Approach to the Pediatric Trauma Patient
David P. Mooney
David K. Magnuson
Martin R. Eichelberger
Trauma Department, Children’s Hospital Boston, Boston, Massachusetts 02115.
University Hospital Health System, Rainbow Babies and Children’s Hospital, Cleveland, Ohio 44106.
Emergency Trauma Services, Children’s National Medical Center, Washington, District of Columbia 20010.
BASIC CONSIDERATIONS
Scope of the Problem
More children die from injuries each year than from all other diseases combined (Fig. 20-1). Trauma disrupts more young lives and exacts a higher socioeconomic price than any other pediatric health-related issue in the industrialized world. More lives would be saved each year and more long-term disability prevented by the development of a successful treatment for traumatic brain injury (TBI) than from curing all childhood cancer. Despite this, trauma care receives only a small fraction of the public attention and research funding directed toward less important conditions.
Each year more than 10,000 children and adolescents die of their injuries, and nearly 30,000 suffer permanent disability, most of which is neurologic (1). Annually, one in four children receives medical care for an injury. The societal cost, in terms of both direct medical expenses for care and rehabilitation, lost productivity by care providers, and lost opportunities for socioeconomic contribution, is staggering. Except for prevention, the component of trauma management that offers the greatest chance of limiting this tragedy is rapid and effective resuscitation.
This chapter discusses some of the biochemical and physiologic derangements that are caused by both injury and resuscitation, reviews salient features of resuscitation techniques with emphasis on the child, and describes some areas of controversy and investigation. Its goal is to create a framework of biologic principles and clinical observations within which basic trauma management can be applied to the injured child.
Only a fraction of children who present to an emergency department (ED) for the evaluation and management of an injury require admission. Nationally, fewer than 2% of children admitted to a hospital for the treatment of an injury expire, most often from an overwhelming brain injury. Although vigilance is required during patient evaluation, the fraction of patients who present to an ED with life-threatening injuries is so small that it calls into question the necessity of the traditional “full court press” for every child. Resuscitation should be conducted by personnel experienced enough with the examination of injured children to allow the history and physical findings to guide the evaluation. Evaluation plans in which every patient has a full battery of studies ordered by a junior house officer, performed regardless of the patient’s presentation, are not appropriate for children and may be harmful. Up to 30% of children who are admitted to a hospital for the treatment of an injury develop acute stress response or posttraumatic stress disorder (2). The contribution of unnecessary, involuntary procedures and poor patient and family interaction during the acute resuscitation to this problem cannot be overstated.
Definition of Resuscitation and Impact on Outcome
Resuscitation of the injured child essentially includes all the judgments and actions necessary to reverse and control the sudden alterations in physiologic homeostasis that occur as the result of trauma. It begins with the recognition of serious injury and progresses through basic first aid, advanced prehospital care, maneuvers performed in the ED, and definitive stabilization in the operating room and intensive care unit. Resuscitation is not complete until injuries have been definitively treated and the child displays physiologic stability without continued intervention.
This chapter focuses, however, on a narrower definition of resuscitation—the acute restoration, maintenance, and monitoring of physiologic function immediately after injury.
This chapter focuses, however, on a narrower definition of resuscitation—the acute restoration, maintenance, and monitoring of physiologic function immediately after injury.
![]() FIGURE 20-1. Percentage of childhood deaths by cause, 2001. (From National Center for Health Statistics, Vital Statistics System, 2003, with permission.) |
Trauma, broadly defined, is the application of a force to the body and the body’s response to that force, whether chemical, electrical, or mechanical. Differences between children and adults in their response to these forces, with respect to patterns of injury, physiologic presentation, and management are well recognized. These differences, however, are blurred both by the continuum of ages treated by pediatric surgeons and the increase in participation by children in activities, such as the use of all-terrain vehicles, previously restricted to adults. Nevertheless, important distinctions do exist, and those who treat pediatric trauma patients must recognize and understand these nuances so the resuscitation process addresses the special needs of the injured child.
The principle of a trimodal pattern of trauma-related mortality and morbidity in adults must be modified for children. In the trimodal model, early deaths occur within minutes of the traumatic event from extreme physiologic derangement that exceeds the body’s ability to compensate. Prevention through behavior modification and safety-conscious product design are the only avenues likely to reduce this mortality. A second mortality peak occurs several hours after the insult. Although initial physiologic compensation may have been sufficient to achieve some temporary accommodation, progressive dysfunction and exhausted reserves bring about a critical impairment of oxygen delivery and the patient’s eventual demise. This group may be impacted by aggressive and systematized delivery of emergency medical services.
A third mortality peak occurs days to weeks after the initial injury and represents the end result of processes that begin within hours after injury and progress despite intervention. Accepted paradigms attribute these deaths to uncontrolled systemic autoinflammation leading to multiple organ failure syndrome, also referred to as systemic inflammatory response syndrome. This generalized, unregulated inflammatory response can be initiated by ischemia-reperfusion injury, infection, and products of tissue destruction, and can generate a widespread cascade of cytokine and cellular responses (3). This late peak in trauma-related mortality is unusual in younger children.
PATHOPHYSIOLOGY OF INJURY AND RESUSCITATION
Much of the serious morbidity and mortality that occurs in the early posttraumatic period is caused by impaired oxygen delivery to vital organs due to global circulatory insufficiency (shock) and reduced oxygen content in circulating blood. Hypoxemia may result from many causes, including airway obstruction, ventilatory failure, and compromised cardiac output from hemorrhage or impaired venous return. The immediate consequences of hypoxemia and hypoperfusion after trauma are progressive cellular ischemia leading to dysfunction and cell death. Although effective resuscitation can limit and ultimately reverse cellular ischemia, biochemical changes may have already occurred that instigate an ongoing pathologic cascade of neuroendocrine messengers, inflammatory mediators, cytokines, and activated cells. Furthermore, reperfusion of ischemic tissues may generate a deleterious autoinflammatory and cytotoxic process, referred to as ischemia-reperfusion injury.
Oxygen Delivery, Ischemia, and Reperfusion
Oxygen delivery to metabolically active cells is determined by two parameters—cardiac output and blood oxygen content—which are in turn dependent on variables that may be altered by both injury and therapeutic intervention. Cardiac output is the product of stroke volume and heart rate: cardiac output = stroke volume × heart rate.
Heart rate depends on the chronotropic effects of circulating catecholamines that circulate in increased concentrations immediately after trauma. Stroke volume reflects myocardial performance and is dependent on three determinants: preload, contractility, and afterload.
Preload depends on venous return and is manifest physiologically as left ventricular end-diastolic volume (LVEDV), which is estimated with variable precision by pulmonary artery wedge pressure or central venous (right atrial) pressure. For the most part, as LVEDV increases, so does stroke volume (Starling’s law). This relation is consistent for any contractility; the increase in stroke volume does not reflect a change in contractility, but rather a positive shift along the Starling curve for that given contractility.
![]() FIGURE 20-2. Cardiac response to increased preload (LVEDV) and contractility. LVEDV, left ventricular end-diastolic volume; SV, stroke volume. |
Contractility, defined as the rate of force generation during contraction, determines the position and shape of the Starling curve. An increase in contractility shifts the whole curve to the left so a given LVEDV generates a larger stroke volume (Fig. 20-2). Contractility is determined by the level of catecholamine stimulation and the availability of oxygen.
Afterload describes the systemic vascular resistance (SVR) to left ventricular outflow ratio. It is determined largely by the degree of constriction of arteriolar resistance vessels. SVR is compensatorily increased in hemorrhagic shock and reduced in septic and neurogenic shock. Septic shock is also characterized by a pathologic redistribution of flow through precapillary shunts, circumventing metabolically active tissue.
The other main determinant of oxygen delivery, blood oxygen content, is mainly determined by the concentration of hemoglobin and its oxygen saturation. Dissolved oxygen represents a trivial amount and is generally ignored in most clinical situations. This may change with the development of synthetic perfluorocarbon resuscitation solutions that dissolve large quantities of oxygen. Oxygen saturation of hemoglobin is dependent on the partial pressure of oxygen and the affinity of hemoglobin for oxygen, which is altered by many factors, including temperature, the partial pressure of carbon dioxide, and the pH.
Compromised oxygen delivery in the injured child occurs by many mechanisms, including reduced PO2 from hypoventilation or parenchymal lung injury, reduced hemoglobin due to hemorrhage, reduced myocardial contractility due to direct injury or acidosis, and preload reduction from hypovolemia, tension hemopneumothorax, or cardiac tamponade. Virtually every resuscitative intervention is directed toward manipulating these variables. In most cases, inadequate oxygen delivery is produced primarily by circulatory insufficiency, a condition loosely referred to as shock.
Shock is defined as a state in which perfusion is inadequate to meet the global demands of metabolically active cells. The classifications of shock are hypovolemic from hemorrhage, or decreased venous return (e.g., tension pneumothorax), cardiogenic from myocardial dysfunction or tamponade, septic from vasomotor instability secondary to endotoxemia, and neurogenic from spinal cord injury. Although hemorrhage is the most common cause of shock in the injured child, the possibility of other causes requires diligence for prompt recognition and specific treatment. Cardiogenic shock with tension pneumothorax is due to both elevated intrathoracic pressure and mediastinal displacement with mechanical distortion of the large veins that return blood to the right atrium. Pleural decompression dramatically improves both problems. Cardiogenic shock from myocardial contusion, although rare in children, may require invasive hemodynamic monitoring and inotropic support. Cardiac tamponade limits stroke volume by preventing normal cardiac filling in diastole. This may be temporized by volume infusion to increase central venous pressure and to overcome the decreased effective compliance, but may require pericardiocentesis or pericardotomy. Neurogenic shock, characterized by hypotension, bradycardia, and hypothermia secondary to acute interruption of sympathetic outflow, responds initially to volume expansion but may also require vasopressor therapy with alpha agonists.
As oxygen delivery falls, oxygen extraction increases. About 25% of available arterial oxygen is used under normal conditions. The extraction process is limited by a declining PO2 gradient between capillary blood and intracellular mitochondria, resulting in a maximal extraction ratio of 50% to 70%. As oxygen delivery falls, despite maximal extraction, cell function shifts to less efficient anaerobic metabolism, which generates lactic acid. Accumulation of lactic acid affects cellular processes and may further impair oxygen delivery.
If cellular hypoperfusion progresses, cell membrane dysfunction occurs due to failure of the Na+-K+-ATPase pump, altering membrane permeability and allowing the entry of sodium and water. The result is cell swelling and contraction of the interstitial fluid compartment. With further ischemia, oxidative phosphorylation is uncoupled, leading to failure of cellular respiration and depletion of adenosine triphosphate. These events are followed rapidly by swelling of the endoplasmic reticulum, the appearance of blebs on the surface membrane, and changes in microfilament and microtubule function. Mitochondrial swelling, protein denaturation, nucleic acid condensation, and disruption of membrane structure indicate irreversible cell injury.
Even if ischemia is reversed by restoration of oxygen delivery, the process of ischemia and reperfusion can cause direct cellular injury. This is explained by the fact that, in ischemic cells, enzymatic pathways are induced that convert molecular oxygen to a variety of toxic oxygen metabolites.
Toxic oxygen metabolites produce cellular damage in a variety of ways. They oxidize nucleic acids and cause DNA nicking; cause membrane lipid peroxidation, which alters membrane fluidity and results in leakage; and cross-link and degrade proteins, rendering them nonfunctional. These metabolites also initiate proinflammatory and chemotactic signals that recruit activated neutrophils (PMNs) to the reperfused tissue (4). Microvascular endothelial and intestinal epithelial cells contain particularly high concentrations of xanthine oxidase. Diffuse injury to these barriers by reactive oxygen metabolites can have profound consequences for cellular integrity. Studies have documented an association between experimental ischemia-reperfusion injury of the intestine and diffuse lung injury that is mediated by cytokines and PMNs, and has histologic and clinical similarities to adult respiratory distress syndrome (ARDS) (5). The premise that such processes are clinically important is supported by investigations that documented protection against ischemia-reperfusion injury by pretreatment with a variety of free-radical scavengers and inhibitors, such as allopurinol, superoxide dismutase, and catalase.
RESUSCITATION PRINCIPLES
Preparation
Proper evaluation and management of the injured child requires planning well in advance of patient arrival. Appropriate equipment and personnel should be in place. Prehospital care protocols, triage criteria, and trauma team activation triggers must be worked out in advance to determine the optimal prehospital and ED response. Patient care protocols should be based as much as possible on a continuous review of existing data and not on an unfortunate anecdote. On patient arrival, an appropriate team of trained personnel knowledgeable of their clearly defined roles must be present and ready to care for the patient.
Triage criteria vary widely by institution. A tiered trauma team response—with the most injured children triggering the largest team response, a moderately injured child triggering a lesser response, and a lesser injured patient managed without a team response—has been demonstrated to improve the efficiency of resuscitation (6). This implies that sufficient and accurate prehospital information has been obtained to allow determination of injury severity. The Trauma Score, the Revised Trauma Score, and the Pediatric Trauma Score have all been proposed for this purpose. Although each method has been demonstrated to have significant flaws, any of the methods are reasonably accurate. Team activation using prehospital information has been demonstrated to be as efficient as using activation based on physician-generated information (7).
Trauma response teams may number more than a dozen different personnel from various departments and physical locations within the facility or at home. Some personnel are needed immediately, whereas others may simply need to be on standby. Communication centers, staffed with dispatch-level personnel, can make this process accurate and efficient.
Equipment
To properly care for injured children, the appropriate equipment must be available. The rapid selection of the correct-size equipment is an ongoing problem with pediatric care. Length-based equipment management and selection systems, such as the Broselow tape have been demonstrated to be accurate in most situations and may be used for equipment sizes, body weights, and medication dosing (8). The American Academy of Pediatrics has developed a list of the equipment necessary to care for injured children (9). Every ED that may be called on to resuscitate an injured child should have the necessary pediatric equipment available to do so.
Roles
The roles played by each member of the trauma response team should be worked out well in advance so the evaluation and early management phase of care proceeds as smoothly as possible. Most important, one physician needs to be identified in advance as the team leader. If not already determined, that person assigns each person in the room to a specific role. Additional personnel are not allowed in the trauma room, unless invited. One physician, with the assistance of a respiratory therapist, must be in charge of airway management. These personnel also remain responsible for maintenance of cervical spine immobility. One physician performs the primary and secondary surveys, and conveys their findings to the team at large. An additional physician may be available to assist with starting intravenous (IV) lines and other procedures. Two nurses interface the patient with the appropriate monitoring devices, place IVs as necessary, and obtain blood for laboratory tests. Urinary catheter placement and the use of warming devices may also fall under their domain. One nurse or technician is responsible for recording the evaluation, management, and ED course of the patient. A radiology technician should be available to promptly take and develop needed radiographs, and a laboratory technician may be helpful in getting blood samples promptly to the lab. Social work, security, and patient care technicians may also be helpful. This, of course, implies that significant staffing is available. In situations in which personnel are limited, each team member may fulfill multiple roles.
More important than the number of personnel present is the clear definition and understanding of team roles prior to patient arrival.
More important than the number of personnel present is the clear definition and understanding of team roles prior to patient arrival.
Prehospital Care
The resuscitation process begins when bystanders or prehospital personnel first encounter the child in the field. The fate of any given child can turn on the decisions and interventions that transpire during these first crucial moments.
Physician involvement in the prehospital arena is necessary to provide pediatric injury-related education, assist with the development of pediatric patient care protocols, and participate in quality improvement efforts. An appropriately senior representative of the trauma program should be actively engaged. This involvement may prove more valuable to injured children than improvements in the inpatient arena.
The prehospital arena is unfamiliar to most surgeons. Its governance system is often through city or county governments, fire departments, or separate governmental bodies. Training levels vary greatly across the nation and within regions from predominantly an Emergency Medical Technician–Basic response to full paramedic coverage. Airway management issues, mainly in the prehospital arena, are the greatest preventable cause of mortality and long-term morbidity in pediatric injury care.
In general, children fare worse than adults in the out-of-hospital phase of resuscitation. The injury-adjusted death rate for children is twice that of adults (10). Similarly, the survival rate for out-of-hospital cardiac arrest in children is only one-half that of adults. Although part of this discrepancy results from the different causes of cardiac arrest in children and adults, an equal part is due to inadequate training with children. Indeed, of the 110 hours of training required for Emergency Medical Technician–Basic national certification, the most common prehospital certification by far, only 6 hours have to do with children. The failure rate for resuscitation interventions in the field is approximately twice as high in children as adults. The most important prehospital intervention for injured children is airway management, yet many series report failure rates for prehospital endotracheal intubation of injured children that approach 50% (11). One series revealed that more than 70% of prehospital scene intubations failed to meet guidelines for endotracheal tube size or depth (12). Unfamiliarity with pediatric skills is understandable because although trauma is the most common indication for pediatric ambulance runs, such runs account for less than 10% of total paramedic patient volume in most metropolitan areas.
The primary objectives for prehospital personnel are
Recognition and treatment of immediately life-threatening problems
Assessment of the mechanism of trauma and extent of injuries
Physiologic stabilization for transport
Gathering of pertinent medical data, if available
Rapid triage to the appropriate-level pediatric trauma facility
Add to these the additional challenges of comforting a terrified and hurt child, as well as a distraught parent, and the paramedic’s task becomes formidable. For these reasons, and because opportunities to resuscitate children in the field are infrequent, field personnel function best by adopting strict protocols to treat the injured child. The priorities and techniques associated with pediatric field resuscitation are similar to those described later for ED care.
Primary Survey and Treatment of Life-threatening Injuries
When the injured child encounters medical personnel, whether in the field or in the emergency room, events transpire in a rapid sequence that is dictated by a systematic protocol to recognize and treat acute injuries. This approach is designed to standardize diagnostic and treatment decisions so individual variations in patterns of injury do not distract care providers from recognizing and treating apparently subtle injuries that can have a profound impact on morbidity and mortality. This systematic framework comprises a primary survey, a resuscitation phase, and a definitive secondary survey (Fig. 20-3). The primary survey is the initial process of identifying and temporizing injuries that are potentially life threatening. It is characterized by its reliance on simple observations to assess physiologic derangement and immediate intervention to prevent death. The components of this approach include attention to airway, breathing, circulation, disability, and exposure— the ABCs of resuscitation.
Airway and Cervical Spine Control
Airway compromise is typically caused by an altered sensorium, but may be caused by foreign bodies, blood, and secretions in the oral cavity; maxillofacial trauma with injury to supporting structures; and direct laryngotracheal trauma. Assessment of the airway includes inspection of the oral cavity; manual removal of debris, loose teeth, and soft tissue fragments; and aspiration of blood and secretions with mechanical suction. Fortunately, the large majority of injured children have an intact airway. If a child is neurologically intact, phonates normally, and is ventilating without stridor or distress, invasive airway management is not needed. If questionable, airway patency can be improved in the spontaneously breathing child by the use of the jaw-thrust or chin-lift maneuvers.
An airway that is unsecured because of coma, combativeness, shock, or direct airway trauma requires endotracheal intubation. However, the value of prehospital endotracheal intubation in children has been called into question. Gauche et al. found a worsened survival rate among children who underwent prehospital intubation as compared with those managed with bag-valve-mask ventilation (13). Two studies have demonstrated worsened survival in adults with moderate traumatic brain injuries (TBI)s who were intubated prior to arrival to the hospital (14,15). Given the infrequency of pediatric prehospital intubation, only the busiest providers have sufficient exposure to this maneuver to maintain appropriate skill levels. A more appropriate focus, both in the prehospital arena and in the ED is the maintenance of adequate oxygenation and ventilation, rather than the performance of any specific procedure.
Because the complication rate for pediatric prehospital intubation remains high, typically secondary to inappropriate tube size or position, it must be assumed for all patients that the airway is unstable and that any equipment used by prehospital or another hospital’s providers has malfunctioned or become malpositioned.
Nasopharyngeal and oropharyngeal airways can improve airway management during bag-mask ventilation, and may help with providing adequate oxygenation and ventilation until definitive control is established. In most cases, orotracheal intubation with inline cervical spine stabilization is the preferred approach to airway control. Although nasotracheal intubation is recommended in nonapneic adults with potential cervical spine injury, this approach is unnecessary and poorly tolerated in children. Because axial traction can further disrupt injured cervical ligaments in children, the neck should be manually stabilized in a neutral position, not pulled, during intubation (16).
Several unique aspects of the anatomy of the pediatric airway may affect management technique. The child’s larynx is more anterior than the adult’s. Removing the anterior half of the rigid cervical collar allows access to the neck for gentle cricoid pressure. The pediatric epiglottis is
shorter, less flexible, and tilted posteriorly over the glottic inlet. Direct control of the epiglottis with a straight laryngoscope blade, such as a Miller, is usually necessary for proper visualization of the vocal cords, which can be easily damaged. The narrowest point in the pediatric airway is the subglottic trachea at the cricoid ring, as opposed to the glottis in adults. Therefore, passage of the endotracheal tube through the cords does not guarantee safe advancement into the trachea or avoidance of subglottic injury. The pediatric airway is shorter than the adult’s, and endobronchial intubation is a common mistake.
shorter, less flexible, and tilted posteriorly over the glottic inlet. Direct control of the epiglottis with a straight laryngoscope blade, such as a Miller, is usually necessary for proper visualization of the vocal cords, which can be easily damaged. The narrowest point in the pediatric airway is the subglottic trachea at the cricoid ring, as opposed to the glottis in adults. Therefore, passage of the endotracheal tube through the cords does not guarantee safe advancement into the trachea or avoidance of subglottic injury. The pediatric airway is shorter than the adult’s, and endobronchial intubation is a common mistake.
Because of the narrower subglottic trachea, uncuffed or low-pressure cuffed tubes should be routinely used in children 8 years of age or younger. The use of low-pressure cuffed tubes, even in children younger than 8 years of age, may allow more flexibility in patients with conditions such as a pulmonary contusion or aspiration pneumonitis that might be expected to require ventilation with higher than typical levels of positive pressure.
The technique of intubation depends on the urgency of establishing an airway. All intubation attempts, if possible, should be preceded by preoxygenation with a bag-valve-mask system and an oral airway if accepted by the patient. This attention to the goal— ventilation and oxygenation, rather than the rushed performance of a procedure—may lead to a higher percentage of successful intubations without the inherent hypoxemia, hypercarbia, and other trauma that may accompany hasty intubation attempts. An adequate oxygen saturation (i.e., more than 95%), as measured by pulse oximetry, is attempted by bag-mask ventilation with 100% oxygen. Thoracic trauma can preclude normal gas exchange and make attainment of an adequate oxygen saturation impossible before intubation. Inducing mild hypocarbia by hyperventilation is advantageous in preventing carbon dioxide accumulation during attempted intubation—a condition that can exacerbate intracranial hypertension.
Premedication with intravenous sedatives and muscle relaxants is also helpful in preventing exacerbations of intracranial hypertension. Appropriate sedatives include short-acting barbiturates or benzodiazepines (midazolam or diazepam). Muscle relaxation is achieved with short-acting nondepolarizing agents (vecuronium) or shorter-acting depolarizing agents (succinylcholine). The presence of burns and devitalized tissue precludes the use of succinylcholine because of the risk of hyperkalemia. All sedatives have myocardial depressant effects and should be avoided in the presence of hypotension. In children younger than 10 years of age, in whom vagally mediated bradycardia is a risk during tracheal manipulation, premedication with atropine or glycopyrrolate may be helpful.
Even in patients with significant maxillofacial trauma, the airway is typically protected and orotracheal intubation should be attempted. In the rare circumstance when endotracheal intubation is not possible because of laryngotracheal trauma or unusual airway anatomy, provision of a surgical airway is mandatory. For children older than 10, the preferred approach is surgical cricothyrotomy. The cricothyroid membrane is a readily identifiable subcutaneous structure, lying immediately subjacent to the thyroid cartilage. This membrane may be exposed through a vertical skin incision directly over it and can be incised horizontally to accommodate a small, uncuffed endotracheal tube. Because the membrane is in a superficial location, fewer complications related to inadvertent venous injury occur than with emergency tracheostomy. Tracheostomy, a rare procedure in injured children, is more difficult to perform in the emergency setting because of the trachea’s deeper location and the possibility of vascular injury or thyroidal laceration. Conversion of a cricothyrotomy to a formal tracheostomy within 48 hours is prudent in most instances to avoid subglottic stenosis. A laryngeal mask airway may provide another means of establishing emergency airway control for ventilation of the comatose child who cannot otherwise be intubated, especially in the prehospital arena.
In smaller children, the cricoid cartilage is a delicate structure easily injured by cricothyrotomy. To avoid this complication, children younger than 10 years of age should undergo needle cricothyrotomy and jet insufflation of the trachea. A 14- to 16-gauge IV catheter is used to access the tracheal lumen through the cricothyroid membrane and is connected to a 100% oxygen source at a flow rate of about 12 L per minute. A Y-connector is interposed in the tubing, leaving one limb of the connector open to air. By alternately covering and uncovering this side limb, oxygen is insufflated into the trachea under pressure. By providing an inspiration/expiration ratio of 1:3, adequate oxygenation can be maintained indefinitely. This method is limited by carbon dioxide accumulation to about 40 minutes, allowing time for advanced airway maneuvers, such as fiberoptic intubation or formal tracheostomy, to be performed.
Breathing
Once a secure airway is confirmed, adequacy of ventilation is evaluated and supplemental oxygen is continued. Compromised ventilation in the trauma victim usually results from one of two general causes: head injury with loss of spontaneous ventilatory drive, or thoracic injury causing interference with mechanical bellows function and lung expansion. Recognition of the former is usually straightforward, whereas recognition of the latter requires a deliberate survey of the thorax. Impaired bellows function can result from any of the following: filling of the pleural space with air or fluid, causing collapse and displacement of the lung; reduction in pulmonary compliance; loss of chest wall integrity; or loss of diaphragmatic function. The potential seriousness of these injuries is underscored by the fact that mortality rates for thoracic trauma in children may approach 25% (17).
The pleural space may be occupied by air (pneumothorax), blood (hemothorax), and rarely lymph (chylothorax) or viscera (ruptured diaphragm). The resulting compression of pulmonary parenchyma causes an impairment of gas exchange sufficient to produce respiratory distress. In the case of traumatic rupture of the diaphragm, loss of muscular integrity also has a direct effect on bellows function. If the contents of the pleural space are under increased pressure or tension, the mediastinum is displaced to the opposite side, which can cause both compression of the contralateral lung and a critical reduction of venous return to the right atrium.
Loss of chest wall integrity presents in two ways. Flail chest, a rare occurrence in children, occurs when multiple adjacent fractures create an isolated segment of the thoracic cage that is disarticulated from the remainder, resulting in paradoxical movement and impairment of the patient’s ability to increase intrathoracic volume during inspiration. This problem is most pronounced during spontaneous breathing and does not apply during assisted positive-pressure breathing. In addition, flail chest is accompanied by a significant underlying pulmonary contusion. Regions of parenchymal hemorrhage and edema cause a ventilation-perfusion mismatching and a decrease in pulmonary compliance, which dramatically increases the work of breathing. These effects can precipitate ventilatory failure, either acutely or more gradually, once compensatory mechanisms have been exceeded. A second, rare chest wall injury is an open pneumothorax. The cross-sectional area of these wounds offers less resistance to air flow than does the tracheobronchial tree. Air flows preferentially through the wound during inspiration, resulting in hypoventilation and lung collapse. A tension pneumothorax may result if the exit of air from the wound is prevented by loose tissue acting like a flap-valve mechanism. The pathophysiology of this injury is also limited to spontaneous breathing and is mitigated by positive-pressure ventilation.
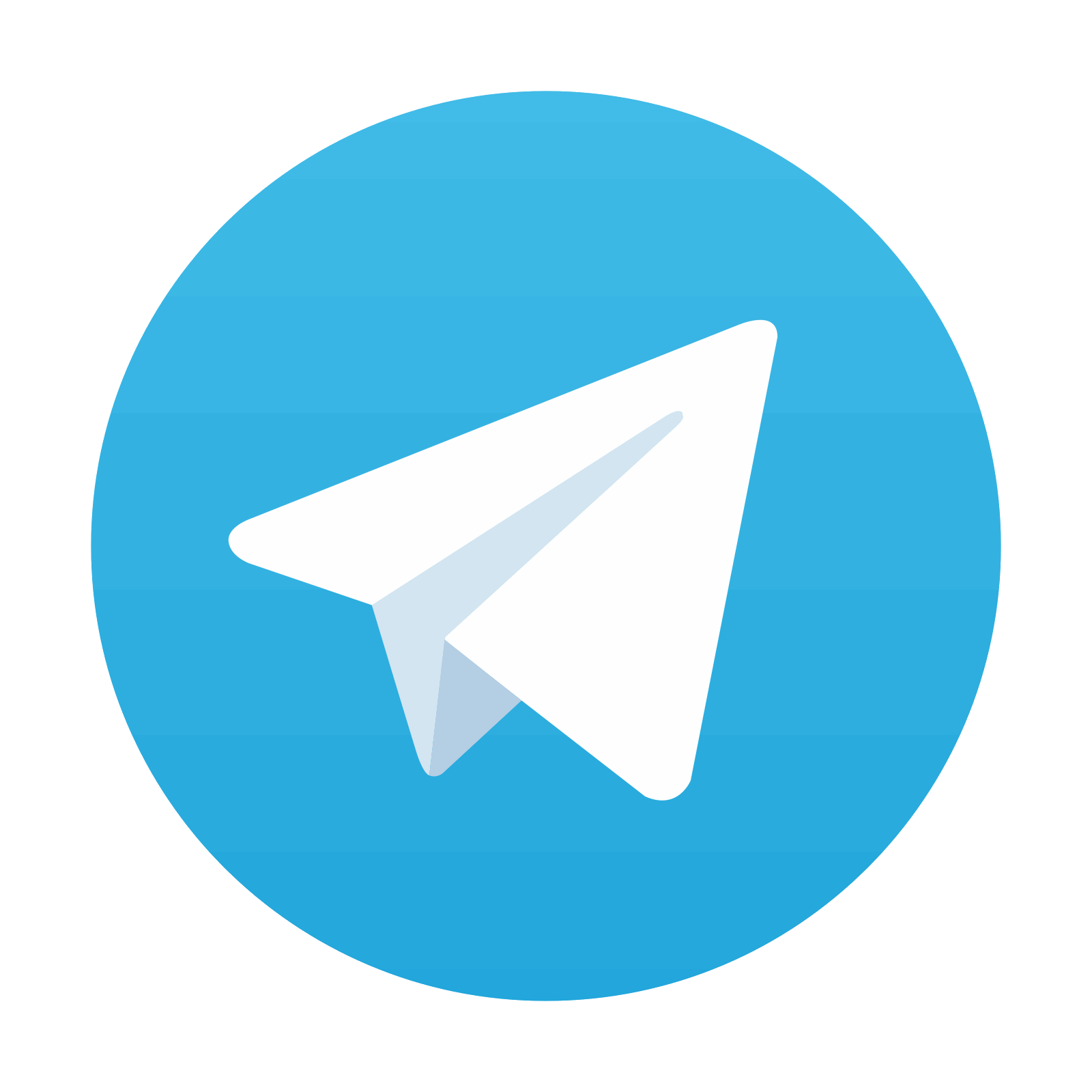
Stay updated, free articles. Join our Telegram channel

Full access? Get Clinical Tree
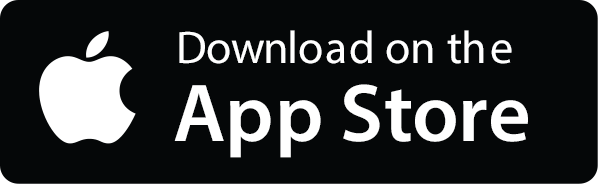
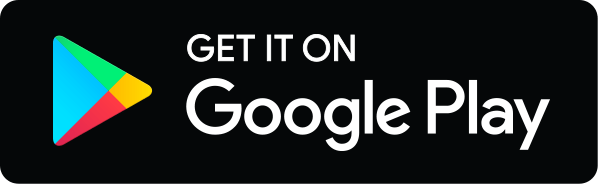
