INTRODUCTION
Reproductive endocrinology is the study of hormones and neuroendocrine factors that are produced by and/or affect reproductive tissues. These tissues include the hypothalamus, anterior pituitary gland, ovary, endometrium, and placenta. A hormone is classically described as a cell product that is secreted into the peripheral circulation and that exerts its effects in distant target tissues (Fig. 15-1). This is termed endocrine secretion. Additional forms of cell-to-cell communication exist in reproductive physiology. Paracrine communication, common within the ovary, refers to chemical signaling between neighboring cells. Autocrine communication occurs when a cell releases substances that influence its own function. Production of a substance within a cell that affects that cell before secretion is termed an intracrine effect.
FIGURE 15-1
Different types of hormone communication. Endocrine: hormones travel through the circulation to reach their target cells. Paracrine: hormones diffuse through the extracellular space to reach their target cells, which are neighboring cells. Autocrine: hormones feed back on the cell of origin, without entering the circulation.
A neurotransmitter, in classic neural pathways, crosses a small extracellular space called a synaptic junction and binds to dendrites of a second neuron (Fig. 15-2). Alternatively, these factors are secreted into the vascular system and are transported to other tissues where they exert their effects in a process termed neuroendocrine secretion or neuroendocrine signaling. One example is gonadotropin-releasing hormone (GnRH) secretion into the portal vasculature with effects on the gonadotropes within the anterior pituitary gland.
FIGURE 15-2
Types of neurotransmitter secretion. A. Classic neurotransmitter release and binding. Transmission of an action potential down a neural axon leads to release of neurotransmitters, which travel across a synaptic cleft to reach their target cell. B. Neurohormonal secretion. An action potential leads to release of neurotransmitters. In this instance, neurotransmitters enter into and travel through the circulation to reach their target organ.
Normal reproductive function requires precise quantitative and temporal regulation of the hypothalamic-pituitary-ovarian axis (Fig. 15-3). Within the hypothalamus, specific centers or nuclei release GnRH in pulses. This decapeptide binds to surface receptors on the gonadotrope subpopulation of the anterior pituitary gland. In response, gonadotropes secrete glycoprotein gonadotropins, luteinizing hormone (LH) and follicle-stimulating hormone (FSH), into the peripheral circulation. Within the ovary, LH and FSH bind to the theca and granulosa cells to stimulate folliculogenesis and ovarian production of steroid hormones (estrogens, progesterone, and androgens), gonadal peptides (activin, inhibin, and follistatin), and growth factors. Among other functions, these ovarian-derived factors feed back to the hypothalamus and pituitary gland to inhibit or, at the midcycle surge, to augment GnRH and gonadotropin secretion. The ovarian steroids are also critical for preparing the endometrium for placental implantation if pregnancy ensues.
FIGURE 15-3
Positive and negative feedback loops seen with the hypothalamic-pituitary-ovarian axis. Pulsatile release of gonadotropin-releasing hormone (GnRH) leads to release of luteinizing hormone (LH) and follicle-stimulating hormone (FSH) from the anterior pituitary. Effects of LH and FSH result in follicle maturation, ovulation, and production of the sex steroid hormones (estrogen, progesterone, and testosterone). Rising serum levels of these hormones exert negative feedback inhibition on GnRH and gonadotropin release. Sex-steroid hormones vary in their effects on the endometrium and myometrium as discussed in the text. Inhibin, produced in the ovary, has a negative effect on gonadotropin release.
HORMONE BIOSYNTHESIS AND MECHANISM OF ACTION
Hormones can be broadly classified as either steroids or peptides, each with their own mode of biosynthesis and mechanism of action. The receptors for these hormones can be divided into two groups: (1) those present on the cell surface, which in general interact with hormones that are water soluble, namely peptides, and (2) those that are primarily intracellular and interact with lipophilic hormones such as steroids. Hormones are normally present in serum and tissues in very low concentrations. Therefore, receptors must have both high affinity and high specificity for their ligand to produce the correct biologic response.
The gonadotropins LH and FSH are biosynthesized and secreted by the gonadotrope subpopulation of the anterior pituitary gland. These hormones play a critical role in stimulating ovarian steroidogenesis, follicular development, and ovulation. The closely related peptide human chorionic gonadotropin (hCG) is produced by placental trophoblast and is important for maintenance of pregnancy.
LH, FSH, and hCG are heterodimers consisting of a common glycoprotein α-subunit linked to a unique β-subunit, which provides functional specificity. Although glycoprotein α- and β-subunits can be found in their unassociated form in the circulation, these “free” subunits are not known to have biologic activity. Nevertheless, their measurement may be useful in screening tests for conditions such as pituitary adenomas and pregnancy.
The LH and hCG β-subunits are encoded by two separate genes within a gene grouping called the LH/CG cluster. The amino acid sequence of the human LH and CG β-subunits demonstrates approximately 80-percent similarity. However, the hCG β-subunit contains an additional 24-amino-acid extension on the carboxy terminus. The presence of these additional amino acids has allowed the development of highly specific assays that can distinguish LH from hCG.
In pituitary thyrotropes, the shared glycoprotein α-subunit also interacts with the thyroid-stimulating hormone β-subunit to form thyroid-stimulating hormone (TSH). This similarity between TSH and hCG can have clinical sequelae. For example, molar pregnancies frequently produce very high levels of hCG, which can bind to TSH receptors, resulting in hyperthyroidism (Walkington, 2011).
This glycosylated peptide hormone is produced by the placental syncytiotrophoblast. With this molecule, the degree and type of glycosylated moieties attached to the peptide frame is variable and may indicate pregnancy stage, placental function, or pathology (Fournier, 2015). One example is the hyperglycosylated hCG that is found more commonly in gestational trophoblastic neoplasia.
hCG can be detected in serum as early as 7 to 9 days after the LH surge. In early pregnancy, hCG levels increase rapidly, doubling approximately every 2 days. Levels of this peptide hormone peak at approximately 100,000 mIU/mL during the first trimester of pregnancy. This is followed by a relatively sharp decline in the early second-trimester concentrations and then maintenance at lower levels throughout the remainder of pregnancy.
hCG binds to LH/CG receptors on corpus luteum cells and stimulates steroidogenesis in the ovary. To maintain endometrial integrity and uterine quiescence, hCG levels are critical. Namely, hCG supports corpus luteum steroid production during early pregnancy before the placenta attains adequate steroidogenic capability. The transition in production of estrogens and progesterone from the ovary to the placenta is often called the “luteal-placental shift.” In addition to effects on ovarian function, hCG exerts autocrine/paracrine effects in the placenta, promoting syncytiotrophoblast formation, trophoblast invasion, and angiogenesis.
As the placenta is the primary source for hCG production, measurement of plasma hCG levels has proved to be an effective screening tool for pregnancies with altered placental mass or function. Relatively elevated levels of hCG are observed in multifetal gestations and fetuses with Down syndrome. Lower hCG levels are observed in cases of poor placentation including ectopic pregnancy or spontaneous miscarriage. Serial hCG measurements can be very helpful to monitor these latter conditions as the doubling time is relatively reliable. Markedly abnormal elevations in hCG levels are most often observed in the presence of gestational trophoblastic disease, discussed in Chapter 37.
Human CG is also secreted by nontrophoblastic neoplasias and can serve as a useful tumor marker. Ectopic (nonplacental) production of hCG, either the intact dimer or the β-subunit, is frequently associated with germ cell tumors and has been reported in various tumors arising from the mucosal epithelium of the cervix, bladder, lung, gastrointestinal tract, and nasopharynx. It has been postulated that hCG inhibits apoptosis in these tumors, thereby allowing rapid growth.
In addition to secretion by placental syncytiotrophoblast, hCG is produced by nonneoplastic cell types and presumably serves other functions (Cole, 2010). For example, cytotrophoblasts secrete a hyperglycosylated variant of hCG that may prove to be a sensitive marker of early pregnancy (Chuan, 2014). The pituitary gonadotropes also make small amounts of hCG. These concentrations rise in postmenopausal women and may be a rare cause of erroneously positive hCG testing in this age group (Cole, 2008).
Sex steroids are divided into three groups based on the number of carbon atoms that they contain. Each carbon in this structure is assigned a number identifier, and each ring is assigned a letter (Fig. 15-4). The 21-carbon series includes progestins, glucocorticoids, and mineralocorticoids. Androgens contain 19 carbons, whereas estrogens have 18.
Steroids are given scientific names according to a generally accepted convention in which functional groups below the plane of the molecule are preceded by the α symbol and those above the plane of the molecule are indicated by a β symbol. A Δ symbol indicates a double bond. Those steroids with a double bond between carbon atoms 5 and 6 are called Δ5 steroids and include pregnenolone, 17-hydroxypregnenolone, and dehydroepiandrosterone. Those with a double bond between carbons 4 and 5 are termed Δ4 steroids and include progesterone, 17-hydroxyprogesterone, androstenedione, testosterone, mineralocorticoids, and glucocorticoids.
Sex steroid hormones are synthesized in the gonads, adrenal gland, and placenta. Cholesterol is the primary building block. All steroid-producing tissues, except the placenta, are capable of synthesizing cholesterol from the two-carbon precursor, acetate. Steroid hormone production, which involves at least 17 enzymes, primarily occurs in the mitochondria and the abundant smooth endoplasmic reticulum found in steroidogenic cells (Mason, 2002). These enzymes are members of the cytochrome P450 superfamily. As such, genes that encode these enzymes begin with CYP.
Steroidogenic enzymes catalyze four basic modifications of the steroid structure: (1) side-chain cleavage (desmolase reaction), (2) conversion of hydroxyl groups to ketones (dehydrogenase reactions), (3) addition of a hydroxyl group (hydroxylation reaction), and (4) removal or addition of hydrogen to create or reduce a double bond (Table 15-1). The steroid biosynthesis pathway is shown in simplified form in Figure 15-5. This pathway is identical in all steroidogenic tissues, but the distribution of products synthesized by each tissue is determined by the presence of requisite enzymes. For example, the ovary is deficient in 21-hydroxylase and 11β-hydroxylase and thus is unable to produce corticosteroids. Of note, many steroidogenic enzymes exist as multiple isoforms, each with different precursor preferences and directional activities. As a result, specific steroids may be produced via multiple pathways in addition to the classic pathway shown in Figure 15-5 (Auchus, 2009).
FIGURE 15-5
Steps in the steroidogenesis pathway. Enzymes are found within the blue ovals. The C-18, C-19, or C-21 designation beneath the sex steroid reflects the number of carbon atoms it contains. Colored boxing groups these pathway products. 3β-HSD = 3β-hydroxysteroid dehydrogenase; 11β-OHase = 11β-hydroxylase; 17αOHase = 17α-hydroxylase; 17β-HSD = 17β-hydroxysteroid dehydrogenase; 21OHase = 21-hydroxylase; DHEA = dehydroepiandrosterone; SCC = side-chain cleavage enzyme.
Estrogens are synthesized by aromatization of C19 androgens by aromatase. The aromatase enzyme is a cytochrome P450 enzyme encoded by the gene CYP19. In addition to the ovary, aromatase is expressed in significant levels in adipose tissue, skin, and brain (Boon, 2010). Importantly, sufficient estrogen can be derived from peripheral aromatization to produce endometrial bleeding in postmenopausal women, especially those who are overweight or obese.
Circulating estrogens in the reproductive-aged female include estrone (E1), estradiol (E2), and estriol (E3). Estradiol is the primary estrogen produced by the ovary during reproductive years. Levels are derived both from direct synthesis in the granulosa cells of developing follicles and through conversion of the less potent estrone. Estrone, the primary estrogen during menopause, is secreted primarily by the ovary. Estriol, the predominant estrogen during pregnancy, is primarily secreted from the placenta. However, both estrone and estriol can be converted from androstenedione in the periphery.
The ovary also produces androgens in response to LH stimulation of theca cell function. The primary products are the relatively weak androgens androstenedione and dehydroepiandrosterone (DHEA), although smaller amounts of testosterone are also secreted. Although the adrenal cortex primarily produces mineralocorticoids and glucocorticoids, it also contributes to approximately one half of the daily production of androstenedione and DHEA and essentially all of the sulfated form of DHEA (DHEAS). In women, 25 percent of circulating testosterone is secreted by the ovary, 25 percent is secreted by the adrenal gland, and the remaining 50 percent is produced by peripheral conversion of androstenedione to testosterone (Fig. 15-6) (Silva, 1987).
The adult adrenal gland is composed of three zones. Each of these zones expresses a different complement of steroidogenic enzymes and as a result synthesizes different products. The zona glomerulosa lacks 17α-hydroxylase activity but contains large amounts of aldosterone synthase (P450aldo) and therefore produces mineralocorticoids. The zona fasciculata and zona reticularis, both of which express the 17α-hydroxylase gene, synthesize glucocorticoids and androgens, respectively.
Within androgen synthesis, the 5α-reductase enzyme converts testosterone to dihydrotestosterone (DHT), a more potent androgen. DHT promotes transformation of vellus hair to terminal hair. Thus, medications that antagonize 5α-reductase are often effective in the treatment of hirsutism (Stout, 2010). This enzyme exists in two forms, each encoded by a separate gene. The type 1 enzyme is found in the skin, brain, liver, and kidneys. In contrast, the type 2 enzyme is predominantly expressed in male genitalia (Russell, 1994).
Most steroids in the peripheral circulation are bound to carrier proteins. These proteins may be either specific proteins, such as sex hormone-binding globulin (SHBG), thyroid-binding globulin, or corticosteroid-binding globulin, or nonspecific proteins such as albumin. Only 1 to 2 percent of androgens and estrogens are unbound or free.
Only the unbound steroid fraction is believed to be biologically active, although albumin’s low affinity for sex steroids likely allows steroids bound to this protein to exert some effect. The amount of free hormone is in equilibrium with the amount of bound. In other words, the amount of free, biologically active hormone is inversely related to the amount of bound hormone, and the amount of bound hormone is a direct reflection of the levels of carrier protein. As a result, small changes in carrier protein expression can produce substantial alterations in steroid effect.
SHBG circulates as a homodimer that binds a single steroid molecule. This binding protein is primarily synthesized in the liver, although it has also been detected in the brain, placenta, endometrium, and testes. SHBG levels are increased by hyperthyroidism, pregnancy, and estrogen administration. In contrast, androgens, progestins, growth hormone (GH), insulin, and corticoids decrease SHBG levels. An increase in weight, particularly central body fat, can significantly blunt SHBG expression. In turn, this decreases bound hormone levels and increases active hormone levels (Hammond, 2012).
Clinically, unbound hormone can be technically difficult to measure, and results should be interpreted with caution. Free testosterone levels are the most commonly ordered free steroid hormone tests, but the most accurate assays are performed by only a few commercial laboratories (Rosner, 2007). The more available calculated free levels are relatively inaccurate. Moreover, free testosterone measurement is rarely necessary for clinical diagnosis in the female and is unlikely to add more information than the total testosterone level. For example, measurement of testosterone levels in patients with presumed polycystic ovarian syndrome (PCOS) is important to exclude an androgen-producing tumor, which will produce markedly elevated total testosterone levels. In contrast, normal or high-normal levels of total testosterone are consistent with the diagnosis of PCOS. Because testosterone lowers SHBG levels, patients with normal total testosterone levels, but with clinical evidence of hyperandrogenism (hirsutism and/or acne), invariably have either increased free testosterone levels or increased sensitivity of the hair follicle and sebaceous glands.
Ultimately, steroids are metabolized mainly in the liver and to a lesser extent in the kidney and intestinal mucosa. Hydroxylation of estradiol results in production of estrone or catechol estrogens. These estrogens are then conjugated to glucuronides or sulfates to form water-soluble compounds for excretion in the urine. Accordingly, administration of certain pharmacologic steroid hormones may be contraindicated in those with active liver or renal disease.
RECEPTOR STRUCTURE AND FUNCTION
Steroid hormones and peptide factors differ in their specific receptor-mediated actions, yet both eventually lead to DNA transcription and protein production in the target cell.
These are cell-membrane-associated receptors that bind peptide factors. These receptors consist of a hydrophilic extracellular domain, an intracellular domain, and a hydrophobic transmembrane domain that spans the cell membrane seven times. When bound to hormone, these receptors undergo a conformational change, activate intracellular signaling pathways, and, through a series of phosphorylation events, ultimately modulate transcription of multiple genes within the target cell.
The gonadotropin-releasing hormone receptor (GnRH-R) is a G-protein-coupled receptor that has been identified in the ovary, testes, hypothalamus, prostate, breast, and placenta (Yu, 2011). Although data are still preliminary, GnRH and its receptor may form an autocrine/paracrine regulatory network in reproductive tissues including the ovaries and placenta in addition to the classic neuroendocrine hypothalamic-pituitary system (Kim, 2007; Lee, 2010).
Both LH and hCG bind to the same G-protein-coupled receptor known as the LH/CG receptor. Relative to LH, hCG has a slightly higher affinity for the receptor and has a longer half-life. In contrast, FSH binds to a unique G-protein-coupled receptor located on the granulosa cell membrane.
Within the ovary, the LH/CG receptor is expressed on thecal cells, interstitial cells, and luteal cells. In the granulosa cells of preantral follicles, LH/CG receptor mRNA is nearly undetectable. However, in the differentiated granulosa cells found during follicular maturation, high levels of this receptor are observed. In addition to the ovary, LH/CG and FSH receptors have also been identified in endometrium, myometrium, and placenta (Stilley, 2014; Ziecik, 2007. The function of the receptor in these extraovarian tissues is poorly understood.
The nuclear receptor superfamily consists of three receptor groups: (1) those that bind steroidal ligands, (2) those that have affinity for nonsteroidal ligands such as thyroid hormone, and (3) orphan receptors. By definition, orphan nuclear receptors do not have an identified ligand. These are believed to be constitutively active, that is, they exhibit basal or intrinsic activity. Despite their structural similarities, estrogens, progestins, androgens, mineralocorticoids, and glucocorticoids all interact with unique members of the nuclear hormone receptor family.
Free steroids diffuse into cells and combine with specific receptors (Fig. 15-7A). Members of this receptor superfamily exhibit a modular structure of distinct domains (Fig. 15-8). Each region contributes distinct activities required for full receptor function. In general, nuclear receptors have two regions that are critical for gene activation, termed activation function 1 (AF1) and activation function 2 (AF2). AF1 is located in the A/B domain and is usually ligand independent. AF2 is in the ligand-binding domain (E) and is often hormone-dependent. The highly conserved DNA-binding region (C) inserts into the DNA helix. Subsequently, steroid receptors enhance or repress gene transcription through interactions with specific DNA sequences, called hormone response elements, in the promoter region of target genes (Klinge, 2001).
FIGURE 15-7
Estrogen-receptor ligand-dependent and ligand-independent activation. A. Classically, the estrogen receptor can be activated by estrogen. Unbound hormone is free to bind with empty steroid receptors found either in the cytoplasm or, more commonly, in the cell’s nucleus. Hormone-bound receptors then bind to specific DNA promoter sequences. This binding typically leads to DNA transcription and eventually to specific protein synthesis. B. The estrogen receptor can also be activated independently of estrogen. Growth factors can increase the activity of protein kinases that phosphorylate different sites on the receptor molecule. This unbound, yet activated, receptor will then exert transcriptional effects. C. Nonnuclear estrogen-signaling pathways can also produce effects. Cell-membrane estrogen receptors are located in invaginations called caveolae. Estrogen binding to these estrogen receptors is linked to the mitogen-activated protein kinase pathway and results in a rapid, nonnuclear effect. (Reproduced with permission from Gruber CJ, Tschugguel W, Schneeberger C, et al: Production and actions of estrogens. N Engl J Med 2002 Jan 31;346(5):340–352.)
As a a general rule, nuclear hormone receptors are localized to the cytoplasm. Following ligand binding, they then are translocated to the nucleus to exert their effects.
Two isoforms of estrogen receptors, ERα and ERβ, are encoded by separate genes (Kuiper, 1997). These receptors are differentially expressed in tissues and appear to serve distinct functions. For example, both ERα and ERβ are required for normal ovarian function. However, mice lacking ERα are anovulatory and accumulate cystic follicles, whereas ovaries missing ERβ are normal histologically despite impaired ovulation (Couse, 2000).
The progesterone receptor also exists in multiple isoforms. Encoded from a single gene, PRA and PRB are identical except for an additional 164 amino acids at the amino terminus (Conneely, 2002). Similar to estrogen receptors, the PR isoforms are not interchangeable. For example, PRA is required for normal ovarian and uterine functions but is expendable in the breast (Lydon, 1996). In contrast to the estrogen and progesterone receptor situation, only one form of the androgen receptor has been identified.
Recent studies have introduced the concept that a subset of steroids, including estrogens and progestins, may alter cell function via nongenomic effects, that is, independent of the classic nuclear hormone receptors (see Fig. 15-7C). These nongenomic effects occur rapidly and may be mediated via cell-surface receptors (Kowalik, 2013; Revelli, 1998). Pharmacologic agents under development specifically target these nongenomic effects to allow more precise therapy for steroid-sensitive disorders.
Many influences alter cellular response to sex steroids and peptide factors. The number of receptors within a cell or on the cell membrane is critical to attain maximum hormonal response. Importantly, the number of receptors on a cell can be modified through gene transcription and receptor protein degradation.
Hormonally induced negative feedback of receptors is termed homologous downregulation or desensitization. Desensitization limits the duration of a hormonal response by decreasing the cell’s sensitivity to a constant, prolonged level of hormone. Within the reproductive system, desensitization is best understood for the GnRH receptor and is used clinically to produce a hypoestrogenic state. Pharmacologic agonists of GnRH, such as leuprolide acetate (Lupron), initially stimulate receptors on pituitary gonadotropes to cause a supraphysiologic release of both LH and FSH. Over a period of hours, agonists downregulate GnRH receptor sensitivity and number, thus preventing further GnRH stimulation. Correspondingly, decreased gonadotropin secretion leads to suppressed estrogen and progesterone levels 1 to 2 weeks after initial GnRH agonist administration.
IMMUNOASSAYS FOR PEPTIDE AND STEROID HORMONES
These tests use antibodies to detect most polypeptide, steroid, and thyroid hormones. They are sensitive and easily automated. Hormone concentration is usually reported as international units per volume rather than mass per volume (Table 15-2). When interpreting immunoassays, several concepts must be understood. These include reference standards, the “hook effect,” normal ranges, and supplementary hormone levels.
Steroid | Subjects | Reference Values |
Androstenedione | Men | 2.8–7.3 nmol/L |
Women | 3.1–12.2 nmol/L | |
Testosterone | Men | 6.9–34.7 nmol/L |
Women | 0.7–2.8 nmol/L | |
Dihydrotestosterone | Men | 1.0–3.10 nmol/L |
Women | 0.07–.086 nmol/L | |
Dehydroepiandrosterone | Men/Women | 5.5–24.3 nmol/L |
Dehydroepiandrosterone sulfonate | Men/Women | 2.5–10.4 μmol/L |
Progesterone | Men | <0.3–1.3 nmol/L |
Women | ||
Follicular | 0.3–3.0 nmol/L | |
Luteal | 19.0–45.0 nmol/L | |
Estradiol | Men | <37–210 pmol/L |
Women | ||
Follicular | <37–360 pmol/L | |
Luteal | 625–2830 pmol/L | |
Midcycle | 699–1250 pmol/L | |
Postmenopausal | <37–140 pmol/L | |
Estrone | Men | 37–250 pmol/L |
Women | ||
Follicular | 110–400 pmol/L | |
Luteal | 310–660 pmol/L | |
Postmenopausal | 22–230 pmol/L | |
Estrone sulfonate | Men | 600–2500 pmol/L |
Women | ||
Follicular | 700–3600 pmol/L | |
Luteal | 1100–7300 pmol/L | |
Postmenopausal | 130–1200 pmol/L |
First, to minimize assay-to-assay variability, a reference material is needed to standardize assays. Reference standards serve as anchors that can provide comparability across time and methods. Such reference preparations are produced by the World Health Organization (WHO) and the National Institutes of Health (NIH). More than 20 assay standards are available to measure LH, FSH, prolactin (PRL), and hCG. Thus, knowing which reference standard is used by a specific assay is essential, as results may differ significantly. Clinically, this can become an issue in patients with possible ectopic pregnancies when serial β-hCG levels are obtained at different health care facilities.
Second, the “hook effect” can alter immunoassay result interpretation. With this effect, significantly elevated hormone levels saturate the assay’s targeting antibody and create a falsely low reading. Moreover, the amount of hormone present in a sample does not necessarily correlate with the biological activity of that hormone. For example, PRL exists in multiple isoforms, many of which are immunologically detectable but not biologically active. Similarly, varying glycosylation patterns of gonadotropins at different times during the reproductive life span are believed to alter their biologic activity.
Another caveat is a result that lies in the “normal range.” For many hormones, a stated normal range is often broad. As such, the hormone level of an individual may double, but remain within the normal range even though the result is actually abnormal for that individual.
Last, the addition of other hormone levels may be necessary to define the significance of a result. In the context of the pituitary gland and its target endocrine glands, it may be adequate to measure the pituitary hormone alone. For example, high levels of circulating gonadotropins are almost invariably due to ovarian failure and loss of negative feedback. This is because pituitary overproduction of functional dimer is rare. Conversely, low gonadotropin levels can be attributed confidently to hypothalamic-pituitary dysfunction. Thus, the measurement of ovarian-derived products such as estrogen may be helpful to confirm the diagnosis but are not critical.
In other clinical scenarios, the measurement of both pituitary and target hormone levels may be indicated. For example, in many laboratories, an abnormal TSH value will lead to “reflex,” that is, automatic testing for thyroid hormone levels. Low levels of both a stimulating-hormone and target hormone indicate an abnormality in either hypothalamic or pituitary function. High levels of a target-gland hormone coupled with low levels of its stimulating pituitary hormone suggest autonomous secretion by the target organ such as occurs in the hyperthyroidism of Graves disease.
These tests may be useful when hypofunction of an endocrine organ is suspected. These tests use an endogenous stimulating hormone to assess the reserve capacity of the tissue of interest. The trophic hormone used may be a hypothalamic releasing factor such as GnRH or thyrotropin-releasing hormone (TRH). Alternatively, a substitute pituitary hormone may be used, such as hCG as a substitute for LH or leuprolide acetate for GnRH. The ability of the target gland to respond is measured by an increase in the appropriate hormone’s plasma level. One example, the leuprolide stimulation test, may be used to evaluate abnormal pubertal development and is described in Chapter 14. Leuprolide substitutes for GnRH because clinical-grade GnRH is often unavailable (Rosenfield, 2013).
These tests may be performed when endocrine hyperfunction is suspected. For example, a dexamethasone suppression test may be given to a patient with suspected hypercortisolism (Cushing disease or syndrome). Described in full in Chapter 17, this test gauges the ability of dexamethasone to inhibit adrenocorticotropic hormone (ACTH) secretion and thus cortisol production by the adrenal. The failure of glucocorticoid treatment to suppress cortisol production would be consistent with primary hyperadrenalism.
THE HYPOTHALAMIC-PITUITARY AXIS
The hypothalamus consists of nuclei located at the base of the brain, just superior to the optic chiasm. Neurons within the hypothalamus form synaptic connections with other neurons throughout the central nervous system (CNS). A subset of the hypothalamic neurons within the arcuate, ventromedial, and paraventricular nuclei project to the median eminence. In the median eminence, a dense network of capillaries arises from the superior hypophyseal arteries. These capillaries drain into portal vessels that traverse the pituitary stalk and then form a capillary network within the anterior pituitary gland (adenohypophysis). The primary direction of this hypophyseal portal system is from hypothalamus to pituitary. However, retrograde flow also exists. This creates an ultrashort feedback loop between the pituitary gland and hypothalamic neurons. The hypothalamus is thus a critical locus for integration of information from the environment, nervous system, and other organ systems.
The anterior pituitary gland consists of endocrine cells and is derived from an invagination of Rathke pouch in the roof of the embryonic oral cavity. In contrast, the posterior pituitary gland (neurohypophysis) is neural tissue and consists of the axon terminals of magnocellular neurons arising in the supraoptic and paraventricular nuclei of the hypothalamus (Fig. 15-9).
FIGURE 15-9
Sagittal section through the hypothalamus and pituitary gland with rostral structures to the left and caudal ones to the right. The hypothalamus is anatomically and functionally linked with the anterior pituitary by the portal system of blood supply. The posterior pituitary contains the axon terminals of neurons arising in the supraoptic (SO) nucleus and paraventricular nucleus (PVN) of the hypothalamus. AHA = anterior hypothalamic area; AN = arcuate nucleus; DMN = dorsomedial nucleus; PHN = posterior hypothalamic nucleus; POA = preoptic area; VMN = ventromedial nucleus. (Reproduced with permission from Cunningham FG, Leveno KJ, Bloom SL, et al: Williams Obstetrics, 23rd ed. New York: McGraw-Hill; 2010.)
The list of known neurotransmitters continues to expand as does our understanding of their anatomic distribution, mode of regulation, and mechanism of action. Neurotransmitters can be classified as: (1) biogenic amines (dopamine, epinephrine, norepinephrine, serotonin, histamine), (2) neuropeptides, (3) acetylcholine, (4) excitatory amino neurotransmitters (glutamate, glycine, aspartic acid), (5) the inhibitory amino acid gamma-aminobutyric acid (GABA), (6) gaseous transmitters (nitric oxide, carbon monoxide), and (7) miscellaneous factors (cytokines, growth factors).
The most significant neurotransmitters in reproductive neuroendocrinology are the three monoamines: dopamine, norepinephrine, and serotonin. Clinically important neuropeptides within the reproductive axis include the endogenous opiates, kisspeptin, neuropeptide Y, galanin, and pituitary adenylate cyclase-activating peptide.
Central opioidergic neurons are important mediators of hypothalamic-pituitary function. Depending on the precursor peptide from which they are derived, these neuropeptides can be categorized into three classes: endorphins, enkephalins, and dynorphins. Of these, endorphins (endogenous morphines) are cleavage products of the proopiomelanocortin POMC gene, which also yields ACTH and α-melanocyte stimulating hormone (α-MSH) (Taylor, 1997). The endorphins serve a wide range of physiologic functions that include regulation of temperature, cardiovascular and respiratory systems, pain perception, mood, and reproduction.
Proopiomelanocortin is produced in highest concentration in the anterior pituitary gland but is also expressed in the brain, sympathetic nervous system, gonads, placenta, gastrointestinal tract, and lungs. The primary peptide synthesized from this pathway depends on the tissue source. For example, the predominant products in the brain are the opiates, whereas pituitary biosynthesis results principally in ACTH production.
Opioids in the brain play a central role in menstrual cyclicity by tonically suppressing the hypothalamic release of GnRH (Funabashi, 1994). Estrogen promotes endorphin secretion, and this is increased further with the addition of progesterone (Cetel, 1985). Thus, endorphin levels increase during the follicular phase, peak during the luteal phase, and drop markedly during menses. This pattern suggests that both opioid tone and progesterone decrease GnRH pulse frequency in the luteal phase, thus stimulating FSH secretion. For reasons that are not fully understood, opioid suppression of GnRH is relieved at the time of ovulation (King, 1984). In addition, functional hypothalamic amenorrhea due to eating disorders, intensive exercise, and stress is correlated with an increase in endogenous opiate concentrations (Chap. 16).
Hypothalamic kisspeptin neurons play a critical role in sexual differentiation, puberty initiation, and adult reproductive function. These neurons are part of the KNDy neuronal system, named for the coexpression of kisspeptin with neurokinin B and dynorphin. This system likely provides an important link between energy homeostasis and reproductive function. This link may stem in part from the action of the adipose-derived factor leptin, which regulates kisspeptin expression (Chehab, 2014).
Kisspeptin neurons send processes to GnRH neurons, allowing direct control of GnRH secretion. Interestingly, one group of kisspeptin neurons may mediate negative steroid feedback, whereas another is responsible for the positive feedback observed before ovulation (Lehman, 2010; Millar, 2014; Skorupskaite, 2014).
Other neurotransmitters, neuropeptide Y (NPY) and galanin, are expressed by neurons located throughout the hypothalamus and project to kisspeptin neurons, to GnRH neurons, and to other areas of the CNS that have roles in reproductive function. NPY and galanin secretion varies in response to changes in energy level as seen in anorexia and obesity. Both of these neuropeptides alter GnRH pulsatility and potentiate GnRH-induced gonadotrope secretion (Lawrence, 2011; Peters, 2009).
Pituitary adenylate cyclase-activating peptide (PACAP) is a hypothalamic peptide secreted into the pituitary portal system. It binds to receptors on anterior pituitary cells and stimulates hormone secretion including gonadotropin secretion, albeit more weakly than GnRH. Gonadotropes themselves also secrete PACAP, suggesting an autocrine/paracrine role for this hormone within the pituitary. PACAP modulates GnRH-receptor expression and, conversely, GnRH alters PACAP-receptor expression on the gonadotrope cell surface. Furthermore, pituitary PACAP gene expression is markedly increased by GnRH (Halvorson, 2014). Thus, these two important neuropeptides are functionally linked at the level of the anterior pituitary.
The anterior pituitary gland contains five hormone-producing cell types and their products. These include: (1) gonadotropes (which produce LH and FSH), (2) lactotropes (PRL), (3) somatotropes (GH), (4) thyrotropes (TSH), and (5) adrenocorticotropes (ACTH). Of these, gonadotropes comprise approximately 10 to 15 percent of all hormonally active cells in the anterior pituitary (Childs, 1983).
With the exception of PRL, which is under tonic inhibition, pituitary hormones are stimulated by hypothalamic neuroendocrine secretion. Both of the gonadotropins, LH and FSH, are regulated by a single releasing peptide, GnRH, which acts on the anterior pituitary’s gonadotrope subpopulation. Most gonadotropes contain secretory granules that contain both LH and FSH, although a significant number of cells are monohormonal, that is, secrete only LH or only FSH.
Of the other pituitary-releasing hormones, corticotropin-releasing hormone stimulates biosynthesis and secretion of ACTH by the pituitary adrenocorticotropes. Thyrotropin-releasing hormone increases thyrotrope secretion of TSH, also known as thyrotropin. Various hypothalamic secretagogues regulate expression of somatotrope-derived growth hormone. Last, PRL expression is primarily under inhibitory regulation by dopamine. As a consequence of these regulatory mechanisms, damage to the pituitary stalk results in hypopituitarism for LH, FSH, GH, ACTH, and TSH, but an associated increase in PRL secretion.
These peptides have characteristics that are important for both their biologic function and clinical use. First, they are small peptides with short half-lives of a few minutes due to their rapid degradation. Second, hypothalamic releasing peptides are released in minute quantities and are highly diluted in the peripheral circulation. Therefore, biologically active concentrations of these factors are locally restricted to the anterior pituitary gland. Clinically, the extremely low concentrations of these hormones render them essentially undetectable in serum. Thus, levels of their corresponding pituitary factors are measured as surrogate markers.
GnRH is a decapeptide with a half-life of less than 10 minutes. Amino acid modifications generate receptor antagonists or agonists with a prolonged half-life (Fig. 15-10) (Padula, 2005). Pulsatile GnRH input is required for activation and maintenance of GnRH receptors. This characteristic is exploited clinically by administering long-acting GnRH agonists to treat steroid-dependent conditions such as endometriosis, leiomyomas, precocious puberty, breast cancer, and prostate cancer. These agonists compete with endogenous pulsatile GnRH at the receptor, depressing gonadotropin secretion and thereby decreasing serum ovarian sex steroid levels.
Humans express two forms of GnRH termed GnRH I and GnRH II (Cheng, 2005). By convention, GnRH I is the classically described hypothalamic GnRH. The GnRH II peptide has been identified in peripheral tissues and differs in receptor activation (Neill, 2002). Further research is needed to determine the overlapping and divergent functions of these two forms.
Many hypothalamic neurons arise within the CNS, but GnRH-containing neurons have a unique embryologic origin. Progenitor GnRH neurons originate in the medial olfactory placode and migrate along the vomeronasal nerve into the hypothalamus (Fig. 16-5). A series of soluble factors regulate GnRH neuronal migration at specific locations along their migratory route. These factors include secreted signaling molecules such as GABA, adhesion molecules, and growth factors (Wierman, 2011). Failure of normal migration may stem from various genetic defects in these signaling molecules and can lead to Kallmann syndrome, which is discussed in Chapter 16, and other forms of hypogonadotropic hypogonadism.
GnRH cell bodies are primarily located within the arcuate nucleus. From these neuronal cell bodies, GnRH is axonally transported along the tuberoinfundibular tract to the median eminence. GnRH is then secreted into the portal system that drains directly to the anterior pituitary gland and stimulates gonadotropin biosynthesis and secretion. The number of GnRH neurons in the adult is strikingly low, with only a few thousand cells dispersed within the arcuate nucleus.
The olfactory origin of GnRH neurons and nasal epithelial cells suggest a link between reproduction and olfactory signals. Compounds released by one individual that affect other members of the same species are known as pheromones. Pheromones obtained from the axillary secretions of women in the late follicular phase accelerate the LH surge and shorten menstrual cycles of women exposed to these chemicals. Secretions from women in the luteal phase have the opposite effects. Thus, pheromones may be one mechanism by which women who are together frequently often exhibit synchronous menstrual cycles (Stern, 1998).
A subset of GnRH neurons sends projections into other areas of the CNS, including the limbic system. These projections are not required for gonadotropin secretion, but they may play a role in modulation of reproductive behavior (Nakai, 1978; Silverman, 1987).
In elegant experiments, Knobil (1974) demonstrated that pulsatile delivery of GnRH to the pituitary gonadotropes is required to achieve sustained gonadotropin secretion. As shown in Figure 15-11, continuous infusion with GnRH rapidly decreases both LH and FSH secretion, an effect that is easily reversed with a return to pulsatile stimulation.
FIGURE 15-11
Graph shows changes in luteinizing hormone (LH) and follicle-stimulating hormone (FSH) levels with variation in gonadotropin-releasing hormone (GnRH) pulsatile release. (Adapted with permission from Knobil E: The neuroendocrine control of the menstrual cycle, Recent Prog Horm Res 1980;36:53–88.)
Compared with the luteal phase, follicular phase GnRH pulsatility is characterized by increased frequency and decreased amplitude. Higher pulse frequency preferentially stimulates LH, whereas lower frequency favors FSH secretion (Thompson, 2014). Therefore, changes in GnRH pulse frequency affect the absolute levels and the ratio of LH to FSH release.
Pulsatile activity is currently believed to be an intrinsic property of GnRH neurons. Other hormones and neurotransmitters provide modulatory effects (Clayton, 1981; Yen, 1985). In animal models, estrogen increases GnRH pulse frequency and therefore leads to an increase in LH levels relative to FSH levels. In contrast, progesterone decreases GnRH pulsatility. The increase in progesterone during the luteal phase may explain the preferential stimulation of FSH observed toward the end of this phase. This rise in FSH is critical for the initiation of follicular recruitment.
In contrast to the other anterior pituitary hormones, PRL release is primarily regulated via inhibition, specifically by dopamine. These dopamine-containing fibers arise chiefly in the hypothalamic arcuate nucleus and project to the median eminence, where dopamine enters the portal vessels (Table 15-3). Prolactin-releasing factors, although less potent, include TRH, vasopressin, vasoactive intestinal peptide (VIP), endogenous opioids, and acetylcholine.
There are five forms of the dopamine receptor divided into two groups, D1 and D2. Cells in the anterior pituitary gland primarily express the D2 subtypes. The medical treatment of prolactinomas has been improved in terms of both effectiveness and patient tolerance by the development of the D2-specific ligands. For example, the dopamine agonist cabergoline is a D2-specific ligand, whereas bromocriptine is nonspecific.
As indicated by its name, thyrotropin-releasing hormone stimulates secretion of thyroid-stimulating hormone from the anterior pituitary gland’s thyrotrope subpopulation. Of note, TRH is also a potent prolactin-releasing factor and results in a clinical link between hypothyroidism and secondary hyperprolactinemia (Messini, 2010).
TSH binds to specific receptors on the plasma cell membrane of thyroid gland cells. This stimulates thyroid hormone biosynthesis. Thyroid hormone exerts negative feedback on TRH- and TSH-releasing cells.
This is the primary hypothalamic factor that stimulates synthesis and secretion of ACTH. Corticotropin-releasing hormone (CRH) is distributed in multiple locations within the hypothalamus and other CNS areas. Release of CRH is stimulated by catecholaminergic input from other brain pathways and inhibited by endogenous opioids.
Corticotropin-releasing hormone binds to CRH receptors in the anterior pituitary to stimulate ACTH biosynthesis and secretion. In turn, ACTH stimulates glucocorticoid production by the adrenal’s zona fasciculata and androgen production by its zona reticularis. CRH secretion is under negative-feedback regulation by circulating cortisol produced in the adrenal gland. In contrast, mineralocorticoid production by the zona glomerulosa is primarily regulated by the renin-angiotensin system. As a result, abnormalities in the CRH–ACTH pathway do not result in electrolyte disturbances.
Central CRH pathways are believed to mediate many stress responses (Kalantaridou, 2004). Clinically, in women with hypothalamic amenorrhea, CRH levels have been found to be elevated. Increased levels of CRH inhibit hypothalamic GnRH secretion by direct action and by augmenting central opioid concentrations (Fig. 16-6). This functional pathway may explain the association between hypercortisolism and menstrual abnormalities.
Growth hormone secretion by pituitary somatotropes is stimulated by hypothalamic growth hormone-releasing hormone (GHRH) and inhibited by somatostatin. GHRH is primarily secreted by the hypothalamus, but small quantities are released by placental and immune cells. In contrast, somatostatin is widely distributed in the CNS and in the placenta, pancreas, and gastrointestinal tract.
As with GnRH, GHRH depends on pulsatile secretion to exert a physiologic effect. Exercise, stress, sleep, and hypoglycemia stimulate GH release, whereas free fatty acids and other factors related to adiposity blunt GH release. Estrogen, testosterone, and thyroid hormone also play a role in increased GH secretion.
GH stimulates skeletal and muscle growth, regulates lipolysis, and promotes the cellular uptake of amino acids. This hormone induces insulin resistance, and thus GH excess may be associated with new-onset diabetes mellitus. Most of the growth effects of GH are mediated via the insulin-like growth factors, IGF-I and IGF-II. These growth factors are produced in high quantities in the liver. Many of the target tissues in which they exert local effects also synthesize IGFs. Within the ovary, IGF-I and IGF-II stimulate granulosa cell proliferation and steroidogenesis during folliculogenesis (Silva, 2009). IGFs also suppress GH secretion through negative feedback mechanisms.
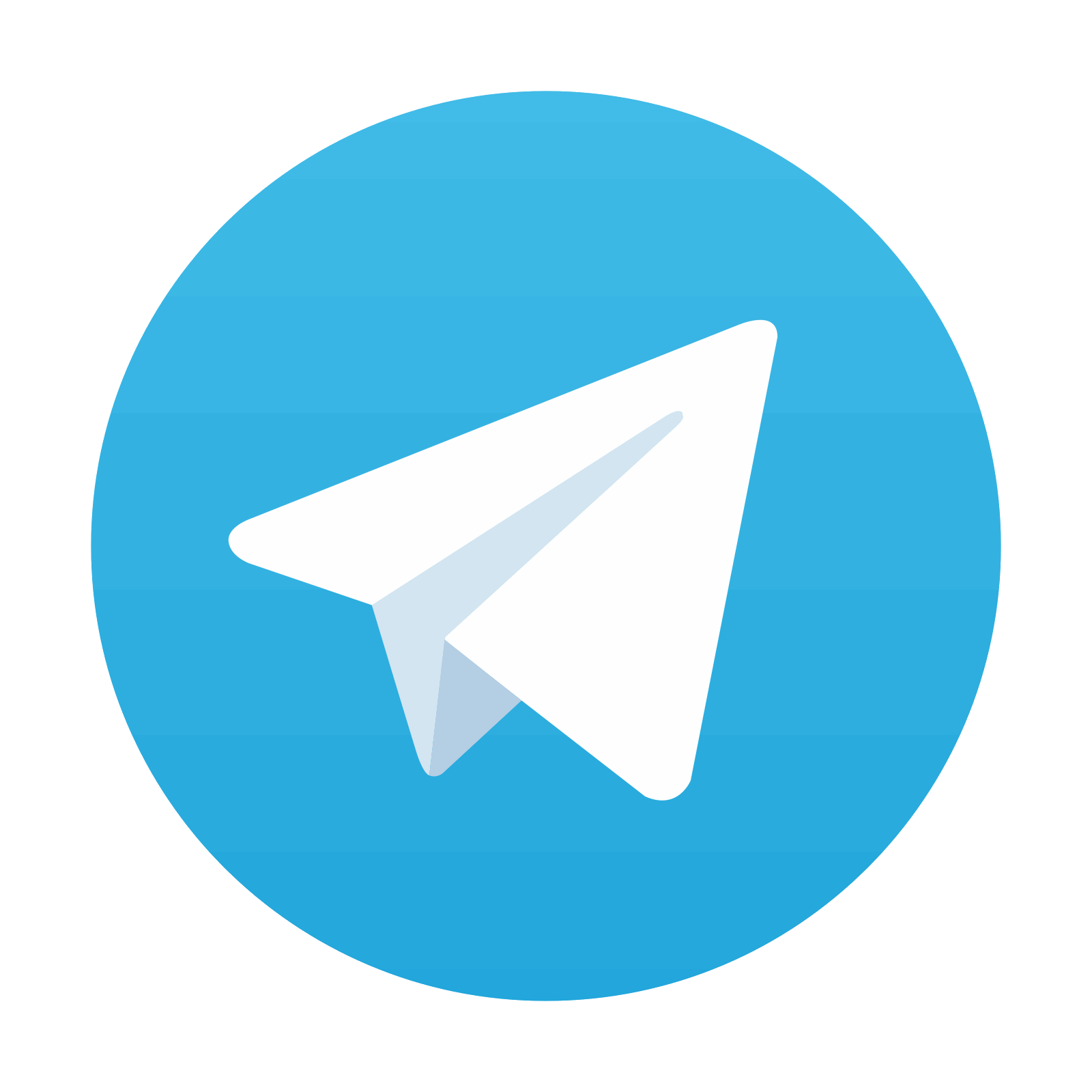
Stay updated, free articles. Join our Telegram channel

Full access? Get Clinical Tree
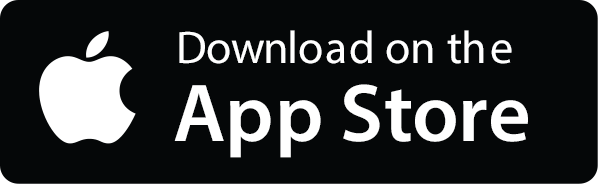
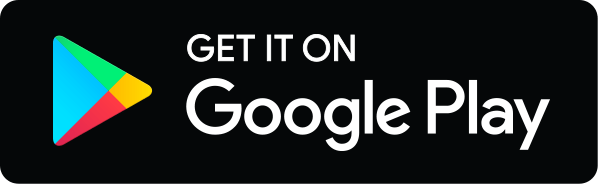