Pathways of complement activation
The classic pathway is initiated by the binding of antibody to antigen, with the antibody being either IgG or IgM bound to the surface of a pathogen. Formation of an antigen–antibody complex causes a conformational change in the Fc portion of the antibody that exposes a binding site for the first complement component, C1q.
The alternative pathway does not require antibody. It is activated by the binding of C3b to hydroxyl and amine groups on the surface of pathogens, including many Gram-positive and Gram-negative bacteria. This pathway is relatively inefficient because its activation requires high concentrations of the various components.
The mannose-binding lectin pathway is more efficient than the alternative pathway. It is initiated by the binding of lectin to glycoproteins or carbohydrates on the surface of pathogens, including Gram-positive and Gram-negative bacteria.
All three pathways of activation converge at the final lytic sequence. Here, C5, C6, C7, C8 and C9 sequentially interact to form a membrane attack complex, which binds to the membrane of the target forming a transmembrane channel through which salts and water can flow, resulting in lysis of the target cell.
The classic pathway links to the adaptive immune system because its activation requires that the host has previously encountered the target and has generated an antibody response to it. The alternative and lectin pathways are considered to be part of the innate immune system because they are activated by microorganisms rather than antibody.
The importance of complement is readily apparent in individuals who lack particular components. For example, classic-pathway deficiencies result in tissue inflammation and individuals with C3 deficiencies are subject to overwhelming bacterial infections.
Limitations of Innate Immunity
Although innate immunity can provide a line of defence against many common microorganisms, such as bacteria, yeasts and parasites, there are many more-sophisticated pathogens, such as viruses or intracellular microorganisms, that the cells of the innate immune system cannot recognise or effectively destroy. This limitation has been addressed by the evolution of the adaptive immune system as a more versatile and adaptable means of host defence.
Adaptive immune response
Adaptive immune responses are mediated by lymphocytes that have the capacity to recognise foreign antigens and distinguish them from self-antigens. In many instances this mechanism confers lifelong protective immunity against re-infection with the same pathogen, which can be intracellular or extracellular.
There are two basic lymphocyte categories: bursa-equivalent cells (B cells) and thymus-derived cells (T cells) (Table 19.2).
B Cells
B cells are so called because of their similarity to lymphocytes found in the bursa of Fabricius organ in birds. B cells develop in the bone marrow, are found in all lymphoid tissues and represent up to 15% of the circulating lymphoid pool. Antibodies on the surface of B cells serve as antigen receptors and binding initiates a series of events whereby antigen is internalised, degraded, complexed with MHC class II molecules and returned to the cell surface. This peptide–MHC complex can then be presented to antigen-specific T cells, which secrete cytokines and induce clonal B cell proliferation and differentiation into antibody-producing plasma cells.
Antibodies
Antibodies, also known as immunoglobulins, are a particularly effective means of combating extracellular organisms such as bacteria, and are efficient at neutralising circulating viruses. In mammals, there are five distinct classes of immunoglobulin (Table 19.3): IgG, IgM, IgA, IgE and IgD. All are made up of a unit comprising two identical light chains and two identical heavy chains but they differ in size, charge, carbohydrate content and amino acid sequence (Figure 19.2). It is the type of heavy chain that determines the class and subclass of antibody; for example, IgM has a mu heavy chain, whereas IgA1 and A2 have alpha 1 and alpha 2 heavy chains, respectively.
Property | IgM | IgG | IgA | IgE | IgD |
---|---|---|---|---|---|
Heavy chain | Mu | Gamma | Alpha | Epsilon | Delta |
Normal serum level (mg/ml) | 1.5 | 13.5 | 3.0 | 0.0003 | 0.03 |
Activates classic complement pathway | + | + | – | – | – |
Binds to phagocyte Fc receptors | ? | + | – | – | – |
Present on membrane of mature B cells | + | – | – | – | + |
Transported in mucosal secretions | – | – | + | – | – |
Induces mast cell degranulation | – | – | – | + | – |
Crosses placenta | – | + | – | – | – |
Fc = crystallisable fragment | Ig = immunoglobulin | + = yes | – = no | ? = not known
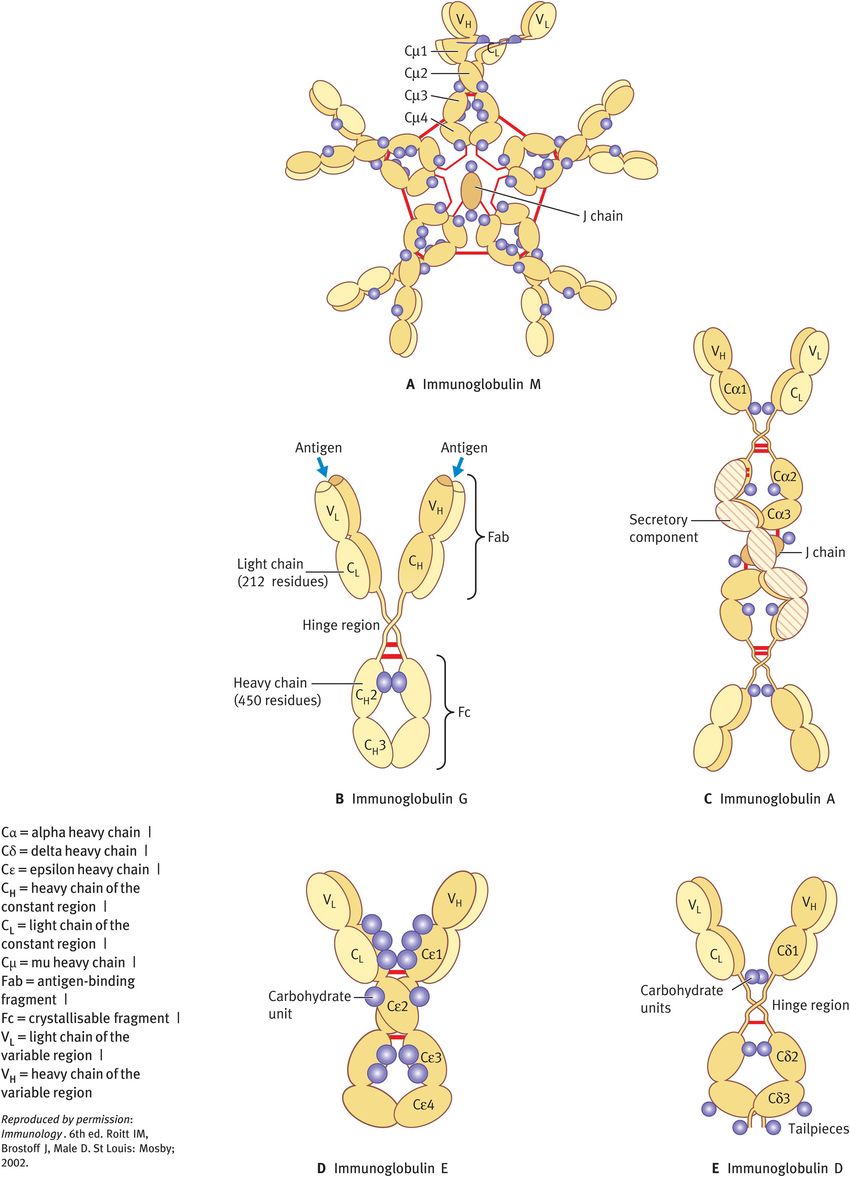
Structure of immunoglobulins
Different parts of an antibody have distinct functions. The antigen-binding fragment (Fab) region comprises the amino-terminal domains of each heavy and light chain (referred to as the variable region) and forms the antigen-binding site, thereby determining the antibody’s antigen specificity. The other part of the antibody, the Fc region, comprises the carboxy-terminal domains of each heavy and light chain (referred to as the constant region). The Fc region determines which effector function will be activated.
All immunoglobulin isotypes, with the exception of IgD, are bifunctional in that they do not only recognise and bind antigen but they also exhibit one or more effector functions.
IgM comprises 5–10% of the serum immunoglobulin pool. It is secreted from plasma cells as a pentamer and is largely confined to the intravascular pool. IgM is the first antibody formed in a primary response to an antigen and is also the first antibody to be synthesised by the newborn baby, but it is unable to cross the placenta. This immunoglobulin is a potent activator of the classic pathway of complement owing to its five complement-binding sites.
IgG comprises 70–80% of the serum immunoglobulin pool. It is the predominant antibody of secondary immune responses and equilibrates between the intravascular and extravascular pools. There are four subclasses with unique effector functions:
IgG1, IgG3 and IgG4 readily cross the placenta and have an important role in protecting the developing fetus.
IgG1 and IgG3 are extremely efficient in activating complement via the classic pathway, IgG2 less so.
IgG1 and IgG3 bind with high affinity to Fc receptors on phagocytic cells and thereby mediate opsonisation.
IgA constitutes 10–20% of the serum immunoglobulin pool. It is the predominant antibody in secretions such as saliva, tears and mucus of the bronchial, digestive and genitourinary tracts. Breast milk is also rich in secretory IgA, which protects the newborn against infection during the first few months of life. IgA participates in secondary immune responses. IgA-containing immune complexes opsonise antigens and induce phagocytosis through Fc receptors. Binding of secretory IgA to microbial antigens prevents their attachment to mucosal cells, thus inhibiting infection and colonisation.
IgE achieves very low serum levels. It binds with high affinity to specific receptors on mast cells and basophils. Cross-linkage of bound IgE by antigen causes degranulation and release of a range of pharmacologically active mediators. IgE can give rise to a variety of allergic manifestations.
IgD comprises less than 0.5% of the serum immunoglobulin pool. It has no known effector functions as a serum protein; instead, it functions as a transmembrane antigen receptor on mature B cells.
Vaccination
The importance of antibody to host defence comes into play when considering the crucial role it plays during vaccination. During infection, a primary antibody response is launched to eliminate the pathogen. This also leads to the generation of long-lived antigen-specific memory B cells, which are restimulated upon subsequent exposure to the same pathogen, resulting in a faster and much more intense secondary response.
It is important to realise that antibodies do not recognise the whole microorganism but particular parts of antigens called epitopes. Successful vaccine development therefore relies upon modification of a pathogen so that it retains its antigenicity (that is, it is still able to generate a primary antibody response) but is innocuous. In essence then, a vaccine essentially replaces the initial infection but still leads to the generation of memory B cells. This consequently provides immunity to vaccinated individuals such that when they are exposed to the microorganism in question, they are able to mount a quick and effective secondary response, leading to protection from and elimination of the pathogen.
T Cells
T cells are the principal mediators of the adaptive immune response and exhibit a wide variety of effector functions including target-cell lysis and cytokine release, which can cause proliferation, differentiation or activation of many different cells of the immune system. Antigen recognition takes place through the T cell receptor (TCR).
Two types of TCR have been characterised:
alpha/beta TCRs, which are expressed on more than 95% of circulating T cells
gamma/delta TCRs, which are rarely expressed on peripheral-blood T cells but are present on populations of T cells in mucosal surfaces (small intestine, pregnant uterus) and liver.
T cell maturation
Alpha/beta T cells are generated in the bone marrow and recognise nonself antigens that are presented by self-MHC class I or class II molecules on the surface of APCs. This specificity is the result of a rigorous selection process.
Following TCR gene rearrangement in the thymus, immature T cells (thymocytes) that recognise self-MHC molecules expressed on cortical epithelial cells with high affinity are positively selected. Those cells that are not selected undergo death by apoptosis.
As the thymocytes migrate to the deeper cortex, they increase their expression of TCR and the cell surface molecules CD3, CD4 and CD8. Those TCRs that recognise self-antigens with high affinity are deleted by a process called negative selection. T cells at this stage of maturation go on to express TCRs at high density and to lose either CD4 or CD8, becoming single-positive (positive for either CD4 or CD8) mature thymocytes.
The mature cells leave the thymus and travel to the secondary lymphoid tissues and periphery, where they have the potential to function as one of the following, depending on whether they express CD4 or CD8:
T helper (Th) cells, which are CD4-positive and MHC class II restricted
cytotoxic T (Tc) cells, which are CD8-positive and MHC class I restricted.
T cells that express a gamma/delta TCR are also generated from bone marrow precursors but differ from T cells that express alpha/beta TCRs in their specificity (they are MHC-unrestricted) and expression of CD4 and CD8 (they are predominantly double-negative).
The mechanism by which T cells become committed to one or the other of these two TCR cell types is largely unknown.
CD4-positive T cells
CD4 is a transmembrane protein that is expressed on T cells and macrophages. It serves as a cell adhesion molecule, facilitating TCR/CD3-mediated signal transduction upon binding of MHC class II molecules on APCs.
CD4-positive T cells can be categorised as Th1 or Th2 cells according to the pattern of cytokines they secrete (Figure 19.3A):
Th1 cells produce IFN-γ and IL-2, which activate macrophages and CD8-positive T cells, promoting cell-mediated immunity.
Th2 cells secrete IL-4 and IL-5, which stimulate B cells to produce antibody, promoting humoral (that is, antibody-mediated) immunity.
Following stimulation by antigen, naïve CD4-positive T cells produce IL-2 and subsequently polarise towards a Th1 or Th2 phenotype, depending on signals received during and after activation (Figure 19.3A). IFN-γ and IL-12 enhance Th1 development, whereas IL-4 promotes differentiation into Th2 cells. The type of APC and the antigen dose may also have a role. It is likely that the polarisation to a Th1 or Th2 immune response occurs through the complex interaction of various factors, rather than on the basis of any single criterion.
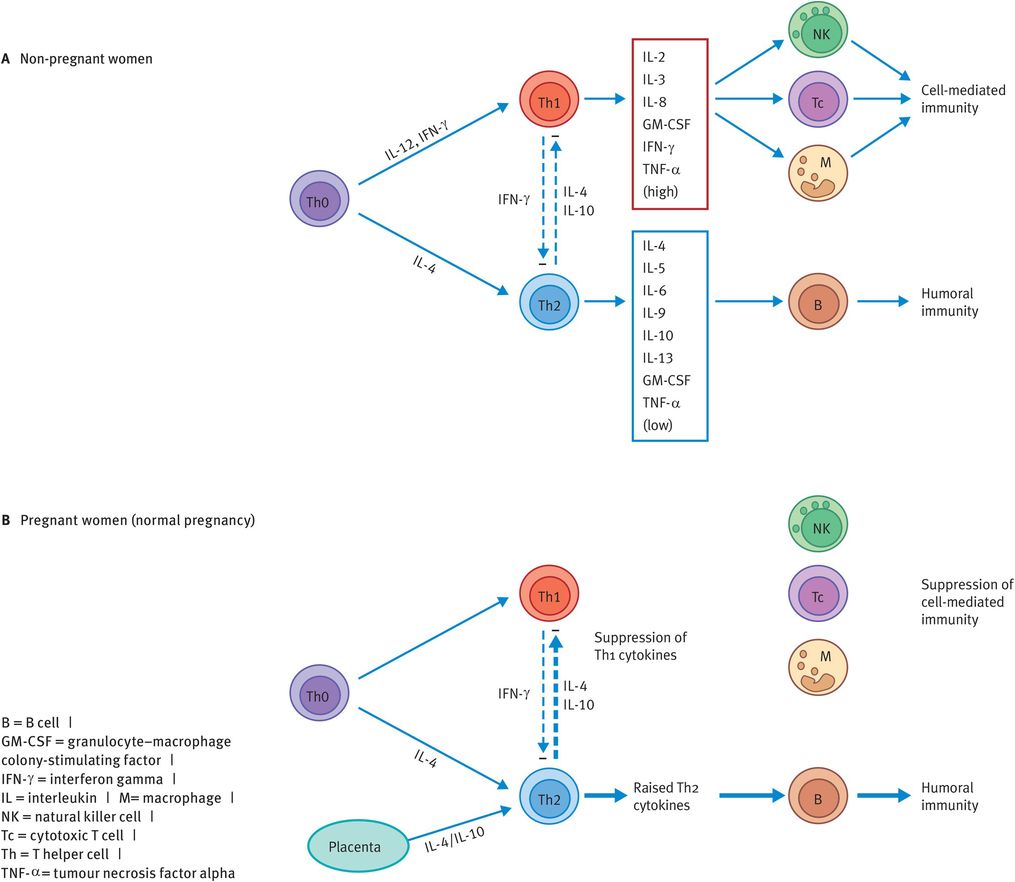
Generation of Th1 and Th2 CD4-positive subsets
Other CD4-positive T cell types are Th3, Th0 and type 1 regulatory T (Tr1) cells:
Th3 cells produce high amounts of transforming growth factor beta (TGF-β).
Th0 cells express both Th1 and Th2 cytokines.
Tr1 cells secrete high levels of IL-10 (and little or no IL-2 and IL-4) leading to immune suppression.
CD8-positive T cells
CD8 serves as a cell adhesion molecule by binding to MHC class I, thereby stabilising the interaction of a class I restricted T cell with a target cell or APC. CD8 expression predominantly identifies a subset of T cells called Tc cells. These can kill tumour cells or virally infected cells following appropriate priming and activation.
In addition to their cytotoxic activity, CD8-positive T cells can also secrete distinct patterns of cytokines. As with CD4-positive cells, CD8-positive precursors can be driven toward a type 1 cytotoxic (Tc1) phenotype by IL-12 and IFN-γ, whereas IL-4 induces the generation of Tc2 cells. Both of these phenotypes retain cytolytic function.
Cytokines
Cytokines are molecules produced by a variety of cell types, including monocytes, macrophages, lymphocytes, endothelial cells, fibroblasts and tissue epithelia, and provide a link between the innate and adaptive immune responses. Although they are often described as hormone-like, cytokines differ from hormones in that their effects are predominantly exerted at a local level. Cytokines are low-molecular-weight proteins that act at very low concentrations by binding to specific cell surface receptors.
A cytokine may act in one of three ways:
in an autocrine fashion by binding to receptors on the membrane of the cell that secreted it
in a paracrine fashion by binding to receptors on cells in the immediate vicinity
in an endocrine fashion by binding to distant cells.
Cytokines can be subdivided into categories (such as interferons, interleukins, chemokines, growth factors) according to their primary function, although there is considerable overlap between these groups.
Interferons
This family of molecules was first recognised by their ability to inhibit viral replication. They are produced early in infection and are pivotal in controlling the spread of a virus until adaptive immunity has had a chance to develop.
IFN-α and IFN-β are produced by virally infected leucocytes and fibroblasts, respectively.
IFN-γ is a product of activated Th1 and NK cells. It has the following functions:
antiviral effects
increasing the expression of both MHC class I and MHC class II molecules
activating macrophages and NK cells
promoting cytotoxic susceptibility of target cells
polarising Th0 cells to the Th1 phenotype.
Interleukins
Interleukins are a large and diverse group of cytokines with a wide range of functions. They are predominantly secreted by lymphocytes. The most important immunoregulatory interleukins are listed in Table 19.4.
Name | Cells secreting this interleukin | Biological function |
---|---|---|
IL-1 | Macrophages and monocytes | Has proinflammatory effects |
IL-2 | T cells | Acts as a growth factor for T cells Stimulates proliferation and differentiation of T cells Increases the cytolytic activity of NK cells |
IL-4 | Th2 cells | Promotes proliferation and differentiation of B cells Upregulates B-cell HLA class II expression |
IL-10 | T cells, B cells, monocytes and mast cells | Has potent anti-inflammatory effects Inhibits the synthesis of IL-2 and IFN-γ by Th1 cells Regulates growth and differentiation of B cells Enhances expression of HLA class II |
IL-12a | Macrophages, dendritic cells and B cells | Enhances the cytolytic activity of CD8+ T cells and NK cells Directs the immune response towards a Th1-type bias by inducing the secretion of IFN-γ, IL-2 and TNF-α |
a Secretion strongly induced by bacteria, bacterial products and certain parasites. | CD8+ = CD8-positive | HLA = human leucocyte antigen | IFN = interferon | IL = interleukin | NK = natural killer | Th = T helper | TNF-α = tumour necrosis factor alpha
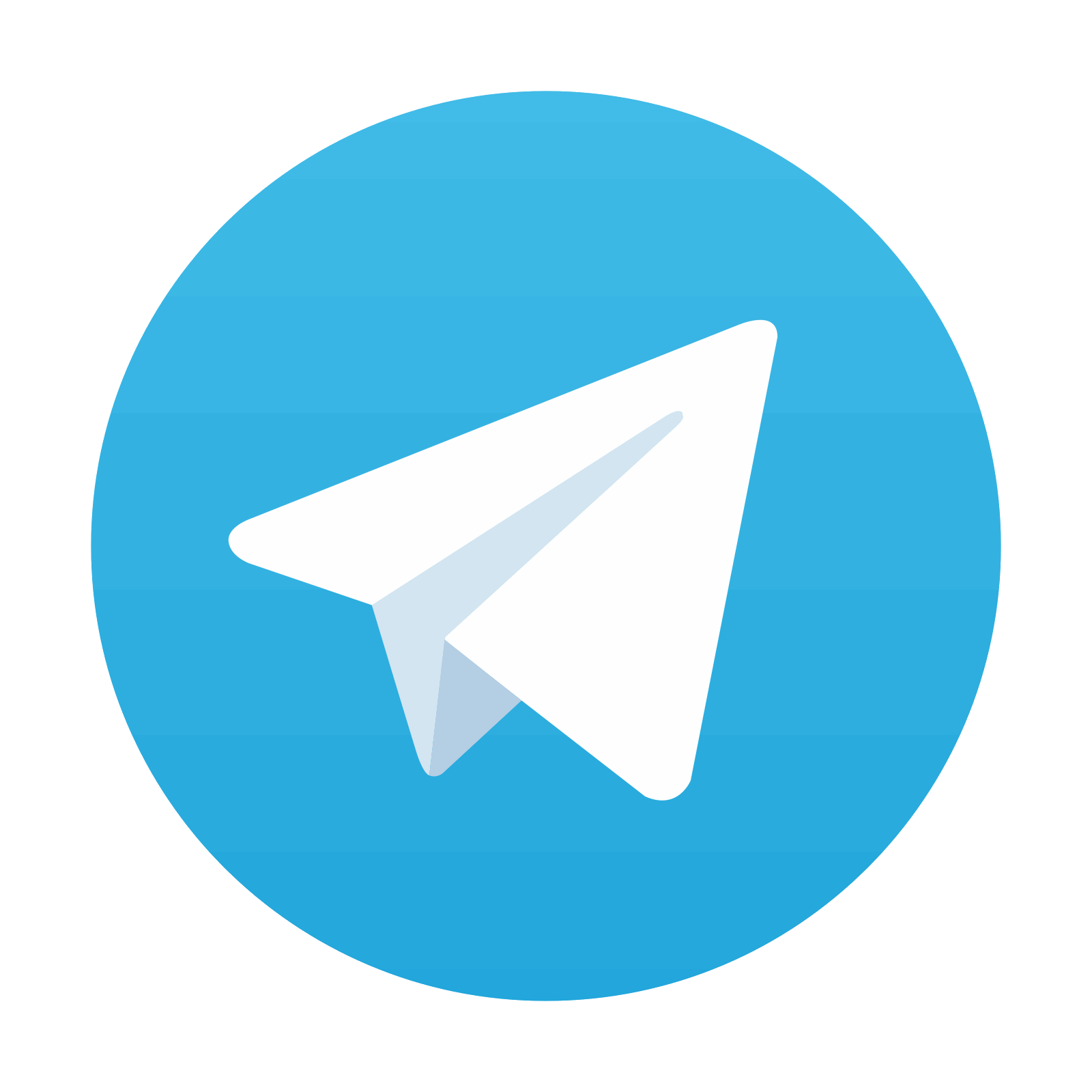
Stay updated, free articles. Join our Telegram channel

Full access? Get Clinical Tree
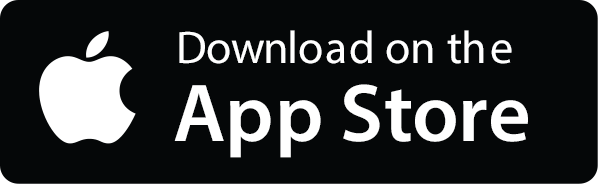
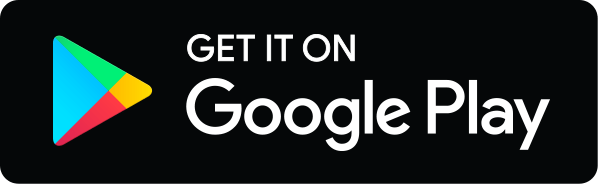
