its profile is associated with poor reproductive outcomes for in vitro fertilization patients. This finding adds a novel microbiological dimension to the reproductive process.
Related editorial, page 682 .
Click Supplemental Materials under article title in Contents at ajog.org
Introduction
Bacteria present in the urogenital tract make up 9% of the total human microbiota, and most of them are not easily culturable. The vaginal microbiota was first identified in 2002 by molecular methods used to detect nonculturable bacteria. A normal vaginal microbiota is defined by the presence of bacterial species (spp.) of the Lactobacillus genus that are commonly associated with a healthy genitourinary status. The vaginal microbiota typically changes throughout the menstrual cycle, depending on factors such as vaginal hygiene, sexual activity, use of intimate products, and underwear composition; greater microbiota stability is associated with the estradiol peak at ovulation and progesterone rise in the midluteal phase. However, alterations in the vaginal microbiota can lead to several pathologies. For example, bacterial vaginosis (BV) is a vaginal syndrome produced by the overgrowth of anaerobic bacteria such as Atopobium vaginae , Gardnerella vaginalis , Mobiluncus curtisii , and Mycoplasma hominis to the detriment of Lactobacillus spp.
Further, the vaginal microbiota has been shown to be different in pregnant and nonpregnant women in terms of stability and composition, demonstrating that the vaginal microbiota could have implications for reproductive and obstetrical processes. BV has been associated with obstetric complications including early and late miscarriage rates and preterm birth. Interestingly, a microbiological culture of the tip of the transfer catheter in patients undergoing in vitro fertilization (IVF) revealed that the presence of bacterial species in the uterine cavity at the time of embryo transfer negatively affects implantation and pregnancy rates. Indeed, Enterobacteriaceae spp., Streptococcus spp., Staphylococcus spp., Escherichia coli , and Gram-negative bacteria have been associated with decreased implantation rates and poor pregnancy outcomes, but no consensus has been reached regarding the origin and genus of bacterial pathogens and the mechanisms by which they could interfere with embryonic implantation. Importantly, prior studies were limited by the number of bacterial species that can be isolated and identified following culture of the catheter tip, and the potential risk of contamination of the catheter tip in the vagina/ectocervix/endocervix.
Although the vagina has long been known to contain microbes, the uterine cavity was classically considered a sterile organ. A report challenging this dogma suggested the existence of an endometrial microbiota comprising different microorganisms ( Lactobacillus spp., Mycoplasma hominis , Gardnerella vaginalis , and Enterobacter spp.) isolated by classic microbiological culture techniques of endometrial samples obtained from hysterectomy. Recently, the molecular identification of bacterial species in the endometrium of asymptomatic patients undergoing hysterectomy for benign indications confirmed that the uterine cavity is not sterile. Although a pathological infection is not always produced by the host-microbiota interactions, a murine model of ascending bacterial infection supports the concept that the endometrium might not be as sterile as thought. Given that changes in the human microbiota have been linked to various disease states, the potential for an endometrial microbiota that contributes to reproductive health merits investigation.
Here, we investigated the existence of a differentiated endometrial microbiota using 16S rRNA gene pyrosequencing, and assessed its hormonal regulation and potential functional impact on reproductive outcome in patients undergoing IVF. The findings imply a role for the endometrial microbiota in reproductive outcomes.
Materials and Methods
Study design
Three separate prospective pilot studies were performed. First, to analyze the existence of a differential endometrial microbiota, paired samples of endometrial fluid (EF) and vaginal aspirates (VA) were obtained simultaneously from 13 fertile women in their prereceptive (two days after the luteinizing hormone surge, known as LH+2) and receptive (seven days after the uteinizing hormone surge, also known as LH+7) phases in the same menstrual natural cycle (total samples analyzed n = 52). To investigate the hormonal regulation of the endometrial microbiota, EF samples (n = 44) were collected at LH+2 and LH+7 within the same natural cycle from 22 fertile women. Finally, the reproductive impact of an altered endometrial microbiota in EF was assessed by implantation, miscarriage, ongoing pregnancy, and live birth rates in infertile subjects undergoing IVF (n = 35) in whom a receptive endometrium was diagnosed using the endometrial receptivity array (ERA). EF samples were obtained in the cycle before embryo transfer. Approval for this study was obtained from the IVI Valencia Ethical Committee (project: 1404-FIVI-015-CS), and subjects provided written informed consent.
Subjects
For comparison between vaginal and endometrial bacterial communities and the study of hormonal regulation of the endometrial microbiota, samples were obtained within the natural cycles of women from the ovum donation program at IVI Valencia, Spain. The functional impact of the endometrial microbiota on reproductive outcome was explored in infertile subjects undergoing IVF treatment in whom a receptive endometrium was diagnosed by ERA (Igenomix SL, Valencia, Spain). A more detailed description is provided in Supplementary Methods .
EF aspiration
EF was obtained in all subjects as previously described.
VA collection
In the first pilot study, 20–80 μL of VA were collected from the posterior vagina before EF aspiration using a sterile catheter under direct vision.
Endometrial receptivity diagnosis
Endometrial receptivity was diagnosed using the ERA as previously described. A more detailed protocol is provided in Supplementary Methods .
Genomic DNA isolation from EF and VA samples
Isolation of DNA from frozen EF or VA was performed following the MagNa Pure compact nucleic acid isolation kit I (Roche, Madison, WI) protocol with modifications. A more detailed protocol is provided in Supplementary Methods .
Polymerase chain reaction and 16S rRNA sequencing
For sequencing and barcoding, the V3-V5 region of the 16S rRNA gene was amplified using key-tagged eubacterial primers, as previously described. Unidirectional pyrosequencing was carried out on a 454 Life Sciences GS FLX+ instrument (Roche) following the Roche Amplicon Lib-L according to manufacturer’s protocol. A more detailed protocol for polymerase chain reaction is described in Supplementary Methods .
Taxonomic assignment and bioinformatics
Taxonomic classifications
Sequences were treated for quality control prior to taxonomic classification ( Supplementary Methods ). QIIME was utilized to produce operational taxonomic units (OTU) clusters and classifications. All the processed 16S rRNA sequences were clustered into OTUs based on their sequence similarity using UCLUST algorithm, setting the sequence similarity threshold to 0.97. For each OTU, a representative sequence was selected for downstream analysis. Taxonomy was assigned to each representative sequence using the ribosomal database project classifier method v2.2. The taxonomic assignments that resulted in no more than 2 sequence reads assigned to a genus, and with a mean ribosomal database project score of <0.9, were considered to be low-quality and were excluded from the community structure analysis. This filtering eliminated a total of 94 low-quality genus assignments for pilot 1, 164 for pilot 2, and 147 for pilot 3. All sequence data were deposited in http://www.ncbi.nlm.nih.gov/sra/ under the SRP078557 accession.
Alpha diversity
QIIME was used to calculate alpha diversity and rarefaction curves before filtering. Shannon and Simpson methods were employed to analyze the biodiversity within a group of samples. Plots were generated by QIIME, with the number of sequences on the x-axis and the corresponding alpha diversity index on the y-axis. In rarefaction curves, if the lines for some categories do not extend, that means that at least one of the samples in that category does not reach that number of sequences. The shape on the horizontal axis plot serves as an indicator of richness: greater y-axis values indicate more species richness and lower values indicate the opposite.
Community clustering analysis
The clustering of communities was done using Bray-Curtis distance and hierarchical clustering with R vegan package.
Principal component analysis of microbial communities
Principal component analysis (PCA) was generated using the prcomp routine in the R package on a data set consisting of the percentage abundances of taxa in each community. The 2 principal components explained 88% of the variance. Principal coordinates analysis (PCoA) plots were generated using Bray-Curtis distances.
Statistical analysis
Statistical analysis on bacterial taxonomic identification was performed using R version 3.1.1. Supervised machine learning models were performed in R ( http://www.R-project.org ) using different packages from CRAN, ROCR for receiver operating characteristic curves, rpart for classification and regression tree (CART), and generalized linear model for logistic regression. A comparison of quantitative variables was done using Student t test or analysis of variance test for independent samples (depending on whether ≥2 groups were compared). The Adonis function in the R package vegan was used to conduct nonparametric multivariate analysis of variance. For comparing categorical data, χ 2 test was performed. P < .05 was considered statistically significant.
Materials and Methods
Study design
Three separate prospective pilot studies were performed. First, to analyze the existence of a differential endometrial microbiota, paired samples of endometrial fluid (EF) and vaginal aspirates (VA) were obtained simultaneously from 13 fertile women in their prereceptive (two days after the luteinizing hormone surge, known as LH+2) and receptive (seven days after the uteinizing hormone surge, also known as LH+7) phases in the same menstrual natural cycle (total samples analyzed n = 52). To investigate the hormonal regulation of the endometrial microbiota, EF samples (n = 44) were collected at LH+2 and LH+7 within the same natural cycle from 22 fertile women. Finally, the reproductive impact of an altered endometrial microbiota in EF was assessed by implantation, miscarriage, ongoing pregnancy, and live birth rates in infertile subjects undergoing IVF (n = 35) in whom a receptive endometrium was diagnosed using the endometrial receptivity array (ERA). EF samples were obtained in the cycle before embryo transfer. Approval for this study was obtained from the IVI Valencia Ethical Committee (project: 1404-FIVI-015-CS), and subjects provided written informed consent.
Subjects
For comparison between vaginal and endometrial bacterial communities and the study of hormonal regulation of the endometrial microbiota, samples were obtained within the natural cycles of women from the ovum donation program at IVI Valencia, Spain. The functional impact of the endometrial microbiota on reproductive outcome was explored in infertile subjects undergoing IVF treatment in whom a receptive endometrium was diagnosed by ERA (Igenomix SL, Valencia, Spain). A more detailed description is provided in Supplementary Methods .
EF aspiration
EF was obtained in all subjects as previously described.
VA collection
In the first pilot study, 20–80 μL of VA were collected from the posterior vagina before EF aspiration using a sterile catheter under direct vision.
Endometrial receptivity diagnosis
Endometrial receptivity was diagnosed using the ERA as previously described. A more detailed protocol is provided in Supplementary Methods .
Genomic DNA isolation from EF and VA samples
Isolation of DNA from frozen EF or VA was performed following the MagNa Pure compact nucleic acid isolation kit I (Roche, Madison, WI) protocol with modifications. A more detailed protocol is provided in Supplementary Methods .
Polymerase chain reaction and 16S rRNA sequencing
For sequencing and barcoding, the V3-V5 region of the 16S rRNA gene was amplified using key-tagged eubacterial primers, as previously described. Unidirectional pyrosequencing was carried out on a 454 Life Sciences GS FLX+ instrument (Roche) following the Roche Amplicon Lib-L according to manufacturer’s protocol. A more detailed protocol for polymerase chain reaction is described in Supplementary Methods .
Taxonomic assignment and bioinformatics
Taxonomic classifications
Sequences were treated for quality control prior to taxonomic classification ( Supplementary Methods ). QIIME was utilized to produce operational taxonomic units (OTU) clusters and classifications. All the processed 16S rRNA sequences were clustered into OTUs based on their sequence similarity using UCLUST algorithm, setting the sequence similarity threshold to 0.97. For each OTU, a representative sequence was selected for downstream analysis. Taxonomy was assigned to each representative sequence using the ribosomal database project classifier method v2.2. The taxonomic assignments that resulted in no more than 2 sequence reads assigned to a genus, and with a mean ribosomal database project score of <0.9, were considered to be low-quality and were excluded from the community structure analysis. This filtering eliminated a total of 94 low-quality genus assignments for pilot 1, 164 for pilot 2, and 147 for pilot 3. All sequence data were deposited in http://www.ncbi.nlm.nih.gov/sra/ under the SRP078557 accession.
Alpha diversity
QIIME was used to calculate alpha diversity and rarefaction curves before filtering. Shannon and Simpson methods were employed to analyze the biodiversity within a group of samples. Plots were generated by QIIME, with the number of sequences on the x-axis and the corresponding alpha diversity index on the y-axis. In rarefaction curves, if the lines for some categories do not extend, that means that at least one of the samples in that category does not reach that number of sequences. The shape on the horizontal axis plot serves as an indicator of richness: greater y-axis values indicate more species richness and lower values indicate the opposite.
Community clustering analysis
The clustering of communities was done using Bray-Curtis distance and hierarchical clustering with R vegan package.
Principal component analysis of microbial communities
Principal component analysis (PCA) was generated using the prcomp routine in the R package on a data set consisting of the percentage abundances of taxa in each community. The 2 principal components explained 88% of the variance. Principal coordinates analysis (PCoA) plots were generated using Bray-Curtis distances.
Statistical analysis
Statistical analysis on bacterial taxonomic identification was performed using R version 3.1.1. Supervised machine learning models were performed in R ( http://www.R-project.org ) using different packages from CRAN, ROCR for receiver operating characteristic curves, rpart for classification and regression tree (CART), and generalized linear model for logistic regression. A comparison of quantitative variables was done using Student t test or analysis of variance test for independent samples (depending on whether ≥2 groups were compared). The Adonis function in the R package vegan was used to conduct nonparametric multivariate analysis of variance. For comparing categorical data, χ 2 test was performed. P < .05 was considered statistically significant.
Results
Endometrial and vaginal microbiota differ in some asymptomatic subjects
To assess any potential experimental bias due to the primer set used in our study, the microbial communities identified in VA using the V3–V5 hypervariable regions of the 16S rRNA gene ( Figure 1 , right column) were compared to the bacterial taxa previously reported in vaginal samples by other groups using the V1-V2 hypervariable regions. The results demonstrate the identification of the same bacterial OTUs in vaginal samples independent of the set of primers used for sequencing (OTU list is detailed in Table S1 ). Then, to test the existence of a differential endometrial microbiota, paired samples of EF (n = 26) and VA (n = 26) from 13 fertile subjects were obtained and their bacterial communities investigated by pyrosequencing of the variable regions V3-V5 of the bacterial 16S rRNA gene. Different OTUs were identified between endometrial and vaginal samples ( Figures 1 , 2 , and 3 , A , and Table S1 ). From them, 9 samples were colonized only by Lactobacillus spp., while the rest of the samples were colonized by combinations of different OTUs in addition to Lactobacillus . Only 2 of 26 pairs of samples showed the presence of the same bacterial OTUs in endometrium and vagina ( Table S1 ). In the remaining 24 pairs of samples, small differences between endometrial and vaginal microbiota were found with OTUs present in endometrium but not in the vagina, and vice versa, although some of those differences were nearly imperceptible and only contributed small percentages ( Figure 4 ). Interestingly, in 6 paired samples the bacterial communities in the endometrium and vagina were completely different, with a high proportion of potential pathogens belonging to Atopobium , Clostridium , Gardnerella , Megasphaera , Parvimonas , Prevotella , Sphingomonas , or Sneathia genera found in the endometrium but not in the vagina (n = 5: subject 1 at LH+2, subject 4 at LH+2, subject 9 at LH+7, subject 10 at LH+7, and subject 13 at LH+2), or potential bacterial pathogens ( Gardnerella , Clostridium , Sneathia , or Prevotella spp.) in the vagina that were not present in the endometrium (n = 1: subject 11 at LH+2) ( Figure 2 ). Combinations of up to 54 bacterial OTUs formed the microbial endometrial communities, while in vagina we detected up to 20 genera. Lactobacillus was found as the major genus in all EF and VA analyzed, but in different percentages between women. Bacterial genera such as Atopobium , Gardnerella , Prevotella , or Sneathia were also commonly identified in both endometrial and vaginal samples. With the present design we demonstrated that the endometrial microbiota is not a carry-over from the vagina, because some bacterial genera present in the endometrium were not in the vagina of the same subject, and vice versa. Thus, although endometrial and vaginal microbiota were not statistically different in the pool of healthy and fertile women ( P = .733) ( Figure 3 , B), we identified the existence of vaginal and endometrial bacterial communities that, although closely related in most of the subjects tested, are not identical in every woman; these differences were detected in approximately 20% of the women tested.
The endometrial microbiota
After validating EF aspiration as an acceptable method to assess the structure of endometrial bacterial communities, EF was obtained from 22 fertile subjects during the acquisition of receptivity at LH+2 and LH+7 (total samples n = 44). A total of 166 different OTUs were identified. The most represented genus was Lactobacillus (71.7% of identified bacteria); while Gardnerella (12.6%), Bifidobacterium (3.7%), Streptococcus (3.2%), and Prevotella (0.866%) were the other most common genera. The bacterial communities found in EF samples from fertile subjects were clustered according to the bacterial OTUs identified and their abundances. The resulting heatmap showed 2 sets of samples classifying depending on the percentage of Lactobacillus OTUs identified ( Figure 5 ). The first set of samples included those with a high abundance of Lactobacillus (>90%) and very low or nonexistent other OTUs. The second set of samples was formed by lower Lactobacillus abundances that coexisted with bacteria represented by other OTUs. According to the criteria used in a recent study in which the vaginal microbiota of pregnant women was analyzed during gestation, the EF samples were classified in terms of the microbiota into 2 different groups: (1) a Lactobacillus dominated (LD)-microbiota for those samples in which >90% of the detected bacteria belonged to Lactobacillus OTUs, and (2) a non-LD (NLD) microbiota when <90% of the OTUs identified in the sample belonged to Lactobacillus OTUs and thus presented >10% of bacterial OTUs including pathogenic or dysbiotic bacteria. This classification is in agreement with previous evidence demonstrating that gonococcal adherence to in vitro cultured endometrial epithelial cells is significantly reduced at a 1:10 ratio (gonococci:lactobacilli). Using this classification, a correspondence between the community state types (CSTs) used by other authors and our classification of LD and NLD microbiota could be established by comparing CSTs 1, 2, 3, and 5 with the LD microbiota, and CST 4 with the NLD microbiota. This classification was used to identify the microbiota status of EF in subjects during the acquisition of endometrial receptivity, and in IVF subjects in the next pilot studies. Thus, of the 44 endometrial microbiota analyzed from fertile subjects, 28 were assigned to the LD group, and the remaining 16 to the NLD group. When the endometrial microbiota of IVF subjects’ samples were classified, 18 were assigned to the LD group and 20 to the NLD group; the remaining 3 did not produce sequencing data and were excluded from the analysis.
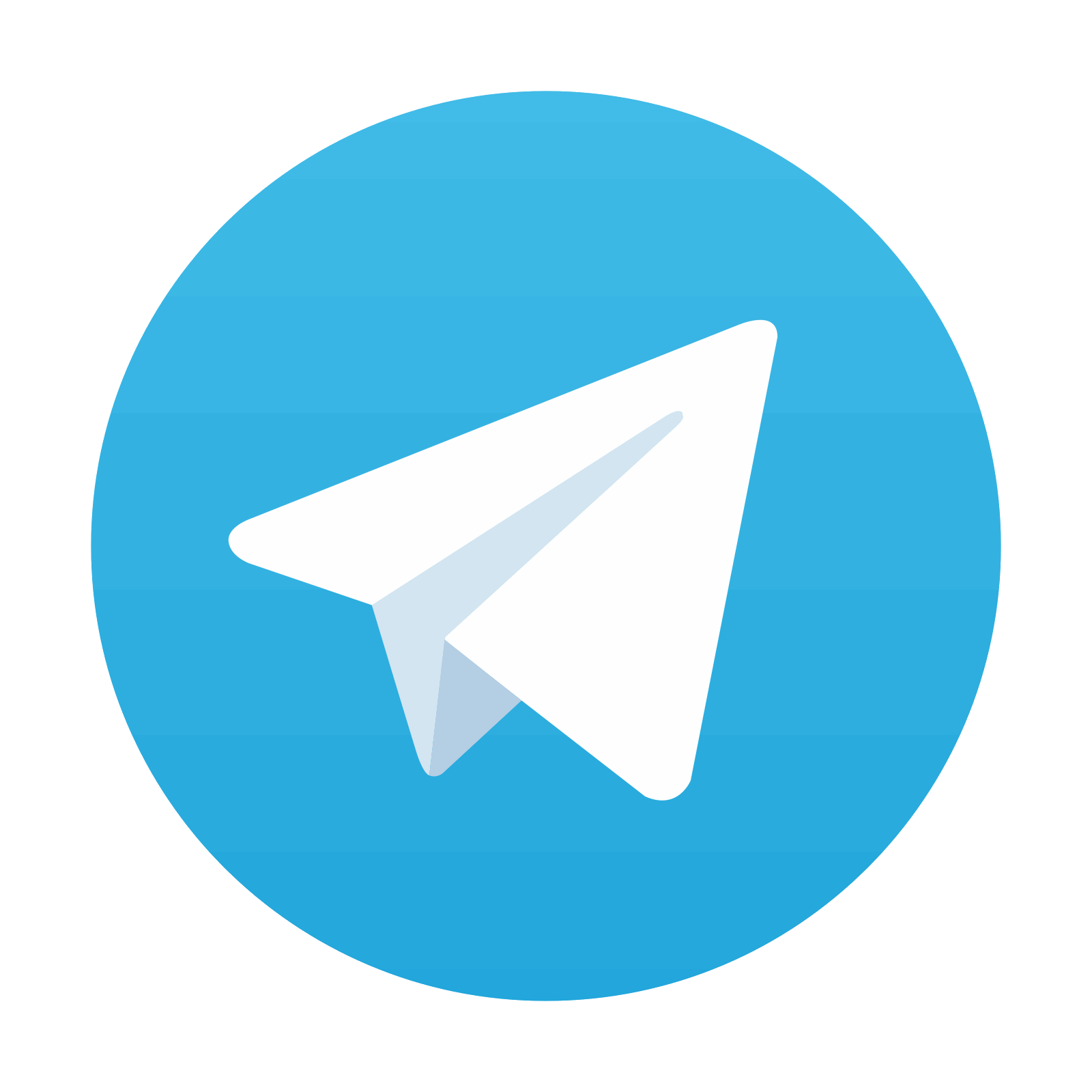
Stay updated, free articles. Join our Telegram channel

Full access? Get Clinical Tree
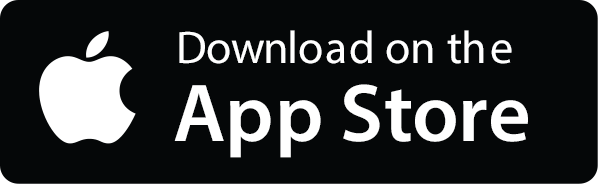
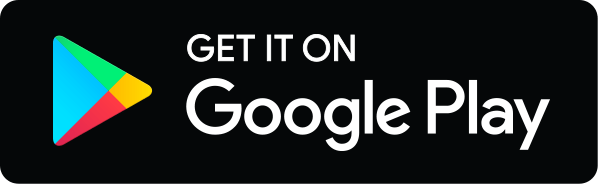
