Chapter 6
Antepartum Fetal Assessment and Therapy
Teresa Marino MD, Joong Shin Park MD, PhD, Errol R. Norwitz MD, PhD
Chapter Outline
PRENATAL CARE IN LOW-RISK PREGNANCIES
PRENATAL CARE IN HIGH-RISK PREGNANCIES
SPECIAL TECHNIQUES FOR ANTEPARTUM SURVEILLANCE
SPECIAL CIRCUMSTANCES REQUIRING ADDITIONAL FETAL SURVEILLANCE
Abnormal Serum Analyte and Nuchal Translucency Screening with Normal Fetal Karyotype
Obstetric care providers have two patients, the mother and the fetus. Although assessment of maternal health is relatively straightforward, assessment of fetal well-being is far more challenging. Several tests have been developed to assess the fetus during pregnancy, including some that are recommended for all pregnancies (e.g., ultrasonography for pregnancy dating) and others that are reserved only for women with pregnancy complications (e.g., middle cerebral artery Doppler velocimetry in pregnancies with isoimmunization). In addition, a limited number of fetal interventions are employed to improve fetal outcome, including some that are used frequently, such as maternal corticosteroid administration, and others that are used much more rarely, such as intrauterine fetal procedures. A review is presented here of the tests available to assess fetal well-being in both low- and high-risk pregnancies and of the fetal therapies used during the antepartum period.
Prenatal Care in Low-Risk Pregnancies
Determination of Gestational Age
The mean duration of a singleton pregnancy is 280 days (40 weeks) from the first day of the last normal menstrual period in women with regular 28-day menstrual cycles. Term, defined as the period from 37 weeks’ (259 days’) to 42 weeks’ (294 days’) gestation, is the optimal time for delivery. Both preterm births (defined as delivery before 37 weeks’ gestation) and post-term births (delivery after 42 weeks’ gestation) are associated with increased perinatal and neonatal morbidity and mortality. Evaluation of fetal growth, efficient use of screening and diagnostic tests, appropriate initiation of fetal surveillance, and optimal timing of delivery all depend on accurate dating of the pregnancy.
A number of clinical, biochemical, and radiologic tests are available to determine gestational age (Box 6-1).1,2 Determination of gestational age is most accurate in early pregnancy, and the estimated date of delivery (EDD) should be established at the first prenatal visit. Embryo transfer dating in women undergoing in vitro fertilization (IVF) is the most accurate clinical dating criterion. Among women with regular menstrual cycles who conceive spontaneously, if the first day of the last menstrual period (LMP) is known and if uterine size is consistent with dates by clinical examination, then Naegele’s rule (subtract 3 months and add 7 days to the LMP) can be used to determine the EDD. Menstrual dating is known to be inaccurate in women taking oral contraceptives, in women who conceive in the immediate postpartum period, and in women who have irregular menstrual cycles or a history of intermenstrual bleeding. Moreover, clinical examination of uterine size can be inaccurate in women with a high body mass index (BMI), uterine fibroids, or a multifetal pregnancy. For these reasons, reliance on standard clinical criteria alone to determine the EDD will lead to an inaccurate diagnosis, with a tendency to overestimate gestational age.3–6 One study reported that reliance on LMP alone leads to a false diagnosis of preterm birth and post-term pregnancy in one fourth and one eighth of cases, respectively.7 Use of other historic factors (e.g., the date of the first positive pregnancy test result or the first perceived fetal movements [“quickening”]) and physical findings (e.g., the date when fetal heart sounds are first audible) may help obstetric providers determine the EDD more accurately (see Box 6-1).
Most early pregnancy tests involve the identification and quantification of human chorionic gonadotropin (hCG), a hormone produced by the syncytiotrophoblast of the fetoplacental unit.8,9 Levels in the maternal circulation increase exponentially to a peak of 80,000 to 100,000 mIU/mL at 8 to 10 weeks’ gestation and then decrease to a level of 20,000 to 30,000 mIU/mL for the remainder of the pregnancy. Commercially available hCG test kits can detect concentrations as low as 25 to 50 mIU/mL in serum or urine, which are typically evident 8 to 9 days after conception.
Uncertainty in dating parameters should prompt ultrasonographic assessment of gestational age. Transabdominal ultrasonography can identify an intrauterine sac in 94% of eutopic (intrauterine) pregnancies once the serum hCG concentration is 6000 mIU/mL or higher.10 With the use of transvaginal ultrasonography, an intrauterine pregnancy can typically be confirmed at a serum hCG level of 1500 to 2000 mIU/mL.11 Failure to confirm an intrauterine sac at these hCG levels should raise concerns about an abnormal pregnancy (e.g., ectopic pregnancy, missed abortion) and requires further evaluation. A fetal pole and cardiac activity should be visible at a serum hCG concentration of approximately 1700 mIU/mL (5 to 6 weeks) and 5400 mIU/mL (6 to 8 weeks), respectively. The EDD derived from ultrasonographic evaluation should be used when there is a 5- to 7-day discrepancy from LMP dating in the first trimester.11
In the first trimester, the fetal crown-rump length (CRL) is the most accurate determinant of gestational age (± 3 to 5 days). In the second trimester, the biparietal diameter (BPD) and length of the long bones (especially femur length) are the ultrasonographic measurements used most often to determine gestational age. Of these, the BPD is the more accurate indicator with a variation of ± 7 to 10 days.12 Two large clinical studies of approximately 50,000 pregnancies demonstrated that a second-trimester BPD measurement, when used instead of menstrual dating to establish the EDD, resulted in a significant increase in the number of women who delivered within 7 days of their due dates and a 60% to 70% reduction in the number of pregnancies continuing post term.13,14 After 26 weeks’ gestation, the variation in the BPD measurement is greater (± 14 to 21 days), thereby making it less valuable in estimating gestational age.12 Both femur length and humerus length correlate strongly with the BPD and gestational age and are sometimes used for additional confirmation.15 By contrast, because abdominal circumference (AC) reflects fetal nutritional status and growth, it is less accurate than either BPD or femur length. All fetal biometric measurements are subject to some degree of error, so a number of techniques have been used to predict gestational age more accurately. Serial determinations of gestational age at 2- to 3-week intervals may be more accurate than a single determination in the third trimester to confirm dating and eliminate the possibility of fetal growth restriction.
Routine Ultrasonography
Routine early ultrasonography significantly improves the accuracy of gestational age dating.3–6,16–19 Early ultrasonography can also detect pregnancy abnormalities (e.g., molar pregnancy), major fetal structural abnormalities (e.g., anencephaly), and multiple pregnancy. Although recommended in Europe, the practice of routine ultrasonography for pregnancy dating has not been recommended as a standard of prenatal care in the United States.20,21
The usefulness of routine second-trimester ultrasonography in all pregnant women remains a subject of debate. Early studies suggested an improvement in perinatal outcome with its use.17,22–25 For example, one prospective clinical trial in Helsinki, Finland, randomly assigned 9310 low-risk women either to a single screening ultrasonographic examination at 16 to 20 weeks’ gestation or to ultrasonography for obstetric indications only; a significantly lower perinatal mortality rate was found in the screening ultrasonography group (4.6 versus 9.0 per 1000 births, respectively).18 This difference was due in part to earlier detection of major fetal malformations (which prompted elective abortion) and multiple pregnancies (which resulted in more appropriate antenatal care) with the screening examination. As expected, routine ultrasonography also led to improved pregnancy dating and a lower rate of induction of labor for post-term pregnancy.18
In contrast, a subsequent large multicenter randomized clinical trial involving 15,151 low-risk women in the United States (designated as the RADIUS study) concluded that screening ultrasonography did not improve perinatal outcomes and had no impact on the management of the anomalous fetus.19,26,27 Although this trial was adequately powered, it has been criticized for the highly selective entry criteria (by one estimate, less than 1% of pregnant women in the United States would have been eligible28) and the selection of primary outcomes (perinatal morbidity and mortality) that were inappropriate for the low-risk population studied. In addition, only 17% of major congenital anomalies were detected before 24 weeks’ gestation in the routine ultrasonography group, so the rate of elective pregnancy termination for fetal anomalies was significantly lower than that in the Helsinki study. The skill and experience of the ultrasonographer is also an important variable in these studies.
Evaluation of Fetal Growth
Normal fetal growth is a critical component of a healthy pregnancy and the subsequent long-term health of the child. Maternal weight gain during pregnancy is at best an indirect measure of fetal growth, because much of the weight gain during pregnancy is the result of fluid (water) retention. Earlier recommendations for weight gain in pregnancy were based on the Institute of Medicine (IOM) guidelines published in 1990.29 In 2009, the IOM revised their 1990 recommendations to include an upper limit of weight gain for obese women (9 kg [20 lb]), and they altered the lower limit of weight gain from 6.8 kg (15 lb) to 5 kg (11 lb) (Table 6-1); they also recommended that all women try to be within the normal BMI range when they conceive.30
TABLE 6-1
Recommendations for Weight Gain in Pregnancy
Mother’s Body Mass Index | Recommended Weight Gain |
18.5 to 24.9 kg/m2 (normal weight) | 11.2 to 15.9 kg (25 to 35 lb) |
25 to 29.9 kg/m2 (overweight) | 6.8 to 11.2 kg (15 to 25 lb) |
>30 kg/m2 (obesity) | 5.0 to 9.0 kg (11 to 20 lb) |
Data from the Institute of Medicine. Nutritional status and weight gain. In Nutrition during Pregnancy. Available at http://iom.edu/Reports/2009/Weight-Gain-During-Pregnancy-Reexamining-the-Guidelines.aspx.
The size, presentation, and lie of the fetus can be assessed with abdominal palpation. A systematic method of examination of the gravid abdomen was first described by Leopold and Sporlin in 1894.31 Although the abdominal examination has several limitations (especially in the setting of a small fetus, maternal obesity, multiple pregnancy, uterine fibroids, or polyhydramnios), it is safe, is well tolerated, and may add valuable information to assist in antepartum management. Palpation is divided into four separate Leopold’s maneuvers (Figure 6-1). Each maneuver is designed to identify specific fetal landmarks or to reveal a specific relationship between the fetus and mother. The first maneuver, for example, involves measurement of the fundal height. The uterus can be palpated above the pelvic brim at approximately 12 weeks’ gestation. Thereafter, fundal height should increase by approximately 1 cm per week, reaching the level of the umbilicus at 20 to 22 weeks’ gestation (Figure 6-2). Between 20 and 32 weeks’ gestation, the fundal height (in centimeters) is approximately equal to the gestational age (in weeks) in healthy women of average weight with an appropriately growing fetus. However, there is a wide range of normal fundal height measurements. In one study, a 6-cm difference was noted between the 10th and 90th percentiles at each week of gestation after 20 weeks.32 Moreover, maximal fundal height occurs at approximately 36 weeks’ gestation, after which time the fetus drops into the pelvis in preparation for labor. For all of these reasons, reliance on fundal height measurements alone fails to identify more than 50% of fetuses with fetal growth restriction (also known as intrauterine growth restriction).33 Serial fundal height measurements by an experienced obstetric care provider are more accurate than a single measurement and will lead to better diagnosis of fetal growth restriction, with reported sensitivities as high as 86%.34
If clinical findings are not consistent with the stated gestational age, ultrasonography is indicated to confirm gestational age and provide a more objective measure of fetal growth. Ultrasonography may also identify an alternative explanation for the discrepancy, such as multifetal pregnancy, polyhydramnios, fetal demise, and uterine fibroids. For many years, obstetric ultrasonography has used fetal biometry to define fetal size by weight estimations. This approach has a number of limitations. First, regression equations used to create weight estimation formulas are derived primarily from cross-sectional data for infants being delivered within an arbitrary period after the ultrasonographic examination. Second, these equations assume that body proportions (fat, muscle, bone) are the same for all fetuses.34–45 Finally, growth curves for “normal” infants between 24 and 37 weeks’ gestation rely on data collected from pregnancies delivered preterm, which are abnormal and probably complicated by some element of uteroplacental insufficiency, regardless of whether the delivery was spontaneous or iatrogenic. Despite these limitations, if the gestational age is well validated, the prevailing data suggest that prenatal ultrasonography can be used to verify an alteration in fetal growth in 80% of cases and to exclude abnormal growth in 90% of cases.46
Ultrasonographic estimates of fetal weight are commonly derived from mathematical formulas that use a combination of fetal measurements, especially the BPD, AC, and femur length.47 The AC is the single most important measurement and is weighted more heavily in these formulas. Unfortunately, the AC is also the most difficult measurement to acquire, and small differences in the measured value result in large changes in the estimated fetal weight (EFW). The accuracy of the EFW depends on a number of variables, including gestational age (in absolute terms, EFW is more accurate in preterm or growth-restricted fetuses than in term or macrosomic fetuses), operator experience, maternal body habitus, and amniotic fluid volume (measurements are more difficult to acquire if the amniotic fluid volume is low). Objective ultrasonographic EFW estimations have an error of 15% to 20%, even in experienced hands.48 Indeed, an ultrasonographic EFW at term is no more accurate than a clinical estimate of fetal weight made by an experienced obstetric care provider or the mother’s estimate of fetal weight if she has delivered before.49 Ultrasonographic estimates of fetal weight must therefore be evaluated within the context of the clinical situation and balanced against the clinical estimate. Serial ultrasonographic evaluations of fetal weight are more useful than a single measurement in diagnosing abnormal fetal growth. The ideal interval for fetal growth evaluations is every 3 to 4 weeks, because more frequent determinations may be misleading. Similarly, the use of population-specific growth curves, if available, improves the ability of the obstetric care provider to identify abnormal fetal growth. For example, growth curves derived from a population that lives at high altitude, where the fetus is exposed to lower oxygen tension, will be different from those derived from a population at sea level. Abnormal fetal growth can be classified as insufficient (fetal growth restriction) or excessive (fetal macrosomia).
Fetal Growth Restriction
The definition of fetal growth restriction has been a subject of long-standing debate. Distinguishing the healthy, constitutionally small-for-gestational-age (SGA) fetus, defined as having an EFW below the 10th percentile for a given week of gestation, from the nutritionally deprived, truly growth-restricted fetus has been particularly difficult. Fetuses with an EFW less than the 10th percentile are not necessarily pathologically growth restricted. Conversely, an EFW above the 10th percentile does not necessarily mean that an individual fetus has achieved its growth potential, and such a fetus may still be at risk for perinatal mortality and morbidity. Therefore, fetal growth restriction is best defined as either (1) an EFW less than the 5th percentile for gestational age in a well-dated pregnancy or (2) an EFW less than the 10th percentile for gestational age in a well-dated pregnancy with evidence of fetal compromise, such as oligohydramnios or abnormal umbilical artery Doppler velocimetry.
Fetal growth restriction has traditionally been classified as either asymmetric or symmetric fetal growth restriction. Asymmetric fetal growth restriction, characterized by normal head growth but suboptimal body growth, is seen most commonly in the third trimester. It is believed to result from a late pathologic event (e.g., chronic placental abruption leading to uteroplacental insufficiency) in an otherwise uncomplicated pregnancy and normal fetus. In cases of symmetric fetal growth restriction, both the fetal head size and body weight are reduced, indicating a global insult that likely occurred early in gestation. Symmetric fetal growth restriction may reflect an inherent fetal abnormality (e.g., fetal chromosomal anomaly, inherited metabolic disorder, early congenital infection) or long-standing severe placental insufficiency due to an underlying maternal disease (e.g., hypertension, pregestational diabetes mellitus, or collagen vascular disorder). In practice, the distinction between asymmetric and symmetric fetal growth restriction is not particularly useful.
Early and accurate diagnosis of fetal growth restriction coupled with appropriate intervention leads to an improvement in perinatal outcome. If fetal growth restriction is suspected clinically and on the basis of ultrasonography, a thorough evaluation of the mother and fetus is indicated. Referral to a maternal-fetal medicine specialist should be considered. Every effort should be made to identify the cause of the fetal growth restriction and to modify or eliminate contributing factors. Up to 20% of cases of severe fetal growth restriction are associated with fetal chromosomal abnormalities or congenital malformations, 25% to 30% are related to maternal conditions characterized by vascular disease, and a smaller proportion are the result of abnormal placentation. Other causes of fetal growth restriction include exposure to teratogens, alcohol, and substance abuse. In a substantial number of cases (>50% in some studies), the etiology of the fetal growth restriction remains unclear even after a thorough investigation.50
Fetal Macrosomia
Fetal macrosomia is defined as an EFW (not birth weight) of 4500 g or greater measured either clinically or by ultrasonography and is independent of gestational age, diabetic status, and actual birth weight.51 Fetal macrosomia should be differentiated from the large-for-gestational age (LGA) fetus, in whom the EFW is greater than the 90th percentile for gestational age. By definition, 10% of all fetuses are LGA at any given gestational age. Fetal macrosomia is associated with an increased risk for cesarean delivery, instrumental vaginal delivery, and birth injury to both the mother (including vaginal, perineal, and rectal trauma) and the infant (orthopedic and neurologic injury).52–56 Shoulder dystocia with resultant brachial plexus injury (Erb’s palsy) is a serious consequence of fetal macrosomia; it is more likely in the setting of diabetes because of the larger diameters of the fetal upper thorax and neck.
Fetal macrosomia can be determined clinically (e.g., Leopold’s maneuvers) or with ultrasonography, and these two techniques appear to be equally accurate.57 Estimated fetal weight measurements are less accurate in macrosomic fetuses than in normally grown fetuses, and factors such as low amniotic fluid volume, advancing gestational age, maternal obesity, and fetal position can compound these inaccuracies. Indeed, clinical examination has been shown to underestimate the birth weight by more than 0.5 kg in almost 80% of macrosomic fetuses.58 For all these reasons, prediction of fetal macrosomia is not particularly accurate, with a false-positive rate of 35% and a false-negative rate of 10%.57,58 A number of alternative ultrasonographic measurements have therefore been proposed in an attempt to better identify the macrosomic fetus, including fetal AC alone,59 umbilical cord circumference,60 cheek-to-cheek diameter,61 and subcutaneous fat in the mid humerus, thigh, abdominal wall, and shoulder.62 However, these measurements remain investigational.
Despite the inaccuracy in the prediction of fetal macrosomia, an EFW should be documented by either clinical estimation or ultrasonography in all high-risk women at approximately 38 weeks’ gestation. Suspected fetal macrosomia is not an indication for induction of labor, because induction does not improve maternal or fetal outcomes and may increase the risk for cesarean delivery.51 The American College of Obstetricians and Gynecologists (ACOG) recommends performance of an elective cesarean delivery when the suspected birth weight exceeds 4500 g in a diabetic woman or 5000 g in a nondiabetic woman.51,52,63
Assessment of Fetal Well-Being
All pregnant women should receive regular antenatal care throughout their pregnancy, and fetal well-being should be evaluated at every visit. Fetal heart activity should be assessed and the fetal heart rate (FHR) estimated. A low FHR (<100 bpm) is associated with an increased risk for pregnancy loss, although congenital complete heart block should be excluded. In the latter half of pregnancy, physical examination of the abdomen should be performed to document fetal lie and presentation.
Fetal movements (“quickening”) are typically reported at 18 to 20 weeks’ gestation by nulliparous women and at 16 to 18 weeks’ gestation by parous women; the presence of fetal movements is strongly correlated with fetal health. Although the mother appreciates only 10% to 20% of total fetal movements,64–67 such movements are almost always present when she does report them.67 Factors associated with a diminution in perceived fetal movements include increasing gestational age, smoking, decreased amniotic fluid volume, anterior placentation, and antenatal corticosteroid therapy. Decreased fetal movements may also be a harbinger of an adverse pregnancy event (e.g., stillbirth) that can be averted if detected early. For these reasons, a subjective decrease in perceived fetal movements in the third trimester should prompt an immediate investigation.
Published studies support the value of fetal movement charts (“kick counts”) in the detection and prevention of fetal complications (including stillbirth) in both high- and low-risk populations.68–73 The normal fetus exhibits an average of 20 to 50 (range of 0 to 130) gross body movements per hour, with fewer movements during the day and increased activity between 9:00 PM and 1:00 AM.74 Several different schemes have been proposed to determine the baseline fetal activity pattern for an individual fetus after 28 weeks’ gestation and to evaluate activity patterns that may represent fetal compromise. One commonly used scheme (“count-to-10”) instructs the mother to rest quietly on her left side once each day in the evening (between 7:00 PM and 11:00 PM) and to record the time interval required to feel 10 fetal movements. Most patients with a healthy fetus will feel 10 movements in approximately 20 minutes; 99.5% of women with a healthy fetus feel this amount of activity within 90 minutes.75 Under this scheme, failure to appreciate 10 fetal movements in 2 hours should prompt immediate fetal assessment. In one large clinical trial, institution of this fetal activity monitoring scheme resulted in a significant increase in hospital visits, labor induction, and cesarean deliveries, but also in a reduction in perinatal mortality from 44.5 to 10.3 per 1000 births.75 Taken together, these data suggest that daily or twice-daily fetal “kick counts” should be performed after 32 weeks’ gestation in high-risk pregnancies. Currently there is insufficient evidence to recommend this practice in low-risk pregnancies.
Prenatal Care in High-Risk Pregnancies
Approximately 20% of all pregnancies should be regarded as high risk (Box 6-2). Because of the attendant risks to both the mother and fetus, additional efforts should be made to confirm fetal well-being throughout such pregnancies. In addition to the testing outlined previously, high-risk pregnancies should be monitored closely and regularly by a multidisciplinary team, including subspecialists in maternal-fetal medicine and neonatology, if indicated.
Goals of Antepartum Fetal Testing
The goal of antepartum fetal surveillance is the early identification of a fetus at risk for preventable neurologic injury or death. Numerous causes of neonatal cerebral injury exist, including congenital abnormalities, chromosomal abnormalities, intracerebral hemorrhage, hypoxia, infection, drugs, trauma, hypotension, and metabolic derangements (e.g., hypoglycemia, thyroid dysfunction). Antenatal fetal testing cannot reliably predict or detect all of these causes; however, those specifically associated with uteroplacental vascular insufficiency should be identified when possible. Antenatal fetal testing makes the following assumptions: (1) pregnancies may be complicated by progressive fetal asphyxia that can lead to fetal death or permanent neurologic handicap; (2) current antenatal tests can adequately discriminate between asphyxiated and nonasphyxiated fetuses; and (3) detection of asphyxia at an early stage can lead to an intervention that is capable of reducing the likelihood of an adverse perinatal outcome.
Of interest, it is not clear whether any of these assumptions are true, and nonreassuring fetal test results may reflect existing but not ongoing neurologic injury. At most, 15% of cases of cerebral palsy are thought to result from antepartum or intrapartum hypoxic-ischemic injury.75–78 Despite these limitations, a number of antepartum tests have been developed in an attempt to identify fetuses at risk. These include the nonstress test (NST), biophysical profile (BPP), and contraction stress test (CST). Such tests can be used either individually or in combination. There is no consensus as to which of these modalities is preferred, and no single method has been shown to be superior.1
Antepartum Fetal Tests
All antepartum fetal tests should be interpreted in relation to the gestational age, the presence or absence of congenital anomalies, and underlying clinical risk factors.79 For example, a nonreassuring NST in a pregnancy complicated by severe fetal growth restriction and heavy vaginal bleeding at 32 weeks’ gestation has a much higher predictive value in identifying a fetus at risk for subsequent neurologic injury than an identical tracing in a well-grown fetus at 40 weeks, because of the higher prevalence of this condition in the former situation. It should be remembered that, in many cases, the efficacy of antenatal fetal testing in preventing long-term neurologic injury has not been validated by prospective randomized clinical trials. Indeed, because of ethical and medicolegal concerns, there are no studies of pregnancies at risk that include a nonmonitored control group, and it is highly unlikely that such trials will ever be performed.
Nonstress Test
The fetal nonstress test, also known as fetal cardiotocography, investigates changes in the FHR pattern with time and reflects the maturity of the fetal autonomic nervous system. For this reason, it is less useful in the extremely premature fetus (< 28 weeks) before the autonomic nervous system has matured sufficiently to influence the FHR. The NST is noninvasive, simple to perform, inexpensive, and readily available in all obstetric units. However, interpretation of the NST is largely subjective. Although a number of different criteria have been used to evaluate these tracings, most obstetric care providers have used the definitions for FHR interpretation established in 1997 by the National Institute of Child Health and Human Development (NICHD) Research Planning Workshop (Table 6-2).80 A 2008 NIH report summarized terminology and nomenclature used in contemporary clinical practice. This report described a three-tier system for FHR tracing interpretation: category I (normal), category II (indeterminate), and category III (abnormal).81
TABLE 6-2
Interpretation of Antepartum Nonstress Test Results
Data from the National Institute of Child Health and Human Development Research Planning Workshop. Electronic fetal heart rate monitoring: research guidelines for interpretation. Am J Obstet Gynecol 1997; 177:1385-90.
By definition, an NST is performed before the onset of labor and does not involve invasive (intrauterine) monitoring. The test is performed by recording the FHR for a period of 20 to 40 minutes; the recording is then evaluated for the presence of periodic changes. The FHR is determined externally with use of Doppler ultrasonography, in which sound waves emitted from the transducer are deflected by movements of the heart and heart valves. The shift in frequency of these deflected waves is detected by a sensor and converted into heart rate. The FHR is printed on a strip-chart recorder running at 3 cm/min. A single mark on the FHR tracing therefore represents the average rate in beats per minute (bpm) of 6 fetal heart beats. The presence or absence of uterine contractions is typically recorded at the same time with an external tonometer. This tonometer records myometrial tone and provides information about the timing and duration of contractions, but it does not measure intrauterine pressure or the intensity of the contractions. Results of the NST are interpreted as reactive or nonreactive. An FHR tracing is designated reactive if there are two or more accelerations of at least 15 bpm for 15 seconds in a 20-minute period (Figure 6-3).80–82 For preterm fetuses (<32 weeks’ gestation), an FHR tracing is designated as reactive if there are two or more accelerations of at least 10 bpm for 10 seconds.
FIGURE 6-3 A normal (reactive) fetal heart rate (FHR) tracing. The baseline FHR is normal (between 110 and 160 bpm), there is moderate variability (defined as 6 to 25 bpm from peak to trough), there are no decelerations, and there are two or more accelerations (defined as an increase in FHR of ≥ 15 bpm above baseline lasting at least 15 seconds) in a 20-minute period.
An NST is performed when formal documentation of the fetal condition is necessary. Because most healthy fetuses move within a 75-minute period, the testing period for an NST should not exceed 80 minutes.83 The NST is most useful in cases of suspected uteroplacental insufficiency. A reactive NST is regarded as evidence of fetal health,84,85 but the interpretation of a nonreactive NST remains controversial. Determination of a nonreactive NST must consider the gestational age, the underlying clinical circumstance, and the results of previous FHR tracings. Only 65% of fetuses have a reactive NST by 28 weeks’ gestation, whereas 95% do so by 32 weeks.79,86 However, once a reactive NST has been documented in a given pregnancy, the NST should remain reactive throughout the remainder of the pregnancy. A nonreactive NST at term is associated with poor perinatal outcome in only 20% of cases. The significance of such a result at term depends on the clinical endpoint under investigation. If the clinical endpoint of interest is a 5-minute Apgar score less than 7, a nonreactive NST at term has a sensitivity of 57%, a positive predictive value of 13%, and a negative predictive value of 98% (assuming a prevalence of 4%). If the clinical endpoint is permanent neurologic injury, a nonreactive NST at term has a 99.8% false-positive rate.87
Visual interpretation of the FHR tracing involves the following components: (1) baseline FHR, (2) baseline FHR variability, (3) presence of accelerations, (4) presence of periodic or episodic decelerations, and (5) changes of FHR pattern over time. The definitions of each of these variables are summarized in Table 6-2.80,81 The patterns are categorized as baseline, periodic (i.e., associated with uterine contractions), or episodic (i.e., not associated with uterine contractions). Periodic changes are described as abrupt or gradual (defined as onset-to-nadir time < 30 seconds or > 30 seconds, respectively). In contrast to earlier classifications, this classification makes no distinction between short-term and long-term variability, and certain characteristics (e.g., the definition of an acceleration) depend on gestational age (see Table 6-2).80,81
A normal FHR tracing is defined as having a normal baseline rate (110 to 160 bpm), normal baseline variability (i.e., moderate variability, defined as 6 to 25 bpm from peak to trough), presence of accelerations, and absence of decelerations. The FHR typically accelerates in response to fetal movement. Therefore, FHR accelerations usually indicate fetal health and adequate oxygenation.80–82 At-risk FHR patterns demonstrate recurrent late decelerations with absence of baseline variability, recurrent variable decelerations with absence of baseline variability, or substantial bradycardia with absence of baseline variability (Figure 6-4). Intermediate FHR patterns have characteristics between the two extremes of normal and at risk already described.80,81
FIGURE 6-4 An “at risk” fetal heart rate (FHR) tracing. The baseline FHR is normal (between 110 and 160 bpm), but the following abnormalities can be seen: minimal baseline FHR variability (defined as 0 to 5 bpm from peak to trough), no accelerations, and decelerations that are late in character (start after the peak of the contraction) and repetitive (occur with more than half of the contractions).
Persistent fetal tachycardia (defined as an FHR > 160 bpm) may be associated with fetal hypoxia, maternal fever, chorioamnionitis (intrauterine infection), administration of an anticholinergic or beta-adrenergic receptor agonist, fetal anemia, or tachyarrhythmia. Persistent fetal bradycardia (FHR < 110 bpm) may be a result of congenital heart block, administration of a beta-adrenergic receptor antagonist, hypoglycemia, or hypothermia (Table 6-3). However, it may also indicate fetal hypoxia.80,81 Both tachyarrhythmias and bradyarrhythmias require immediate evaluation.
Baseline FHR variability, perhaps the most important component of the NST, is determined on a beat-to-beat basis by the competing influences of the sympathetic and parasympathetic nervous systems on the fetal sinoatrial node. A variable FHR, characterized by fluctuations that are irregular in both amplitude and frequency,80,81 indicates that the autonomic nervous system is functioning and that the fetus has normal acid-base status. Variability is defined as absent, minimal, moderate, or marked (see Table 6-2) (Figure 6-5).80,81 The older terms short-term variability and long-term variability are no longer used.81 Normal (moderate) variability indicates the absence of cerebral hypoxia. With acute hypoxia, variability may be minimal or marked. Persistent or chronic hypoxia is typically associated with loss of variability. Reduced variability also may be the result of other factors, including maternal drug administration (see Table 6-3), fetal arrhythmia, and neurologic abnormality (e.g., anencephaly).1,80,81
Vibroacoustic Stimulation
Fetal vibroacoustic stimulation (VAS) refers to the response of the FHR to a vibroacoustic stimulus (82 to 95 dB) applied to the maternal abdomen for 1 to 2 seconds in the region of the fetal head. An FHR acceleration in response to VAS represents a positive result and is suggestive of fetal health. VAS is a useful adjunct to shorten the time needed to achieve a reactive NST and to decrease the proportion of nonreactive NSTs at term, thereby precluding the need for further testing. In one study of low-risk women at term, VAS reduced the proportion of nonreactive NSTs over a 30-minute period by 50% (from 14% to 9%) and shortened the time needed to achieve a reactive NST by an average of 4.5 minutes.88 VAS has no adverse effect on fetal hearing. The absence of an FHR acceleration in response to VAS at term is associated with an 18-fold higher risk for nonreassuring fetal testing in labor89 and a 6-fold higher risk for cesarean delivery.90
Biophysical Profile
An NST alone may not be sufficient to confirm fetal well-being. In such cases, a biophysical profile (BPP) may be performed. The BPP is an ultrasonographic scoring system performed over a 30- to 40-minute period designed to assess fetal well-being. Initially described for testing of the post-term fetus, the BPP has since been validated for use in both term and preterm fetuses, but not during active labor.91–97 The five variables described in the original BPP were (1) gross fetal body movements, (2) fetal tone (i.e., flexion and extension of limbs), (3) amniotic fluid volume, (4) fetal breathing movements, and (5) the NST.97 More recently, the BPP has been interpreted without the NST (Table 6-4).
TABLE 6-4
Characteristics of the Biophysical Profile
Biophysical Variable | Normal Score (Score = 2) | Abnormal Score (Score = 0) |
Fetal breathing movements (FBMs) | At least one episode of FBM lasting at least 30 sec | Absence of FBM altogether or no episode of FBM lasting ≥ 30 sec |
Gross body movements | At least three discrete body/limb movements in 30 min (episodes of active continuous movements should be regarded as a single movement) | Fewer than three episodes of body/limb movements over a 30-min period |
Fetal tone | At least one episode of active extension with return to flexion of fetal limbs or trunk; opening and closing of hand are considered normal tone | Slow extension with return to partial flexion, movement of limb in full extension, or absence of fetal movements |
Qualitative amniotic fluid (AF) volume | At least one pocket of AF that measures ≥ 1 cm in two perpendicular planes | No AF pockets or an AF pocket measuring < 1 cm in two perpendicular planes |
Reactive nonstress test | At least two episodes of FHR acceleration of ≥ 15 bpm lasting ≥ 15 sec associated with fetal movements over 30 min of observation | Fewer than two episodes of FHR accelerations or accelerations of < 15 bpm over 30 min of observation |
Data from Manning FA. Fetal biophysical assessment by ultrasound. In Creasy RK, Resnik R, editors. Maternal-Fetal Medicine: Principles and Practice. 2nd edition. Philadelphia, WB Saunders, 1989:359.
The individual variables of the BPP become apparent in the normal fetus in a predictable sequence: fetal tone appears at 7.5 to 8.5 weeks’ gestation, fetal movement at 9 weeks, fetal breathing at 20 to 22 weeks, and FHR reactivity at 24 to 28 weeks. In the setting of antepartum hypoxia, these characteristics typically disappear in the reverse order of their appearance (i.e., FHR reactivity is lost first, followed by fetal breathing, fetal movements, and finally fetal tone).93 The amniotic fluid volume, which is composed almost entirely of fetal urine in the second and third trimesters, is not influenced by acute fetal hypoxia or acute fetal central nervous system dysfunction. Rather, oligohydramnios (decreased amniotic fluid volume) in the latter half of pregnancy and in the absence of ruptured membranes is a reflection of chronic uteroplacental insufficiency and/or increased renal artery resistance leading to diminished urine output.98 It predisposes to umbilical cord compression, thus leading to intermittent fetal hypoxemia, meconium passage, or meconium aspiration. Adverse pregnancy outcome (including a nonreassuring FHR tracing, low Apgar scores, and/or admission to the neonatal intensive care unit) is more common when oligohydramnios is present.98–101 Weekly or twice-weekly screening of high-risk pregnancies for oligohydramnios is important because amniotic fluid can become drastically reduced within 24 to 48 hours.102
Although each of the five features of the BPP are scored equally (2 points if the variable is present or normal and 0 points if absent or abnormal, for a total of 10 points), they are not equally predictive of adverse pregnancy outcome. For example, amniotic fluid volume is the variable that correlates most strongly with adverse pregnancy events. The management recommended on the basis of the BPP score is summarized in Table 6-5.97 A score of 8 or 10 is regarded as reassuring; a score of 4 or 6 is suspicious and requires reevaluation; and a score of 0 or 2 suggests nonreassuring fetal status (previously referred to as “fetal distress”).91,92 Evidence of nonreassuring fetal status should prompt evaluation for immediate delivery.93,94
Contraction Stress Test
Also known as the oxytocin challenge test (OCT), the contraction stress test is an older test of uteroplacental function. It assesses the response of the FHR to uterine contractions induced by either intravenous oxytocin administration or nipple stimulation (which causes release of endogenous oxytocin from the maternal neurohypophysis). A minimum of three contractions of minimal-to-moderate strength in 10 minutes is required to interpret the test. A negative CST (no decelerations with contractions) is reassuring and suggestive of a healthy, well-oxygenated fetus. A positive CST (repetitive late or severe variable decelerations with contractions with at least 50% of the contractions) is suggestive of a fetus suffering from impaired maternal-to-fetal oxygen exchange during uterine contractions and is associated with adverse perinatal outcome in 35% to 40% of cases (Figure 6-6). The combination of a positive CST and absence of FHR variability is especially ominous. Consideration should be given to immediate and urgent delivery of a fetus with a positive CST, with or without FHR variability. It should be noted, however, that the false-positive rate of this test exceeds 50%.84 If the CST is uninterpretable or equivocal, the test should be repeated in 24 to 72 hours. Studies suggest that more than 80% of results of repeated tests are negative. The rate of antepartum intrauterine fetal demise within 1 week of a negative CST is 0.04%.84,97
FIGURE 6-6 A positive contraction stress test (CST) result. There are at least three contractions in a 10-minute period. The baseline fetal heart rate (FHR) is 130 bpm, there is minimal baseline FHR variability (defined as 0 to 5 bpm from peak to trough), and there are decelerations that are late in character (start after the peak of the contraction) and repetitive (occur with more than half of the contractions).
Because this test is time consuming, requires skilled nursing care, and necessitates an inpatient setting owing to the possibility of precipitating fetal compromise requiring emergency cesarean delivery, the CST is reserved for specific clinical indications. Moreover, there are a number of contraindications to its use, including placenta previa, placental abruption, prior classic (high-vertical) cesarean delivery, and risk for preterm labor. Despite these limitations, the CST allows for indirect evaluation of fetal oxygenation during periods of uterine contractions and diminished uteroplacental perfusion and may therefore provide a better assessment of fetal well-being and fetal reserve than either the NST or the BPP (Table 6-6).1,84,95,103
TABLE 6-6
False-Positive and False-Negative Rates for the Nonstress Test, Biophysical Profile, and Contraction Stress Test
Test | False-Positive Rate (%) | False-Negative Rate (per 1000 live births)* |
Nonstress test (NST) | 58 | 1.4 to 6.2 |
Biophysical profile (BPP): | 0.7 to 1.2 | |
• Score 6/10 | 45 | |
• Score 0/10 | 0 | |
Contraction stress test (CST) | 30 | 0.4 to 0.6 |
* Data are presented as perinatal mortality rate within 1 wk of a reactive NST, a BPP score of 8 or 10, or a negative CST after adjustments for congenital anomalies and known causes.
Data from references 1, 84, 95, and 103.
Umbilical Artery Doppler Velocimetry
Doppler velocimetry shows the direction and characteristics of blood flow and can be used to examine the maternal, uteroplacental, or fetal circulation. The umbilical artery is one of the few arteries that normally has diastolic flow and consequently is one of the vessels most frequently evaluated during pregnancy. Umbilical artery Doppler velocimetry measurements reflect resistance to blood flow from the fetus to the placenta. Normally, umbilical artery resistance falls progressively throughout pregnancy, reflecting the increase in number of tertiary stem vessels. Factors that affect placental vascular resistance include gestational age, placental location, pregnancy complications (e.g., placental abruption, preeclampsia), and underlying maternal disease (chronic hypertension).
Doppler velocimetry of umbilical artery blood flow provides an indirect measure of fetal status. Decreased diastolic flow with a resultant increase in the systolic-to-diastolic (S/D) ratio suggests an increase in placental vascular resistance and fetal compromise. Severely abnormal umbilical artery Doppler velocimetry (defined as absence of or reversed diastolic flow) is an especially ominous observation and is associated with poor perinatal outcome in the setting of fetal growth restriction (Figure 6-7).104–108 The role of ductus venosus and/or middle cerebral artery (MCA) Doppler velocimetry in the management of fetal growth restriction pregnancies is not well defined. Preparation for delivery—including administration of corticosteroids for fetal lung maturity and transfer to a tertiary delivery center—should be considered when Doppler findings are severely abnormal in the setting of fetal growth restriction, regardless of gestational age. However, in the presence of a normally grown fetus, it is unclear how to interpret such findings. For these reasons, umbilical artery Doppler velocimetry should not be performed routinely in women at low risk for fetal abnormalities. Appropriate indications include fetal growth restriction, cord malformations, unexplained oligohydramnios, suspected or established preeclampsia, and, possibly, fetal cardiac anomalies.
FIGURE 6-7 Umbilical artery Doppler velocimetry. A, Normal waveform in the umbilical artery as shown on Doppler velocimetry. Forward flow can be seen during both fetal systole and diastole. B, Absent end-diastolic flow. Forward flow can be seen during systole, but there is no flow during diastole. C, Reverse diastolic flow. Forward flow can be seen during systole, but there is reverse flow in the umbilical artery during diastole, which is suggestive of high resistance to blood flow in the placenta.
Umbilical artery Doppler velocimetry has not been shown to be useful in the evaluation of some high-risk pregnancies, including diabetic and post-term pregnancies, primarily owing to a high false-positive rate.2,109–112 Thus, in the absence of fetal growth restriction, obstetric management decisions are not usually made on the basis of Doppler velocimetry findings alone. New applications for Doppler technology include the use of MCA peak systolic velocity for the noninvasive evaluation of fetal anemia resulting from isoimmunization. When severe anemia develops in a fetus, blood is preferentially shunted to the vital organs, such as the brain, and the shunt can be demonstrated by an increase in MCA peak systolic flow velocity.113 This finding can help the perinatologist counsel affected patients about the need for cordocentesis and fetal blood transfusion. Doppler studies of other vessels (including the uterine artery, fetal aorta, ductus venosus, and fetal carotid arteries) have contributed to our knowledge of maternal-fetal physiology but as yet have resulted in few clinical applications.
Multiple Modalities to Assess Fetal Well-Being
All standard tests to assess antepartum fetal well-being (i.e., NST, BPP, CST) are evaluated according to their ability to predict the absence of fetal death during the 1-week period after the test. The false-negative rate (defined as a reassuring test result with a subsequent bad outcome) and false-positive rate (an abnormal result with a subsequent normal outcome) for each of these tests are listed in Table 6-6.1,84,95,103 The false-negative rates for all three tests are relatively low. Because the NST has a high false-positive rate, some authorities consider it a screening test to identify fetuses requiring further assessment with either a BPP or a CST. No method of fetal assessment is perfect, and clinical judgment plays a large role in any management decision.
Special Techniques for Antepartum Fetal Surveillance
Perinatal Ultrasonography
Ultrasonography uses high-frequency sound waves (3.5 to 5 MHz for transabdominal transducers and 5 to 7.5 MHz for transvaginal transducers) that are directed into the body by a transducer, reflected by maternal and fetal tissue, detected by a receiver, processed, and displayed on a screen. Increasing the wave frequency results in greater display resolution at the expense of diminished tissue penetration. Interpretation of images requires operator experience. Widespread clinical application of two-dimensional ultrasonography began in the 1960s after pioneering work by researchers in the United States and Great Britain.114 Although no deleterious biologic effects have been associated with obstetric ultrasonography, the rates of false-positive and false-negative diagnoses based on the images are a major limitation.
Perinatal ultrasonography can be classified broadly into three types of examinations: basic, targeted (comprehensive), and limited. The basic examination (level I) involves determination of fetal number, viability, position, gestational age, and gross malformations. Placental location, amniotic fluid volume, and the presence of abnormal maternal pelvic masses can be evaluated as well.20 Most pregnancies can be evaluated adequately with this type of examination alone. If the patient’s history, physical findings, or basic ultrasonographic results suggest the presence of a fetal malformation, an ultrasonographer who is skilled in fetal evaluation should perform a targeted or comprehensive examination (level II). During a targeted ultrasonographic examination, which is best performed at 18 to 20 weeks’ gestation, fetal structures are examined in detail to identify and characterize any fetal malformation. Ultrasonographic markers of fetal aneuploidy (see later discussion) can be evaluated as well. In some situations, a limited examination may be appropriate to answer a specific clinical question (e.g., fetal viability, amniotic fluid volume, fetal presentation, placental location, cervical length) or to provide ultrasonographic guidance for an invasive procedure (e.g., amniocentesis).
Current debate centers on identifying those patients who would benefit from an ultrasonographic evaluation and determining what type of evaluation would be optimal. Advocates of the universal application of ultrasonography cite the advantages of more accurate dating of pregnancy (see earlier discussion) and earlier and more accurate diagnosis of multiple gestation, structural malformations, and fetal aneuploidy (see later discussion). Opponents of routine ultrasonographic examination view it as an expensive screening test ($100 to $250 for a basic examination) that is not justified by published research, which suggests that routine ultrasonography does not change perinatal outcome significantly.19,26,27 Although routine ultrasonography for all low-risk pregnant women is controversial, few would disagree that the benefits far outweigh the costs for selected patients. The ACOG20 has recommended that the benefits and limitations of ultrasonography should be discussed with all pregnant women.
First-trimester ultrasonography
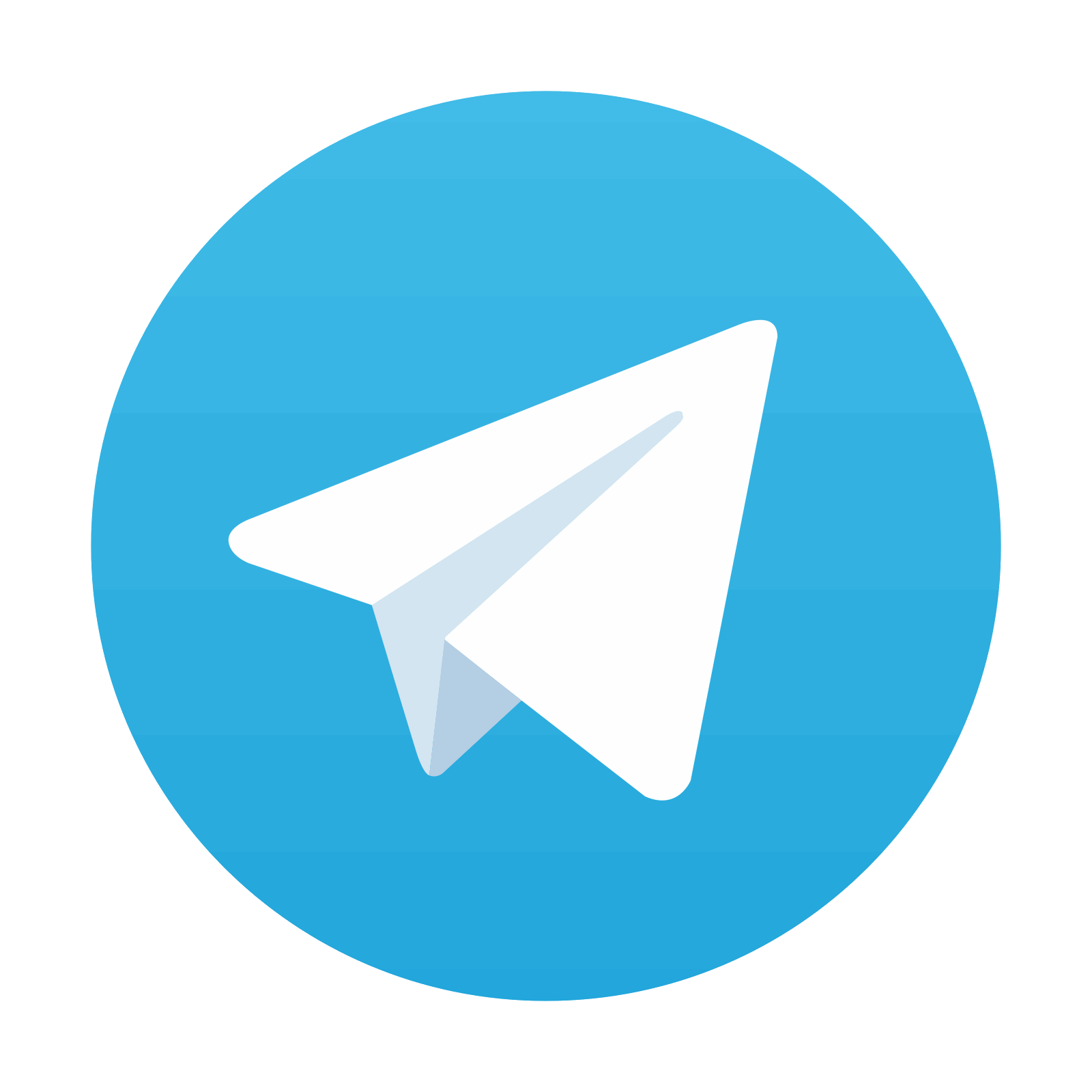
Stay updated, free articles. Join our Telegram channel

Full access? Get Clinical Tree
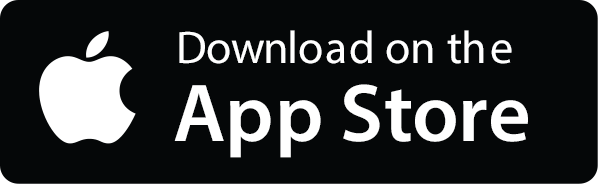
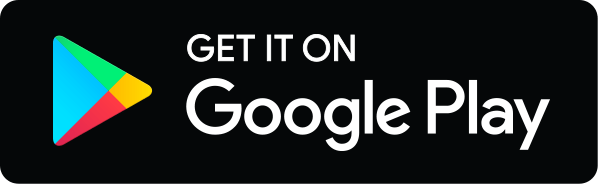