The care of children with heart disease has increasingly become a neonatal specialty. Fetal diagnosis of congenital heart defects (CHDs) is now routine. Reparative cardiovascular surgery is commonplace in the first month of life in select cases and is now undertaken in utero. Defects once considered inoperable, most notably hypoplastic left heart syndrome, can be treated, with greatly improved long-term results. In parallel with these clinical advances, there has been exciting progress in understanding the cellular and molecular basis of normal and abnormal cardiogenesis and the relationship of heart defects to other congenital defects. Nonetheless, the incidence of CHDs has not decreased,49 indicating much more work is required to find ways to prevent CHDs. The expansion in our understanding from combining clinical and basic science findings may lead us to better predict the severity and consequences of congenital heart defects and also lead to strategies for alleviating the consequences to children and adults. It is possible to investigate cardiovascular development at various levels using a variety of disciplines: molecular biology, biochemistry, biomedical engineering, cell and tissue biology, genetics, physiology, and epidemiology. Significant results have emerged when information was shared across disciplines. For example, the hemizygous deletion in the elastin gene has been shown to be responsible for Williams syndrome (or Williams-Beuren syndrome), the autosomal dominant form of supravalvular aortic stenosis.39 Predictions about the clinical manifestations of this disease were elucidated after mutant elastin proteins were found in humans; existence of these mutant proteins was proposed based on knowledge about the protein structure provided by studies at the bench. In the reverse direction, analysis of the mutant human proteins in animal models, tissue culture, and other in vitro studies advanced understanding of the disease and the biochemistry and role of elastin in vascular cell signaling. These signaling pathways may become targets for therapy. The passage of information across these investigative levels has resulted in a remarkably productive synergy. The following description of human heart development, especially the earlier events, is synthesized from information provided by studies of animal models and human embryonic and fetal tissues. A timetable of selected events in human heart development is presented in Table 79-1.33,36,44 Figure 79-1 is a diagram depicting the major transitions in early mammalian heart development.47 TABLE 79-1 Developmental Landmarks in Cardiac Morphogenesis AS, Atrial septum; AV, atrioventricular; IVS, interventricular septum. †Roman numerals refer to Streeter horizons. Data from Moore KL. The developing human: clinically oriented embryology. Philadelphia: Saunders; 1982; Pansky B. Review of medical embryology. New York: Macmillan; 1982; Sissman NJ. Developmental landmarks in cardiac morphogenesis: comparative chronology. Am J Cardiol. 1970;25:141.
Cardiac Embryology
Overview of Normal Heart Development
Week
Days
Somites
Length (mm)*
Stage†
Developmental Events
3
15
VIII
(Primitive streak)
16
17
18
1-3
1.5
Blood islands in chorion, stalk, yolk sac
19
20
1
1.5
Cardiogenic plate
21
4
Tubes (2) connect with blood vessels
4
22
4-7
2
X
Tubes fuse
23
X
Single median tube (first contractions), looping begins
24
13-20
2.5-3
XI
25
26
21-29
3.5
XII
Cardiac loop
27
Single atrium
28
25
4-5
XIII
Bilobed atrium, primary AS begins growth
5
29
6-7
XIV
IVS appears
30
28
Primary AS continues growth (placental circulation begins)
31
7-8
XV
Ridges form inside outflow tract
32
Primary AS begins perforation formation
33
9-10
XVI
34
XVII
Secondary AS begins to grow; primary AS has foramen secundum
35
11-14
AV cushions begin fusion (three-chambered heart)
6
36
Arterial (aortic arch) and venous morphogenesis begins
37
14-16
XVIII
IVS growing
38
39
17-20
XIX
40
Septation of bulbus and ventricle, valve formation
41
21-23
XX
IVS maturation continues, AV canal splits into two
42
7
43
22-24
XXI
Beginning of separation of AV myocardial connection
44
25-27
XXIII
(Fetal stages begin)
45
46
47
48
49
Membranous and muscular IVS is complete, resulting in four-chambered heart
24-40
210-360
Birth and beginning of neonatal circulation
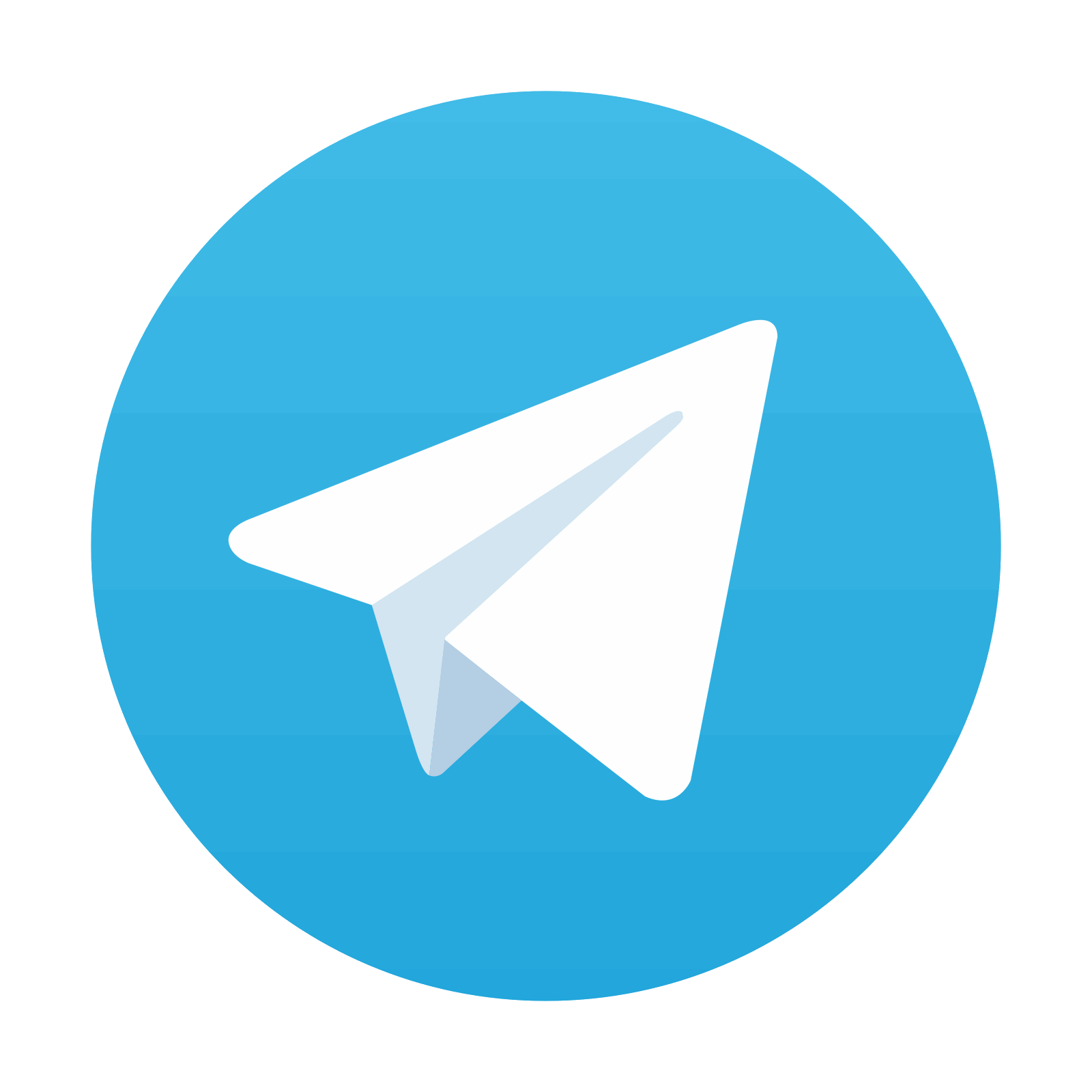
Stay updated, free articles. Join our Telegram channel

Full access? Get Clinical Tree
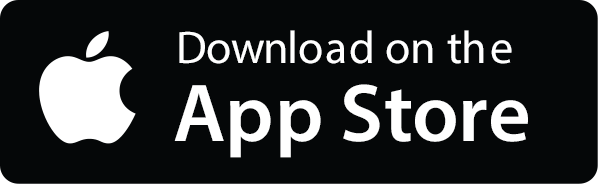
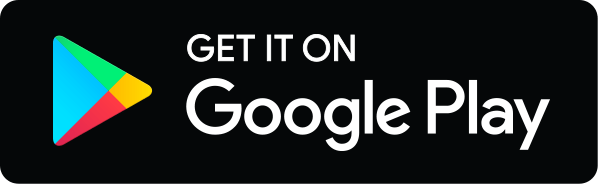